Fig. 2.1
The depth-dose profile of carbon ion beams (290 MeV/u)
Multiple focused beams are usually required to selectively irradiated deep-seated tumors with external X-rays. Intensity-modulated radiotherapy (IMRT) is the most recent development to provide a better dose distribution of such multiple beam radiotherapies [3]. This makes it possible to realize a highly conformal dose distribution and steep dose gradients even with X-rays. However, there are concerns regarding the low-dose bath effect of multiple entry/exiting paths of X-rays, which lead to a larger proportion of the body receiving low-level radiation during IMRT. This is because a high-energy X-ray, which is a form of electromagnetic radiation with no mass or charge, passes through the body very easily and ionizes (deposits energy) its whole pathlength. As a consequence, the normal tissue integral dose (NTID) is higher when this new technique is used. On the other hand, radiotherapy using carbon ion beams can produce a more conformal dose distribution and steeper dose gradients without increasing the NTID, with a smaller number of beams compared with IMRT (Fig. 2.2).
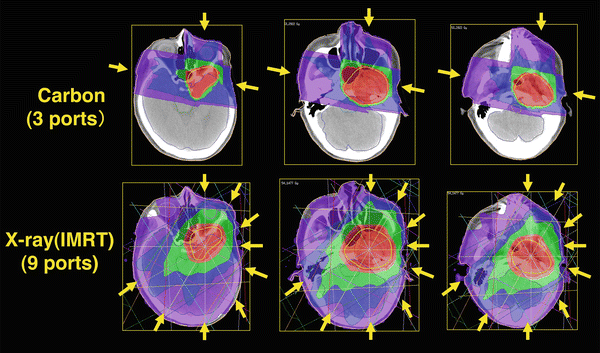
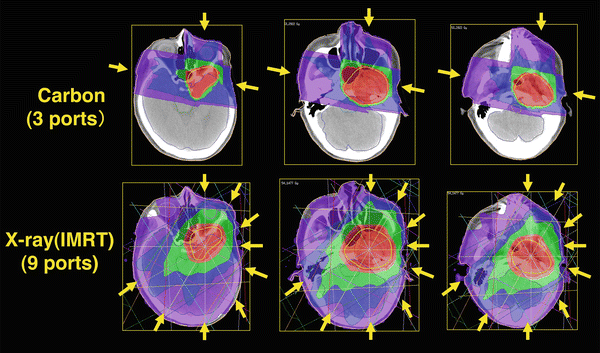
Fig. 2.2
The dose distribution of IMRT using X-rays (left) and carbon ion beams (right). Carbon ion beams could produce a more conformal dose distribution and steeper dose gradients without an increase in the normal tissue integral dose and with a smaller number of beams compared with IMRT
In addition to the definite range, another advantage of the carbon ion is that it travels in almost a straight line, so that sharp and accurate collimation is possible. The lateral scattering is only 1.5 mm at a 20 cm depth in water for the carbon ion beam. This is much smaller than the 6.5 mm for protons. On the other hand, higher energies are required to reach deeply seated tumors for carbon ion beams compared to proton beams.
The magnetic rigidity fixes the bending radius of the particles and determines the size of the accelerator and beam delivery system. The energy and magnetic rigidity of carbon ions are 330 MeV and 5.67 Tm for the 20 cm range in water, while these values for protons are 175 MeV and 2.00 Tm, respectively. As a consequence, a larger accelerator and beam delivery system is required to make a carbon ion facility.
2.3 Biological Advantages and Hypo-fractionation in Carbon Ion Radiotherapy
As a particle (ion) of charge Z, speed v, passes a quasi-stationary electron in a medium, the impulse, the momentum transferred (force x time), is proportional to Z × (1/v). Therefore, the energy transferred is proportional to Z 2/v 2. Hence the ionization density increases as the speed of the particle slows down, until very close to end of the range, when the ionic charge is reduced by electron pickup and the ionization falls rapidly to zero. The high-dose Bragg peak is formed by this phenomenon. Thus, the position of the Bragg peak of the particle can be accurately controlled by its acceleration energy in a known medium. The Z 2 dependence is also very important for therapy. This means that, for equal velocities, the ionization density for the carbon ion (Z = 6, A = 12) is 36 times greater than that of the proton. However, a carbon ion has 12 times more total kinetic energy, so the range of the carbon ion is three times lower.
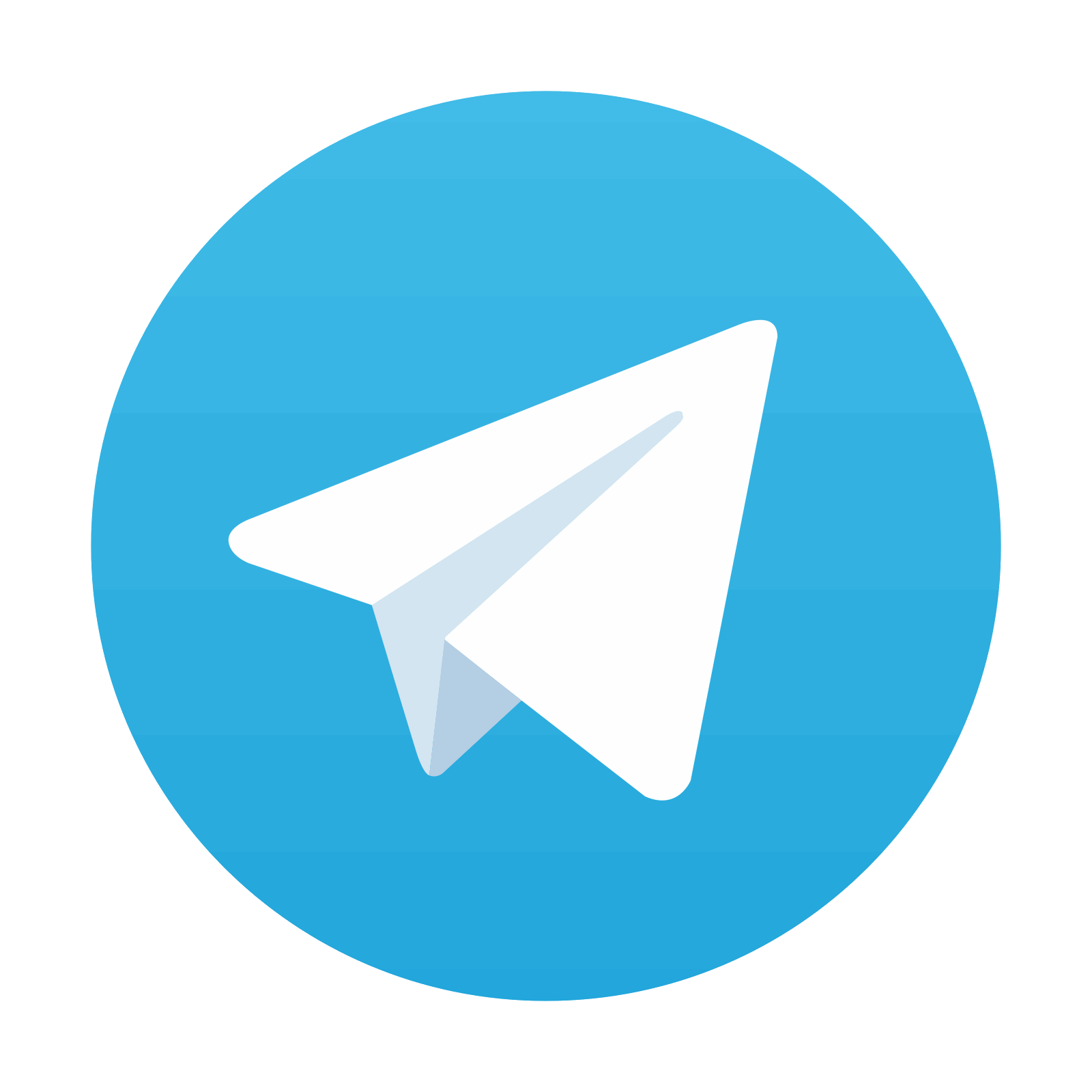
Stay updated, free articles. Join our Telegram channel

Full access? Get Clinical Tree
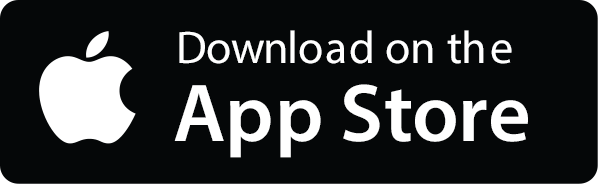
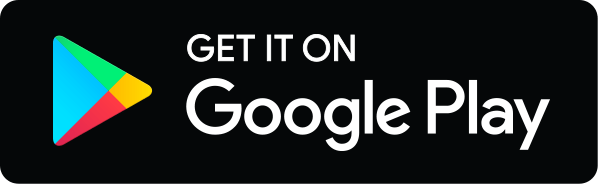