Stereotactic Body Radiation Therapy
22.1. INTRODUCTION
Stereotactic body radiation therapy (SBRT) refers to a stereotactic radiotherapy procedure for treating extracranial tumors with ultrahigh doses per fraction (6 to 30 Gy), in a hypofractionated regimen of five or fewer fractions. SBRT has been mostly applied to the tumors in the spine, lung, liver, pancreas, kidney, and prostate. Because the treatment involves large doses per fraction delivered in a few fractions, conformation of high dose to the target volume together with a rapid fall-off of dose outside the target volume is of critical importance in minimizing damage to normal tissue. Accordingly, the accuracy of dose delivery and conformity of dose distribution are of paramount importance. The stringent accuracy requirements of tumor localization and dose delivery in SBRT can only be attained through meticulous planning, patient immobilization, respiratory motion management, and state-of-the-art image guidance techniques for target localization and geometric verification.
Major characteristics that distinguish SBRT from conventional 3-D/IMRT radiotherapy include unconventional dose fractionation (e.g., large dose per fraction delivered in fewer fractions), applicability only to well-circumscribed tumors (with a maximum cross-sectional diameter of about 5 cm or less), allowance of small or no margins for beam penumbra, stringent need for patient immobilization and respiratory motion management, and higher frequency of patient monitoring and geometric verification through image guidance. To this list, one may also add the requirement of providing special SBRT training for the participating staff.
Radiobiological rationale underlying SBRT is essentially similar to that underlying cranial stereotactic radiosurgery and radiotherapy, i.e., high dose fractions delivered over a short period of time have more potent biologic effect than expected for the same total dose delivered through conventional fractionation. However, such dose intensification can also substantially increase the risk of normal tissue toxicities. Because the radiation doses delivered in SBRT are essentially ablative, the volume of normal tissue receiving high-dose exposure must be minimized. This is only possible by accurate delivery of highly conformal dose to the target while sparing surrounding normal tissues through steep dose gradients. For an overview of radiobiological and clinical rationale of SBRT, the reader is referred to the cited literature (1, 2, 3, 4).
22.2. SIMULATION
The goal of SBRT simulation is to provide clear visualization of patient anatomy with the patient in the treatment position and to acquire three-dimensional anatomic data that would be used for treatment planning, treatment setup, and dosimetric calculations. Modern methods of simulation incorporate CT, MRI, and PET (discussed in Chapter 12). The choice of a particular imaging modality depends on the tissue characteristics being imaged. Whereas CT simulation is the primary imaging modality, MRI and PET are often used as complementary modalities. PET/CT simulator is an example of two complementary imaging systems integrated together with a common couch, thus allowing acquisition of PET and CT images in a single simulation session without change in patient position relative to the setup isocenter. As a result, uncertainties in image registration and image fusion are reduced.
SBRT simulation consists essentially of three components: sufficient patient immobilization, management/control of tumor motion, and acquisition of 3-D anatomic image data for treatment planning and image-guided treatment delivery. These components are discussed below.
A. PATIENT IMMOBILIZATION
The safe delivery of ultrahigh doses delivered with SBRT requires greater spatial accuracy than that of conventional fractionated radiation therapy. When the technique was first introduced in the 1990s, image-guided treatment delivery was not yet commercially available. Conventional immobilization devices were inadequate to ensure accurate and reproducible patient fixation. As a result, stereotactic “body frames” were developed to better immobilize patients for SBRT.
Several variations of stereotactic body frames are offered by vendors (e.g., Elekta, Leibinger, Medical Intelligence Body Fix). Ideally, body frames are light weight (e.g., <10 kg) and constructed of low density material, to reduce photon absorption as much as possible. These systems typically use vacuum cushions to conform and immobilize patient body. Localization apparatus is attached to the body frame or the couch top to provide reference coordinates of external fiducials. Some systems incorporate abdominal compression devices such as manual compression paddle or pneumatic compression belt to induce shallow breathing and thus minimize respiratory motion.
Lax and Blomgren (5) were the first to describe the use of the Elekta Body Frame for SBRT. The Elekta Body Frame contained an external reference coordinate system which consisted of longitudinal scales mounted to the outside of the body frame, and a stereotactic arc for lateral and AP coordinates. An additional arc was placed on the frame which contained a central screw for compressing the abdomen for diaphragmatic control. An image of the original Elekta Body Frame is shown in Figure 22.1.
Well-constructed body frames can improve patient reproducibility (e.g., as measured by the deviations to patients bony anatomy) to within a couple of millimeters (5,6). However, variations in the position of the target can be larger due to interfractional internal target motion. Lax (5) and Wulf (6) have reported deviations of 3 to 4 mm in the transverse plane, and 4 to 5 mm in the longitudinal plane for a variety of treatment sites within the Elekta Body Frame. Improvements in target alignment can only be made with image-guided radiation therapy.
B. MANAGEMENT OF TUMOR MOTION
Intrafractional variations in tumor position must be accounted for within a SBRT program. A number of different approaches have been used to manage tumor motion, which may be divided into passive and active motion management strategies (7).
Passive motion management involves accounting for tumor motion in the delineation of the patient target volumes. Typically, this is performed using 4-D computed tomography, or 4DCT. 4DCT scanning produces a series of CT datasets which correspond to different phases of the respiratory cycle. The phase of a particular point on the respiratory cycle is defined as the percentage of the cycle which has elapsed relative to the origin. The respiratory cycle ranges from full inspiration at the beginning of the cycle (i.e., phase = 0%) to full expiration (phase = 50%) and back to full inspiration (phase = 100%). There are several systems available which act as surrogates for the respiratory phase, including the position of the abdominal surface, respiratory air flow, and the position of internal anatomy (e.g., the diaphragm). For example, the Varian
Real-time Position Management (RPM) system shown in Figure 22.2A uses an infrared camera to track the motion of a reflective marker placed on a patient’s abdomen. The vertical (anteriorposterior) motion of the marker is recorded by the camera and plotted versus time on the display. The periodic trace is visible on the RPM display example shown in Figure 22.2B. Each peak in the trace represents the maximum vertical position of the marker which corresponds to full inspiration (i.e., phase = 0%). The position of the marker acts as a surrogate for the respiratory cycle, and is used for the generation of phased CT datasets, or in the gating of the treatment beam.
Real-time Position Management (RPM) system shown in Figure 22.2A uses an infrared camera to track the motion of a reflective marker placed on a patient’s abdomen. The vertical (anteriorposterior) motion of the marker is recorded by the camera and plotted versus time on the display. The periodic trace is visible on the RPM display example shown in Figure 22.2B. Each peak in the trace represents the maximum vertical position of the marker which corresponds to full inspiration (i.e., phase = 0%). The position of the marker acts as a surrogate for the respiratory cycle, and is used for the generation of phased CT datasets, or in the gating of the treatment beam.
In the GE/Varian implementation of 4DCT, the CT scanner operates at a fixed table position in cine mode, obtaining axial images at each desired phase of the respiratory cycle. The CT scan protocol is set up such that these images are taken at a frequency that exceeds the user-defined phase binning. For example, if the patient’s respiratory cycle was 4 seconds, an axial image should be obtained at least every 0.4 seconds if there are 10 phases desired for the output. At any axial table position, the cine duration is set equal to the breathing cycle plus the time required to obtain a single scan, which is usually around 6 to 7 seconds. After this time, the couch is incremented and axial images are obtained at the next table position in the same fashion.
The CT data obtained during the imaging are assigned a phase corresponding to the position of the reflective marker. The software then selects those images obtained at the phases closest to those desired by the user. The output is a series of CT axial images, each corresponding to a different phase of the respiratory cycle.
In addition to the individual datasets, additional CT datasets are obtained corresponding to the average, minimum, and maximum reconstructed CT numbers for each voxel. The latter
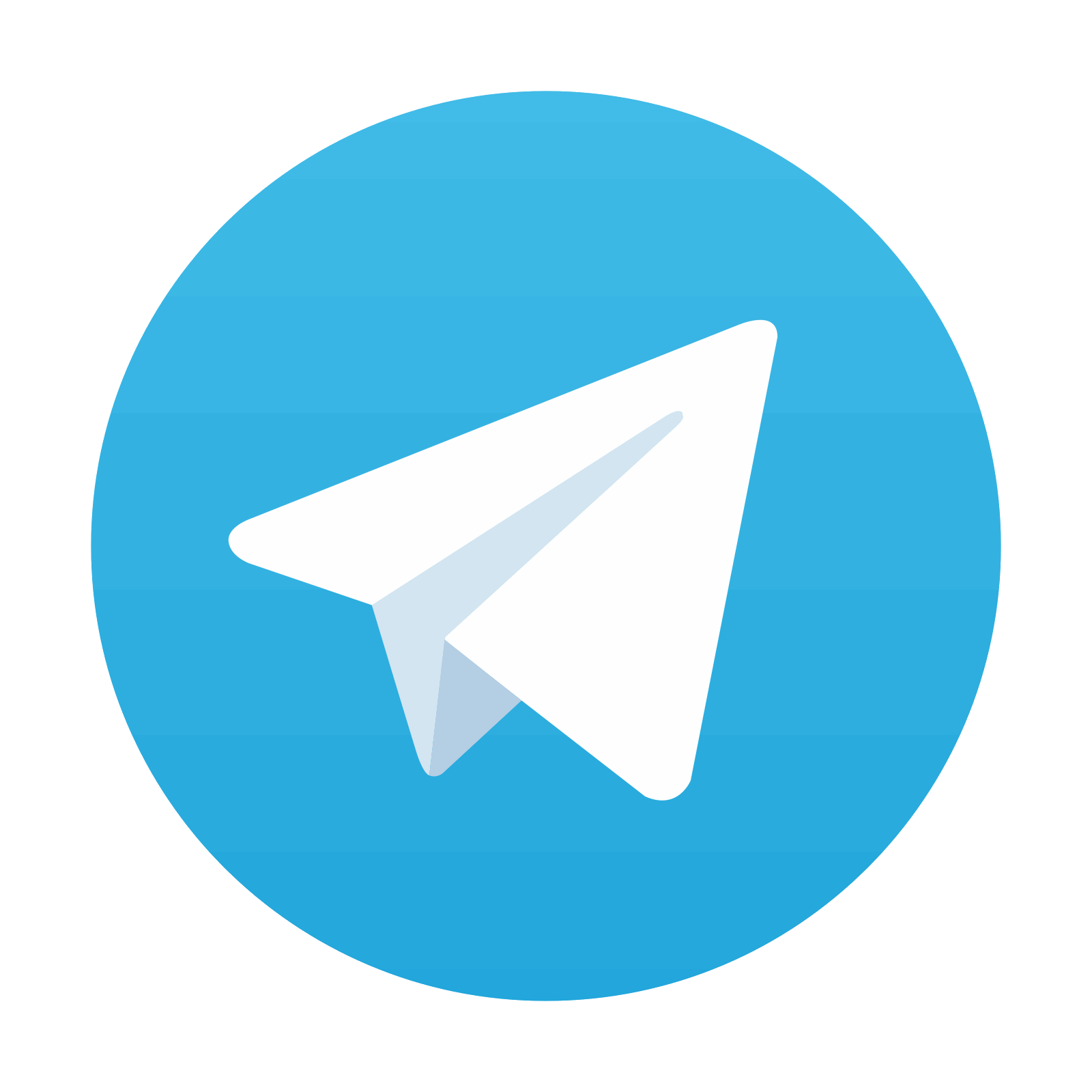
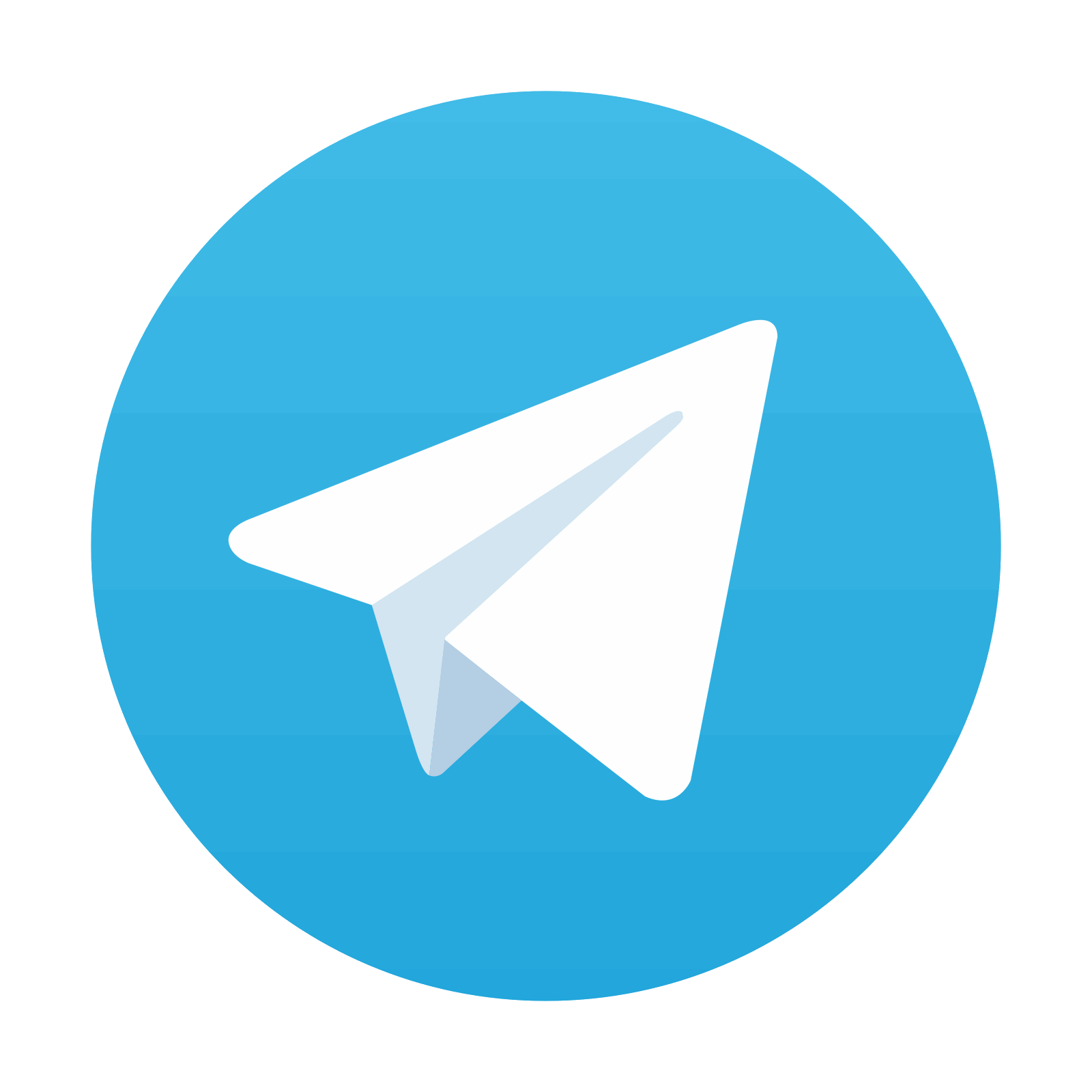
Stay updated, free articles. Join our Telegram channel

Full access? Get Clinical Tree
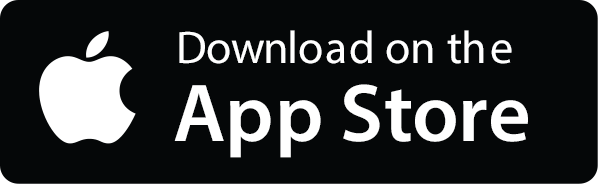
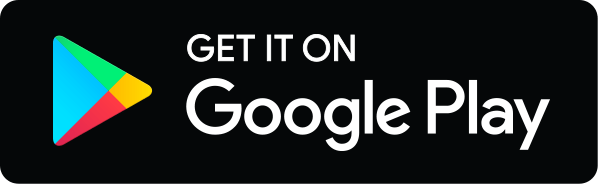
