1/3 liver (Gy)
2/3 liver (Gy)
Whole liver (Gy)
TD 5/5
50
35
30
TD 50/5
55
45
40
This tolerance is considerably less than the dose needed for durable control of biliary tumors. This model has been further adapted, particularly through work done at the University of Michigan [3–6], and a mean dose to the liver of less than 32 in 2 gray (Gy) fractions was established as a more accurate TD 5/5. Additionally, they have shown that there may be no upper limit on dose in volumes less than 25 % of the liver [4, 5]. The quantitative analyses of normal tissue effects in the clinic (QUANTEC) initiative published in 2010 established similar dose tolerances based in large part on the efforts from the Michigan group [7]. They also included a tolerance dose reduction in 4–6 Gy for patients with mild pre-existing hepatic dysfunction. This improved the understanding of the threshold for RILD and has allowed for doses capable of controlling tumors, particularly with the use of stereotactic body radiation therapy (SBRT) (Table [2]).
Table 2
Summary of control rates and reported survival in SBRT for intrahepatic cholangiocarcinoma
Study | Patients | Fractionation | System | Local control | OS | Notes |
---|---|---|---|---|---|---|
Blomgren et al. [1] Karolinska Hospital | 23 with intrahepatic cancers (1 IHCC) | Mean does to the PTV from 8–63 Gy | Traditional linear accelerator with 4–8 non-coplanar uncollimated octagaonal beam | 14 patients had a measurable response with five of those with stable disease | Not reported | First three patients treated with 7.7–20 Gy in one fraction and a fourth patient received two 20 Gy fractions separated by 72 days. All for of these patients progressed |
Tse et al. [2] Princess Margaret Hospital | 41 patients with intrahepatic cancers (ten with IHCC) | Median dose of 36 Gy (24–54 Gy) in 6 fractions | Individualized dose-escalation strategy integrating cine MRI and breath hold technique | One-year in-field control rate—65 % | Median survival—13.4 months for all patients | No RILD observed |
IHCC patient median survival—15.0 months | One patient had a tumor-duodenal fistula 15 months after SBRT and died of a GI bleed | |||||
One-year survival—58 % | One patient developed a small bowel obstruction requiring surgery at 15 months | |||||
Goodman et al. [3] Stanford University | 26 patients with intrahepatic tumors (5 with IHCC) | Dose-escalation single-fraction study | CyberKnife with implanted fiducials | One-year local failure rate—23 % | Median survival—28.6 months | No Grade 3 toxicities. Three patients developed duodenal ulcers (one early, two late) |
18 Gy (3 pts) | ||||||
22 Gy (9 pts) | ||||||
30 G (8 pts) | ||||||
Two-year OS—50.4 % | Two patients developed chest wall pain near the site of sbrt | |||||
Ibarra et al. [4] Multi-institutional | 32 patients with primary intrahepatic tumors (11 patients with IHCC) | 22–37.5 Gy in varying fractionations | Varied | FFLP of 55.5 % for IHCC | One-year OS—45 % | 39.5 % had Grades 1–2 toxicities (mainly nausea) |
Barney et al. [5] Mayo Clinic | Ten patients with unresectable or recurrent cholangiocarcinoma | 3–5 fractions to a dose of 45—60 Gy | IMRT or 3D conformal planning delivered with a standard linac | No in-field failures at median follow-up 14 months | Median OS—14 months | One patient had liver failure resulting in death |
2 Tumor Motion and the Use of Image-guided Adaptive Radiation
The advent of 3D conformal therapy and intensity-modulated radiation therapy has allowed for radiation treatment plans that shape dose around the target with a rapid fall off in surrounding tissues. This has allowed for treatment volumes to be vastly decreased compared to traditional 2D radiation (Fig. 1). As treatment volumes have decreased, the possibility of missing the target has vastly increased. Modern radiation treatments are typically designed first to target the visible tumor or gross tumor volume (GTV). This is then expanded to encompass microscopic disease in a clinical target volume (CTV). Finally, this CTV is expanded to account for day-to-day setup error, intra-fractional motion, and uncertainty in the planning target volume (PTV). The expansion to PTV and the ratio of treated volume to GTV and CTV increases with uncertainty. In an ideal system, PTV would equal CTV.
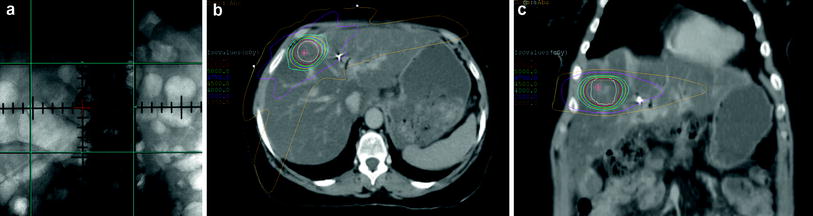
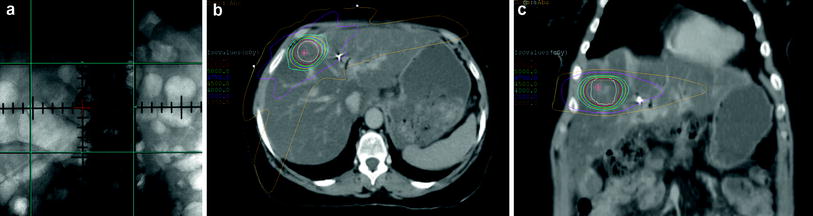
Fig. 1
Comparison of 2D radiation fields with SBRT fields. a Traditional 2D fields relied on bony targets without any adjustment for motion or target localizer such as fiducials. b Axial CT scan showing SBRT plan with very high radiation doses using multiple beam angles. c Coronal CT from same SBRT plan
3 Four-dimensional CT Scanning
The introduction of CT scanning through the entire range of the respiratory cycle, 4D-CT scans, revealed that respiratory motion and its effect on abdominal tumors are exceptionally variable [8–10]. This uncertainty in upper abdominal tumors is primarily driven by tumor motion from breathing but is also affected by the filling of hollow organs such as the stomach and duodenum. In a review of organ motion by Langen and Jones [11], liver motion (using a variety of techniques such as scintigraphy, MRI, and ultrasound) was found to average between 8 and 25 mm under normal breathing conditions and up to 37–55 mm on deep breathing.
This motion must be accounted for and traditionally this meant expanding margins and increasing radiation dose to normal tissues. The use of 4D-CT imaging has led to the use of internal target volumes (ITVs) in several disease sites. ITV is defined as a target which accounts for physiologic tumor motion. 4D-CT scans are created by acquiring several datasets at each CT slice position throughout the entire respiratory cycle. Reconstruction of this data creates a series of CT scans at predefined positions in the respiratory cycle. Tumor motion can be assessed by viewing these scans in the axial, coronal, and sagittal planes, as a movie or “cine-mode review” which displays the entire tumor range-of-motion (Fig. 2). The tumor can then be targeted throughout its entire range-of-motion.
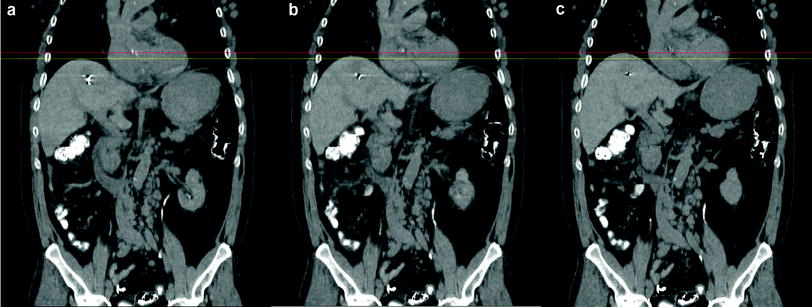
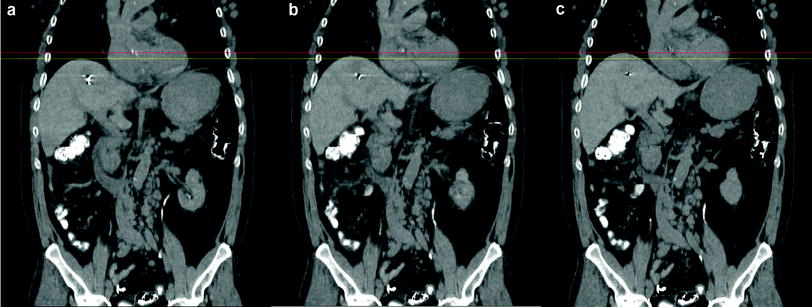
Fig. 2
Coronal CT demonstrating tumor motion throughout the respiratory cycle in the same patient. a End inspiration b mid-cycle and c end-expiratory. Red and yellow lines represent same plane at each point and allow for measurement of tumor motion. The artifact within the liver represents a gold seed fiducial
4 Reducing Organ Motion
While 4D-CT scanning describes tumor and organ motion, several techniques have been developed to try to reduce this motion, thus decreasing the volume treated. Abdominal compression techniques employ a device to apply a high amount of pressure to the abdomen. This increases intra-abdominal pressure, reducing diaphragmatic motion. Initial studies in abdominal SBRT from the Karolinska Institute in Sweden describe their abdominal compression methods which reduced organ motion to 5–10 mm [12, 13]. Several additional studies have shown that liver tumor motion can be reduced to <1 cm using this technique [14, 15]. In a study from the University of Texas Southwestern, 4D-CT scans were performed on 10 patients using either no compression, medium compression, or high compression [14]. A special load cell which calculated the force applied was used to define and reproduce the two levels of compression similarly in each patient. Medium compression and high compression reduced tumor motion in the superior-inferior direction by a mean of 40 and 50 %, respectively. One pitfall of this technique is the possibility for liver shape deformation. However, a recent study from the Princess Margaret Hospital (PMH) demonstrated that inter-fraction liver-to-liver misregistrations due to deformation were less than 5 mm for most patients [16].
Active breathing control (ABC) is a method of controlled breathing which requires patient training to attempt to turn the beam on only during a favorable point in the breathing cycle. This can be difficult due to patient co-morbidities which limit their control over respiration. Respiratory gating utilizes computerized video tracking, typically of the chest wall, to time the beam activation to a certain point in the breathing cycle. Several early studies demonstrated the feasibility of this technique with very good inter-fraction reproducibility [5, 17]. Further advancing this technique, a study from PMH utilized both daily imaging with ABC [18]. Adjustments and repositioning was performed for any positioning errors greater than 3 mm. A total of 109 of 120 fractions delivered to a total of 20 patients required this repositioning. Using this technique, they demonstrated an average reduction in absolute systematic errors from 4.1 mm (cranial–caudal), 2.4 mm (anterior–posterior), and 3.1 mm (medial–lateral) to 1.1 mm (CC), 1.3 mm (AP), and 1.6 mm (ML).
5 Image-Guidance
Accounting for motion with an ITV invariably increases the volume of treated liver. One method of increasing accuracy of the treatment delivered and reducing treated tissue is the use of adaptive image-guidance to reduce systematic uncertainty. There are, generally, two forms of adaptive image-guidance: offline and online. Offline image-guidance consists of daily or weekly review by the physician of images taken at the time of treatment delivery. Adjustments are then made in the next day treatment as needed. These typically consist of small shifts in patient position but can be as drastic as creation of a new radiation plan. While this can be a very useful tool, it does not allow for real-time adjustments prior to delivery. If daily fractions are small, this is likely of little consequence.
As daily doses have increased to as high as 30 Gy per day, the need for accurate imaging in real time at delivery has become essential. Online imaging consists of imaging done at treatment which is reviewed by the physician prior to delivery. While this can be as simple as non-diagnostic megavoltage imaging, newer treatment machines come equipped with onboard imaging (OBI) capable of diagnostic X-rays and even CT. In the era of 2D radiation therapy, daily or weekly megavoltage portal imaging was used to ensure whether the radiation was being delivered to the target. MV imaging, while useful in other disease sites, has limited utility in the upper abdomen as tracking to bony anatomy does not provide for respiratory motion or daily changes in hollow viscera.
The development of treatment machines capable of diagnostic-quality kilovoltage CT scanning (CT-on-rails, cone-beam CT scans, fan beam CT) has expanded this capability tremendously. While most liver tumors do not appear on CT images, gold fiducials implanted near the tumor can be easily tracked each day prior to treatment with image-guidance (Fig. 3
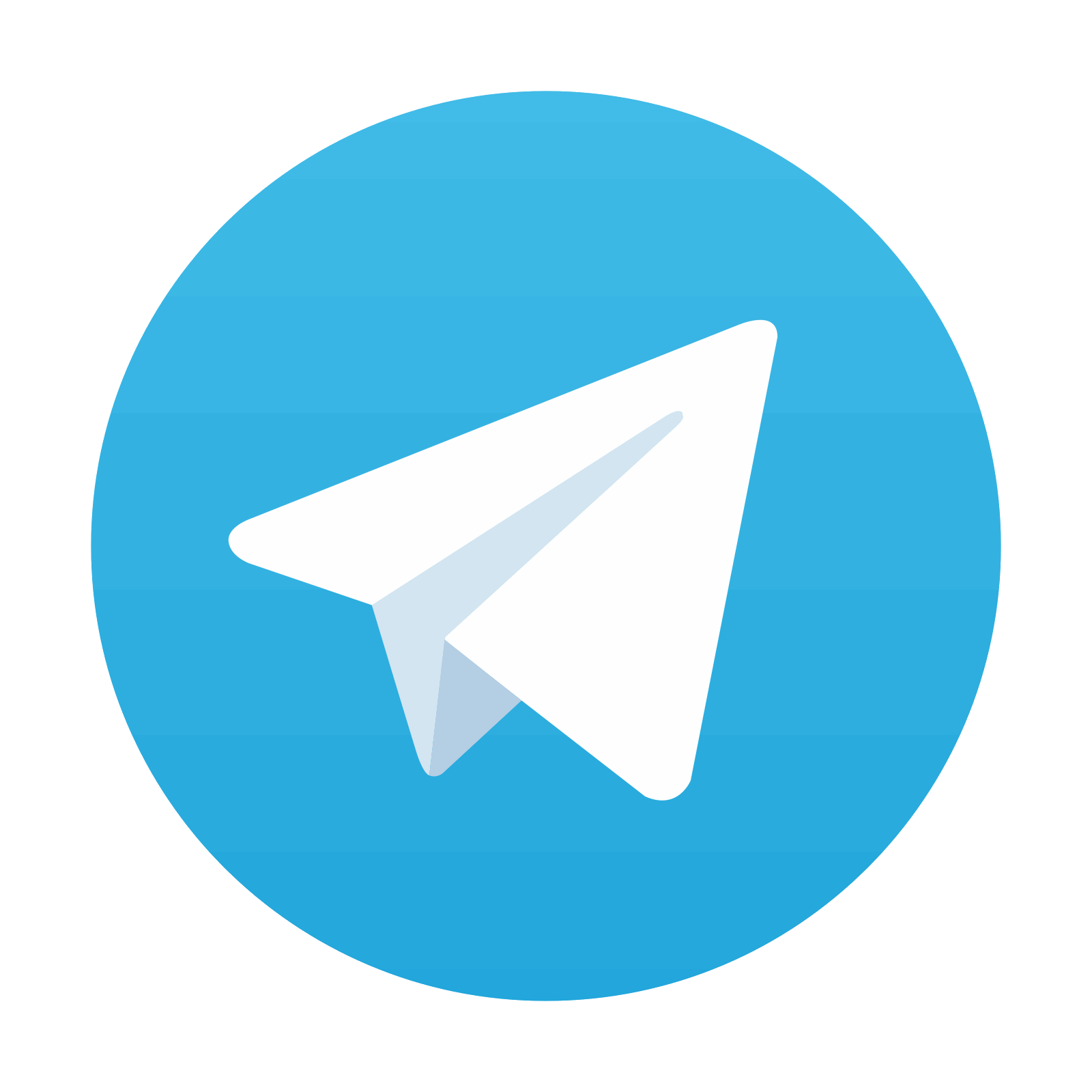
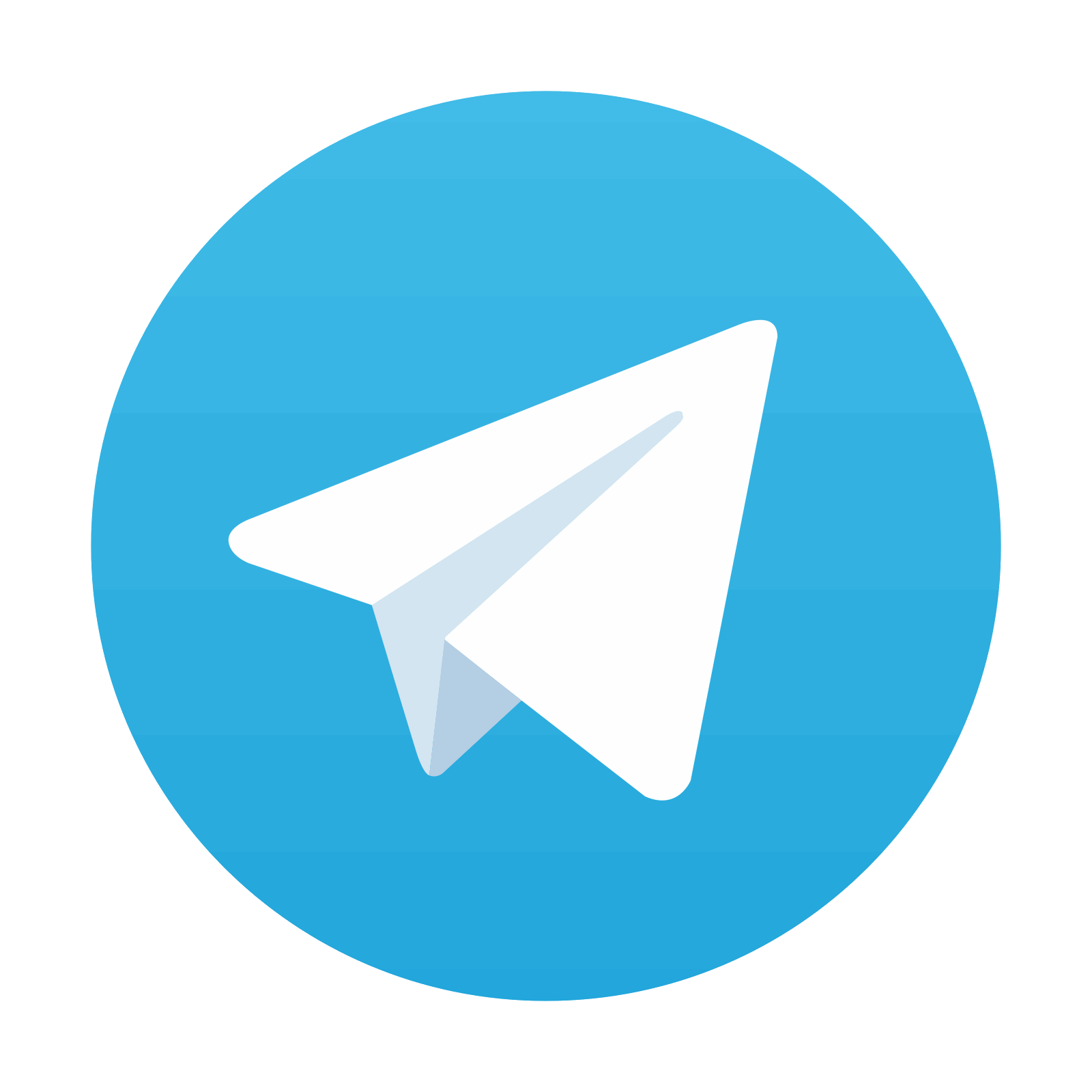
Stay updated, free articles. Join our Telegram channel

Full access? Get Clinical Tree
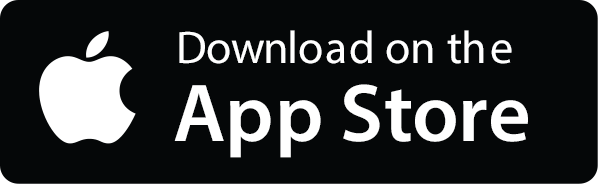
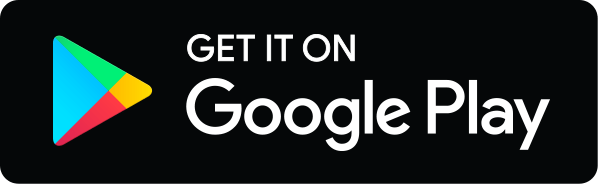