Spine, Spinal Cord, and Meninges
NORMAL SPINE AND VARIATIONS
The normal spine forms around the notochord, and separate vertebrae and intervertebral discs eventually develop. At birth, ossification of the vertebral bodies and neural arches is well underway, and one can readily identify three ossified structures for each vertebra. These include one for the vertebral body and one for each side of the neural arch. The synchondroses between these ossification centers are usually readily visualized (Fig. 8.1).
Normal curvatures of the spine in the neonate include a mild cervical lordosis, dorsolumbar kyphosis, and a lumbosacral lordosis (Fig. 8.1). As the infant grows older, the cervical and lumbosacral lordoses become more pronounced. The individual vertebral bodies are rectangular, especially in the thoracic region. In the lumbar area, normal
variation includes a slight oval or rounded appearance to the upper lumbar vertebral bodies (Fig. 8.1). Nutrient canals (central vascular grooves) are regularly seen on both frontal and lateral views (Fig. 8.1). Later, these become less prominent, and all the vertebral bodies become more square-cornered. Eventually, the normal ring epiphysis becomes visible (Fig. 8.2C), and then, in adolescence, it fuses with the vertebral body. The vertebra and spinal cord also are readily visualized with magnetic resonance imaging (MRI) (Fig. 8.2 A, and B).
variation includes a slight oval or rounded appearance to the upper lumbar vertebral bodies (Fig. 8.1). Nutrient canals (central vascular grooves) are regularly seen on both frontal and lateral views (Fig. 8.1). Later, these become less prominent, and all the vertebral bodies become more square-cornered. Eventually, the normal ring epiphysis becomes visible (Fig. 8.2C), and then, in adolescence, it fuses with the vertebral body. The vertebra and spinal cord also are readily visualized with magnetic resonance imaging (MRI) (Fig. 8.2 A, and B).
Ossification of the first and second cervical vertebrae is somewhat different from that of the other vertebrae. The first cervical vertebra has no body, but ossification centers for both lateral masses and the posterior aspect of the neural arch are usually present. In approximately 20% of newborn infants, ossification of the anterior arch of the first cervical vertebra is also seen at birth. By 6 months to 1 year of age, all infants show ossification of this latter structure. The body of C2 ossifies in a fashion similar to that of the other vertebral bodies, but the dens arises separately from two ossification centers. At birth, these centers usually have fused into a single structure, but in a few instances, a residual superior cleft may persist. This is often referred to as a bifid odontoid process. Occasionally, an extra, but normal, ossification center is seen just over this midline cleft, the os terminale (Fig. 8.3). When dens hypoplasia is present, this bone overgrows and becomes the os odontoideum.
Variation in the diameter of the spinal canal can be problematic because relative proportions usually are different from those in older children and adults. This is especially true of the cervical spine, where the normal interpedicular and sagittal distances can appear alarmingly wide (Fig. 8.4A). A similar problem arises in the lumbar region because the normal canal also widens in this area. Normal interpedicular distances in newborns and young infants are presented in Figure 8.5.
Another normal variation in the lumbar region of older infants and children consists of flattening of the inner aspects of the pedicles and posterior scalloping of the vertebral bodies of the upper lumbar vertebra. These findings are usually more pronounced in older infants and children, and one may falsely suspect an expanding intraspinal lesion in such cases (Fig. 8.6A). One also should be aware of the fact that posterior scalloping is normal in the lumbar vertebra (Fig. 8.6B). It should not be misinterpreted for pathologic scalloping due to an expanding intracanalicular lesion.
In the cervical spine, the various normal synchondroses can pose a problem in differentiation from fracture. Fortunately, fractures of the cervical spine are not particularly common in the neonate and very young infant, but these normal synchondroses should be appreciated (Fig. 8.4B). Most often, one sees these synchondroses on oblique views of the cervical spine, and the synchondroses between the dens and arch of C2 must be distinguished from the hangman’s fracture(1).
Pseudosubluxation of Cervical Spine
With pronounced forward flexion, there is a distinct tendency for the infant’s upper cervical spine to appear dislocated or subluxed(2). This is related to generalized laxity of the ligaments in the infant and horizontal positioning of the apophyseal joints. In the older child and adult, the joints become more vertical in position, but in the infant and young child their horizontal attitude predisposes to anterior displacement of the upper vertebral bodies, one on another. Most often, this involves C2 on C3, but pseudodislocation of the cervical spine can be seen down to the C4-5 level (Fig. 8.7).
Since the spine must be flexed to produce this pseudoabnormality, the soft tissues anterior to the spine also usually are distorted, and with the airway so buckled, the problem just becomes more confusing. Nonetheless, it is important to appreciate these changes because somewhat similar displacement of C2 on C3 can be produced by a hangman’s fracture of C2. Differentiation of the two conditions can be accomplished by use of the posterior cervical line(2). This line, drawn from the cortex of the spinous tip of C1 to the same location on C3, should touch or come within 1-1.5 mm of the anterior cortex of the spinous tip of C2 (Fig. 8.8). It remains normal with pseudosubluxation, but when the hangman’s fracture is present the line misses the anterior cortex of C2 by 2 mm or more.
Wedging of C3, and sometimes C4, is another normal variation that commonly is seen in infants and young children(3). It also is associated with normal hypermobility through this area, and it is the chronic, hammering impingement of C2 on C3 that causes C3 to under grow anteriorly. As a consequence, it becomes wedged (Fig. 8.9), and the configuration should not be misinterpreted for a compression fracture(3). Anterior compression fractures of C3 are uncommon in any age group, but if there still is doubt, computed tomography (CT) should be performed because it can clearly establish whether a fracture through the vertebral body exists.
C1-Dens Distance
The distance between the anterior arch of C1 and the odontoid process often appears unduly wide in infants. In adults, maximum normal distance is in the neighborhood of 2 to 2.5 mm, but in young infants, it frequently is 3 mm and can range as high as 4 to 5 mm and still be normal. This latter measurement occurs in less than 5% of cases. Normally, no matter what the original configuration this space, if normal, it does not increase by more than 2 mm during flexion.
Clefts of C1
Since CT was introduced, it has become more and more apparent that normal clefts exist in the anterior and posterior
(4, 5) portions of the ring of C1. These clefts can be identified as being normal and innocuous by their smoothly corticated edges (Figs. 8.3 and 8.10). They must be differentiated from fractures that occur through the ring of C1 in the form of the Jefferson fracture. Fractures generally demonstrate thin radiolucent lines with nonsclerotic bony edges.
(4, 5) portions of the ring of C1. These clefts can be identified as being normal and innocuous by their smoothly corticated edges (Figs. 8.3 and 8.10). They must be differentiated from fractures that occur through the ring of C1 in the form of the Jefferson fracture. Fractures generally demonstrate thin radiolucent lines with nonsclerotic bony edges.
![]() FIGURE 8.9. Normal wedging of C3. Note the marked wedging of C3 (arrow). No fractures were present in this patient. |
![]() FIGURE 8.10. Anterior defect of C1. Note the anterior defect of C1 (arrow); this is normal. For both anterior and posterior defects, see Figure 8.3C. |
Normal Spinal Cord
The normal spinal cord follows the same variations in diameter as does the spinal canal; it is wider in the cervical
region than in the thoracic area. Also recall that the spinal cord ends lower in the newborn infant, but as the infant grows older, the cord migrates towards the head, eventually ending at about L1(6,7,8). This level usually is reached in the first few months of life.
region than in the thoracic area. Also recall that the spinal cord ends lower in the newborn infant, but as the infant grows older, the cord migrates towards the head, eventually ending at about L1(6,7,8). This level usually is reached in the first few months of life.
The spinal cord is readily evaluated with ultrasound and MRI in infants, and ultrasound is especially useful in the neonate. Normal findings are illustrated in Figure 8.11. On the sagittal view, the normal spinal cord produces three echogenic lines, the outer two of which represent the anterior and posterior aspects of the cord. The cord itself is hypoechoic, and within it lies the third line, the echogenic central canal. The normal conus tapers smoothly and becomes continuous with the filum terminale. Below this level are the fibers of the cauda equina that produce linear echogenicities that blend together and almost totally fill the arachnoid space. Occasionally, one encounters dilation of the distal-most portion of the central canal. The configuration is usually somewhat oval, and the dilated structure is called the “ventriculus terminalis”(9,10,11,12). It is a normal finding not to be interpreted for some pathologic problem (Fig. 8.12).
A problem often arises in terms of numbering the vertebral bodies when ultrasound is being used. It has been suggested that the angle that occurs between the lumbar and sacral portions of the spine is consistent and can be used as a marker(13). In other cases, one may match the echogenic vertebra with those seen on plain films and thereby obtain accurate numbering of the vertebral bodies.
Many of the foregoing normal findings can cause problems with diagnosis and differentiation from pathologic conditions. A more comprehensive review(14) of these problems is available.
REFERENCES
1. Swischuk LE, Hayden CK Jr, Sarwar M. The dens-arch synchondrosis versus the hangman’s fracture. Pediatr Radiol 1979;8: 100-112.
2. Swischuk LE. Anterior displacement of C2 in children: physiologic or pathologic? A helpful differentiating line. Radiology 1977; 122:759-763.
3. Swischuk LE, Swischuk PN, John SD. Wedging of C3 in infants and children: usually a normal finding and not a fracture. Radiology 1993;188:523-526.
4. Chambers AA, Gaskill MF. Midline anterior atlas clefts: CT findings J Comput Assist Tomogr 1992;16:868-870.
5. Hosalkar HS, Gerardi JA, Shaw BA. Combined asymptomatic congenital anterior and posterior deficiency of the atlas. Pediatr Radiol 2001;31:810-813.
6. DePietro MA. The conus medullaris: normal US findings throughout childhood. Radiology 1993;188:149-153.
7. Hill CAR, Gibson PJ. Ultrasound determination of the normal location of the conus medullaris in neonates. AJNR 1995;16: 469-472.
8. Wolf S, Schneble F, Troger J. The conus medullaris: time of ascendence to normal level. Pediatr Radiol 1992;22:590-592.
9. Coleman LT, Zimmerman RA, Rorke LB. Ventriculitis terminalis of the conus medullaris: MR findings in children. AJNR 1995; 16:1421-1426.
10. Kriss VM, Kriss TC, Babcock DS. The ventriculus terminalis of the spinal cord in the neonate: a normal variant on sonography. AJR 1995;165:1491-1493.
11. Kriss VM, Coleman RC. Sonographic appearance of the ventriculus terminalis cyst in the neonatal spinal cord. J Ultrasound Med 2000;19:207-209.
12. Truong BC, Shaw DWW, Winters WD. Dilation of the ventriculus terminalis: sonographic findings. J Ultrasound Med 1998;17:713-715.
13. Beek FJA, van Leeuwen MS, Bax NMA, et al. A method for sonographic counting of the lower vertebral bodies in newborns and infants. AJNR 1994;15:445-449.
14. Swischuk LE. Normal cervical spine variations mimicking injuries in children. Emerg Radiol 1999;6:299-306.
CONGENITAL ABNORMALITIES OF THE SPINE, SPINAL CORD, AND MENINGES
To completely understand the various congenital anomalies of the spine, one must understand the developmental steps of vertebral body and arch formation. Only a few highlights are considered here, but for more detailed information refer to the classic study of The Human Spine in Health and Disease by Schmorl and Junghanns(1).
Early in the development of the vertebral bodies, two lateral chondrification centers are present, one on either side of the notochord. Two ossification centers also eventually appear, one anterior and one posterior. Eventually, the lateral chondrification centers fuse to form a single unit, but if union is incomplete, a sagittal cleft or butterfly vertebra results (Fig. 8.13). If only one of these lateral chondrification centers fails to develop, a hemivertebra results (Fig. 8.14). These hemivertebrae may be single or multiple, and abnormal spinal curvatures often result. There also is an increased incidence of ipsilateral rib anomalies and, in some cases, hypoplasia of the ipsilateral pulmonary artery and lung.
Abnormalities of the dorsal and ventral ossification centers are much less common. However, if one or the other fails to develop, a dorsal (more common) or ventral hemivertebra results (Fig. 8.15A). Underdevelopment of the ventral portion of a vertebral body frequently leads to severe gibbus
deformity. Occasionally, an entire vertebral body may fail to develop while the arch develops normally (Fig. 8.15B). In rare instances, both the body and laminal arch are absent (Fig. 8.15C), and in still other instances, only the laminal arches are absent(2). In such cases, instability of the spinal column may be profound, severe curvature abnormalities frequently result, and cord compression can occur(2).
deformity. Occasionally, an entire vertebral body may fail to develop while the arch develops normally (Fig. 8.15B). In rare instances, both the body and laminal arch are absent (Fig. 8.15C), and in still other instances, only the laminal arches are absent(2). In such cases, instability of the spinal column may be profound, severe curvature abnormalities frequently result, and cord compression can occur(2).
“Blocked” vertebra is the term applied to fusion of one or more adjacent vertebral bodies. The intervening intervertebral discs may be rudimentary or entirely absent. The isolated anomaly usually is of no particular consequence. Blocked vertebrae also are seen as part of the Klippel-Feil abnormality of the cervical spine. In isolated form, they most commonly occur in the cervical spine, and the discs above or below the region of abnormality may be hypertrophied and bulge into the spinal canal. They are, however, innocuous, and the finding is part of the entire anomaly (Fig. 8.16A).
Congenital absence of the pedicles(3) also occurs (Fig. 8.16B), especially in the lumbar region(4). They are of no particular consequence, but can be associated with aplastichypoplastic anomalies of the spine. They can cause back pain(3), but this is unusual.
REFERENCES
1. Schmorl G, Junghanns H. The human spine in health and disease. Translated from 4th German edition by Wilk SP, Goin LS. New York: Grune & Stratton, 1959:24-59.
2. Hughes LO, McCarthy RE, Glasier CM. Segmental spinal dysgenesis: a report of three cases. J Pediatr Orthop 1998;18:227-232.
3. Wiener MD, Martinez S, Forsberg DA. Congenital absence of a cervical spine pedicle: clinical and radiologic findings. AJR 1990; 155:1037-1041.
4. Polly DW Jr, Mason DE. Congenital absence of a lumbar pedicle presenting as back pain in children. J Pediatr Orthop 1991;11: 214-219.
Spondylolisthesis and Spondylolysis
Spondylolysis and subsequent spondylolisthesis are seldom seen on a congenital basis in infants. If, however, they should occur, severe curvature abnormalities may develop (Fig. 8.15). In older children, the problem is encountered more frequently, and primarily in the lumbar spine. There often is debate as to whether the lesion is congenital or acquired, but most likely it is acquired. However, it also may be, as I have noticed, that the pars interpeduncularis is thinner than usual in these patients. As a result, it would be more susceptible to subclinical or overt trauma. Reactive marrow changes have been demonstrated in the adjacent pedicles with MRI(1), and increased bone activity has been noted with nuclear scintigraphy using SPECT imaging(2, 3). All of this supports a traumatic, rather than a congenital origin. It is a lesion of active children(4), especially those who are athletic, and place excessive strain on the lumbar spine.
The degree of subsequent slippage of the upper vertebral body varies, and while usually the condition is painful(2, 5), it is surprising how often it is discovered as an incidental finding. It is a relatively common cause of low back pain in children, and associated spinal nerve root entrapment has been demonstrated(6). If only a defect exists, the term “spondylolysis” is used, but if there is associated anterior displacement of the upper vertebral body, the condition is called “spondylolisthesis.” The defect usually is visible on lateral view but is more exquisitely demonstrated on oblique view (Fig. 8.17). The findings also are vividly demonstrable with CT (Fig. 8.18).
The next most common place for spondylolysis to occur is at the C2 level(7, 8). It is questionable in many cases, however, whether the finding is completely congenital or actually the result of trauma and representative of a missed hangman’s fracture(9). One useful rule in differentiating the two is that, with congenital defects, the margins of the defect are smooth and sclerotic (Fig. 8.19), and the neural arch usually is deformed(10). It is not deformed when the defect is due to a hangman fracture. Congenital defects usually are stable on flexion-extension views, because the defect is replaced by fibrous or cartilaginous tissue. Familial cases have been reported(11).
When a posterior neural arch defect is due to a hangman fracture, the defect is narrow, sharp, and shows no sclerosis of the defect edges. The fracture is unstable and will require surgical intervention. In my experience, hangman fractures of C2 are more common than congenital defects, but they frequently go unnoticed (Fig. 8.20). Because they often are asymptomatic(12), they often are not initially detected, and also for this reason, others(13), as do I, believe that most of these defects are posttraumatic.
![]() FIGURE 8.18. Lumbar spondylolisthesis: CT findings. Axial view demonstrates bilateral pars defects with sclerotic edges (arrows). |
REFERENCES
1. Ulmer JL, Elster AD, Mathews VP, et al. Lumbar spondylolysis: reactive marrow changes seen in adjacent pedicles on MR images. AJR 1995;164:429-433.
2. Bellah RD, Summerville DA, Treves ST, et al. Low back pain in adolescent athletes: detection of stress injury to the pars interarticularis with SPECT. Radiology 1991;180:509-512.
3. Anderson K, Sarwark JF, Conway JJ, et al. Quantitative assessment with SPECT imaging at stress injuries of the pars interarticularis and response to bracing. J Pediatr Orthop 2000;20: 28-33.
4. Muschik M, Hahnel H, Robinson PN, et al. Competitive sports and the progression of spondylolisthesis. J Pediatr Orthop 1996; 16:364-369.
5. Lucey SD, Gross R. Painful spondylolisthesis in a two-year-old child. J Pediatr Orthop 1995;15:199-201.
6. Jinkins JR, Matthes JC, Sener RN, et al. Pictorial essay. Spondylolysis, spondylolisthesis, and associated nerve root entrapment in the lumbosacral spine: MNR evaluation. AJR 1992; 159:799-803.
7. Fardon DF, Fielding JW. Defects of the pedicle and spondylolisthesis of the second cervical vertebra. J Bone Joint Surg 1981;63: 526-528.
8. Smith JT, Skinner SR, Shonnard NH. Persistent synchondrosis of the second cervical vertebra simulating a hangman’s fracture in a child. J Bone Joint Surg Am 1993;75:1228-1230.
9. Konez O, Goyal M, Ciaverella CP. Bilateral absence of para interarticularis of C2: developmental or posttraumatic abnormality. Emerg Radiol 2001;8:48-50.
10. Williams JP III, Baker DH, Miller WA. CT appearance of congenital defect resembling the hangman’s fracture. Pediatr Radiol 1999;29:549-550.
11. Nordstrom REA, Lahdenranta TV, Kaitila II, et al. Familial spondylolisthesis of the axis vertebra. J Bone Joint Surg 1986; 68:704-706.
12. Kleinman PK, Shelton YA. Hangman’s fracture in an abused infant: imaging features. Pediatr Radiol 1997;27:776-777.
13. Howard AW, Letts RM. Cervical spondylolysis in children: it is posttraumatic? J Pediatr Orthop 2000;20:677-681.
Coronal Cleft Vertebra
Coronal cleft vertebra frequently is found as an incidental finding and usually involves the lumbar vertebrae. Coronal cleft vertebrae can be seen singly or multiply and can occasionally extend into the thoracic region (Fig. 8.21). The defect probably results from tardy union of the anterior and posterior ossification centers.
Coronal cleft vertebrae have been documented with conditions such as imperforate anus, VATER syndrome, spinal meningocele, and chondrodystrophia calcificans, but they are probably just as frequently encountered in the absence of these conditions and in normal infants. Consequently, one must be cautious in assigning any special significance to this anomaly. The clefts usually disappear as the infant grows older, and it is unusual to encounter them after 6 to 12 months of age. Coronal cleft vertebra is more common in males than in females and is common in a number of syndromes(1).
![]() FIGURE 8.19. Congenital defect posterior arch of C2. A. Note the congenital defect (arrow) with sclerotic margins. B. Note the defect (arrow) and markedly dysplastic, anomalous posterior arch of C2. |
REFERENCE
1. Westvik J, Lachman RS. Coronal and sagittal clefts in skeletal dysplasia. Pediatr Radiol 1998;28:764-770.
Specific Cervical Spine Anomalies
Occipitalization of C1, with or without associated basilar invagination and platybasia, is not usually a problem in the newborn infant. Problems related to these anomalies usually arise later in childhood. Extensive fusion-segmentation anomalies are seen with the Klippel-Feil syndrome and are seen in the neonate. This problem is very heterogenous(1), but most infants show marked shortening of the neck, with or without torticollis. Radiographs demonstrate extensive fusion-segmentation and curvature anomalies of the cervical, and at times, the upper thoracic spine (Fig. 8.22A). In some cases, associated upward rotation of the scapula also occurs and results in the so-called Sprengel’s deformity (Fig. 8.22B). Rib anomalies also are occasionally encountered, and the Klippel-Feil syndrome can be associated with congenital deafness, renal agenesis, and other anomalies.
Another relatively constant feature of this complex of anomalies is the omovertebral bone. This structure can be seen in the ligament extending from the spine to the abnormally positioned scapula in those patients with associated Sprengel’s deformity (Fig. 8.22C). Usually, the cervical spinal cord is normal even though the spinal anomalies may appear alarming. However cord abnormalities can occur(2). In mild cases, only minimal deformity of the cervical vertebral bodies may be seen and no clinical abnormality is detectable. However, neurologic deficits can develop in adulthood(3, 4).
Congenital absence of the odontoid is rare, and absence of the laminal arches and other anomalies were discussed previously. A bifid odontoid is the result of lack of fusion of the two ossification centers of the odontoid(5). Hypoplasia of the odontoid is associated with ligament underdevelopment and laxity and formation of the os odontoideum(6). The os odontoideum is an overgrown normal os terminale which overgrows when the dens is hypoplastic(6). It also can be acquired after trauma(7, 8). This occurs in infancy but is rare. In infancy in these cases blood supply to the dens is disrupted with severe upper spinal injuries and the dens fails to grow to normal proportions. It then becomes hypoplastic and as such induces the os terminale, on a compensatory basis, to overgrow and form an acquired os odontoideum. In these cases, as apposed to when the os odontoideum is congenital there are no other anomalies of the upper cervical spine. With a congenital os odontoideum, anomalies are numerous and often very bizarre (Fig. 8.23). The os odontoideum can fuse with the anterior arch of C1, and the entire complex of findings is more common in syndromes such as trisomy 21, spondyloepiphyseal dysplasia, and a variety of chondrodystrophies(6). When the posterior arch of C1 is severely hypoplastic or absent, hypermobility is profound (Fig. 8.24). In terms of deficiencies the posterior arch of C1 it should be noted that isolated defects are most common at this level (Fig. 8.25). Furthermore, even though they may appear bizarre and alarming, they are stable unless they are associated with a hypoplastic dens.
REFERENCES
1. Clarke RA, Catalan G, Diwan AD, et al. Heterogeneity in Klippel-Feil syndrome: a new classification. Pediatr Radiol 1998;28: 967-974.
2. Andronikou S, Fieggen AG. Klippel-Feil syndrome with cervical diastematomyelia in an 8-year-old boy. Pediatr Radiol 2001;31: 636.
3. Guiller JT, Miller A, Bowen JR, et al. The natural history of Klippel-Feil syndrome: clinical, roentgenographic, and magnetic resonance imaging findings at adulthood. J Pediatr Orthop 1995;15: 617-626.
4. Ulmer JL, Elster AD, Ginsberg LE, et al. Klippel-Feil syndrome: CT and MR of acquired and congenital abnormalities of cervical spine and cord. J Comput Assist Tomogr 1993;17:215-224.
5. Garant M, Oudjhane K, Sinsky A, et al. Duplication odontoid process: plain radiographic and CT appearance of a rare congenital anomaly of the cervical spine. AJNR 1997;18:1719-1720.
6. Swischuk LE, John SD, Moorthy C. The os terminale os odontoideum complex. Emerg Radiol 1997;4:72-81.
7. Schuler TC, Kurz L, Thompson DE, et al. Natural history of os odontoideum. J Pediatr Orthop 1991;11:222-225.
8. Kuhns LR, Loder RT, Farley FA, et al. Nuchal cord changes in children with os odontoideum: evidence for associated trauma. J Pediatr Orthop 1998;18:815-819.
Sacral Agenesis, Hypoplasia, and Caudal Regression
There is a wide gamut of lower lumbar and sacral spine abnormalities ranging from absence to mild hypoplasia. Hypoplasia may involve the entire sacrum or be more pronounced on one side. In the more severe cases of sacral agenesis, the lower lumbar spine is also usually absent. Absence of the spine above T11 or T12 is usually incompatible with life, and in these more severe cases the lower extremities are often fused in the so-called mermaid deformity. Extensive genitourinary and gastrointestinal anomalies (ectopic anus) are commonly present in such cases. Both of the lower extremities may be absent or underdeveloped, and the entire complex has been referred to as the caudal regression syndrome. These abnormalities are more frequently seen in infants delivered of diabetic mothers.
Generalized sacral hypoplasia, especially if mild, may go unnoticed but in later life may lead to various degrees of bowel, bladder, or motor impairment. In sacral agenesis, on the other hand, neurologic deficit is more profound, and associated clubfoot deformity, wasting of the muscles of the lower extremities, and incontinence of the urinary and anal sphincters are seen. Neurologic deficit in these cases results from underdevelopment of the corresponding nerve roots and distal spinal cord. Innervation usually is intact to the L5-S1 level and, as a result, development of the thigh muscles
is relatively normal. This leads to a tapered or coneshaped appearance of the lower extremities.
is relatively normal. This leads to a tapered or coneshaped appearance of the lower extremities.
Roentgenographically, the sacral anomalies are readily appreciated (Fig. 8.26). The pelvic bones are more closely apposed than normal, and the pelvis often becomes somewhat contracted. Intraspinal lipomas can co-exist, and these infants frequently show cutaneous abnormalities such as tufts of hair, dimples, and subcutaneous lipomas.
Meningocele and Meningomyelocele
Meningocele and meningomyelocele are common abnormalities of the spinal cord and meninges. Meningocele denotes herniation of the meninges only; in meningomyelocele, the sac also contains the spinal cord and nerve roots. Both abnormalities are commonly associated with lacunar skull and the Arnold-Chiari malformation. Most commonly, meningocele and meningomyelocele are encountered in the lumbosacral region, and next most commonly they occur in the lower thoracic spine. Least frequent are cervical defects, and in rare instances, these lesions may be contiguous with a midline posterior occipital encephalocele. Multiple defects also can occur.
There is no difficulty in clinically recognizing this abnormality, because the widened spinal canal and numerous vertebral defects and deformities are readily apparent roentgenographically (Fig. 8.27). In some cases, the neural arches are so defective that only vague vestiges remain. Some of these rudimentary bony structures are so misplaced that one might misinterpret them as representing the bony spicule of diastematomyelia. In other, less common instances there may be a soft tissue bulge only and little in the way of vertebral body or neural arch abnormality. In more advanced cases, the anterior aspect of the vertebral bodies becomes rounded (Fig. 8.27B). These patients can demonstrate a severe lumbar kyphosis that now is corrected surgically in early infancy(1). This is performed to avoid later severe scoliotic and kyphotic curvature problems.
It is not possible to determine the precise level of communication from plain roentgenograms because it does not necessarily correspond to the area of most severe deformity. The communication may be small but, in any case, if one is interested in obtaining further information regarding these lesions and, more specifically, in outlining the spinal cord and nerve roots, it is best to employ MRI(2,3,4). This modality most clearly delineates the abnormal underlying anatomy. In the neonate, however, ultrasonography can be useful in delineating the sac of the meningocele, the echogenic neural elements inside, and the presence of associated lesions such as lipomas.
Not uncommonly, the spinal cord in patients with meningomyelocele or meningocele shows widening secondary to hydromelia(3). Lipomas frequently co-exist(1) and on CT studies are of low signal intensity (i.e., radiolucent). On MRI, they produce high T1 signal intensity, and they are highly echogenic on ultrasonographic studies. Rib anomalies and deformities also commonly co-exist, and with more severe dysraphism, intraabdominal structures such as the aorta and kidneys can be displaced posteriorly and centrally. For this reason, it is often difficult to examine these kidneys with ultrasound. Meningoceles and meningomyeloceles also can be detected in utero with ultrasound.
Although most meningoceles are midline and dorsal in location, one can occasionally encounter a lateral or anterior meningocele. The latter abnormalities most commonly occur in the thoracic or sacral regions. Such meningoceles
are frequently associated with neurofibromatosis, and the roentgenographic findings include the presence of a mass (meningocele), a variety of vertebral abnormalities, and in the thorax, thinning of the adjacent ribs.
are frequently associated with neurofibromatosis, and the roentgenographic findings include the presence of a mass (meningocele), a variety of vertebral abnormalities, and in the thorax, thinning of the adjacent ribs.
Anterior sacral meningoceles enter into the differential diagnosis of presacral pelvic masses in newborn and young infants. Primarily, one must differentiate them from presacral teratoma, hydrometrocolpos, ovarian cysts, and pelvic neuroblastoma. Intrasacral or so-called occult sacral meningoceles are not usually detected in the newborn and young infant.
In rare instances, one may come upon a complete midline defect of the spinal column, including the neural arches posteriorly and the vertebral bodies anteriorly (Fig. 8.28). This abnormality has been called “double spine,” total rachischisis, split notochord, or combined anterior and posterior meningomyelocele(6, 7). In some instances, dorsal herniation of the gastrointestinal tract through this defect can occur(6). In other instances, associated neurenteric cysts or neurenteric fistulas can be encountered, and yet in other cases the defects may be associated with hamartomas or actual deformed, accessory extremities(8, 9) (Fig. 8.28). Although originally the split notochord syndrome was considered an entity unto itself, more and more it is included in the spectrum of meningocele, diastematomyelia, diplomyelia, and neurenteric cyst(7).
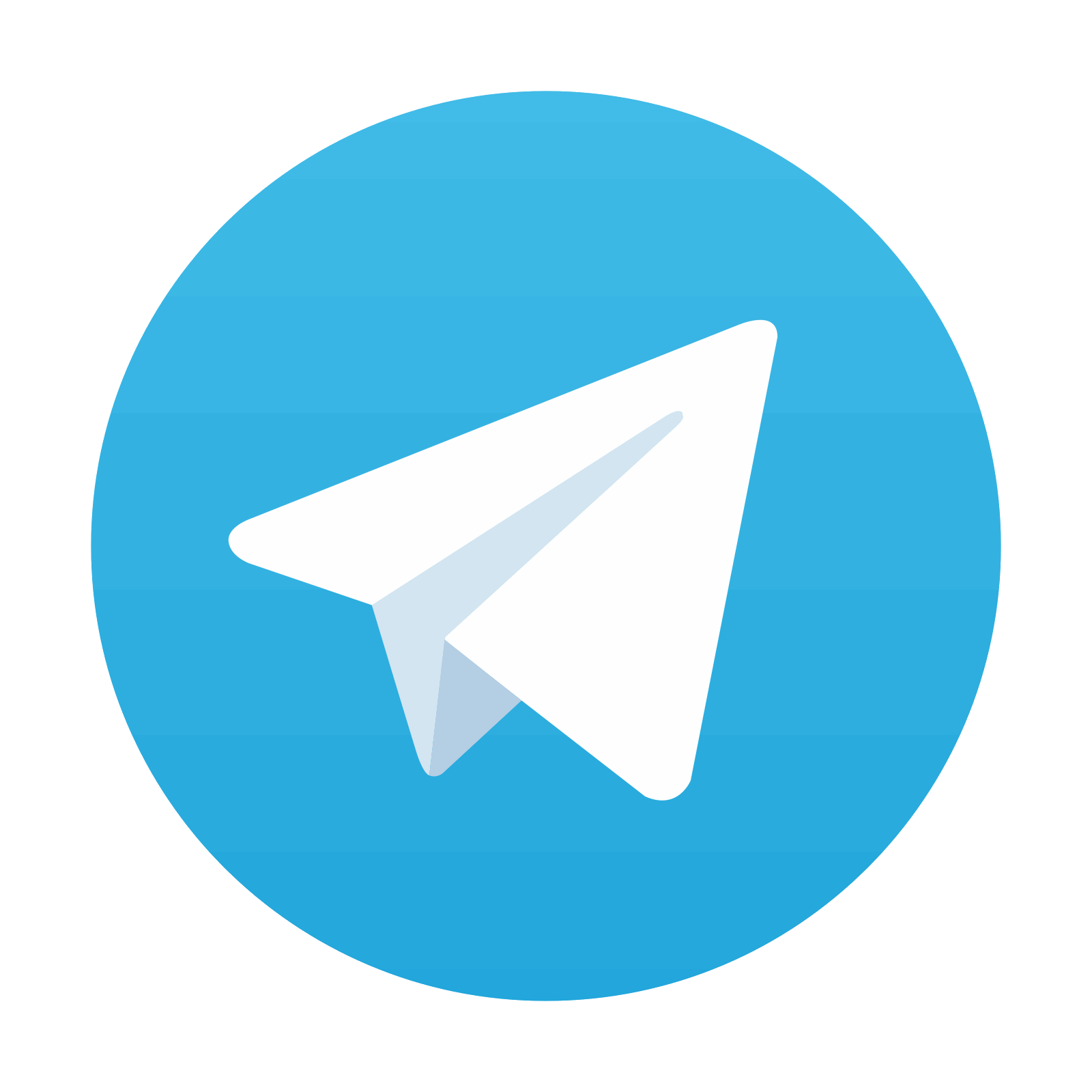
Stay updated, free articles. Join our Telegram channel

Full access? Get Clinical Tree
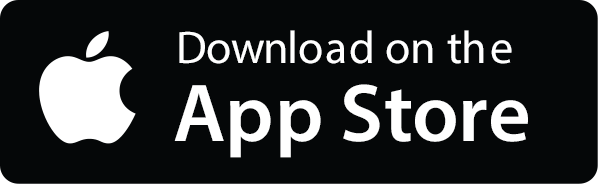
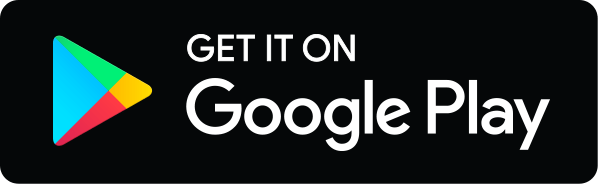