Fig. 10.1
Reconstruction of a phantom slice using two different sampling schemes which are supplementary to each other (panels (a) and (b), respectively). In the standard reconstruction, this would still produce the same artifacts as seen here. The summation of the reconstructions of the complementary data sets is shown in part (c) of the figure. The aliasing artifacts are destroyed due to the principal accuracy of the OPED algorithm
The algorithms developed in the course of the project were tested by assessment of reconstructed images using the “scientific visualizer” tool.
A number of measurements with a Jaszczak phantom (Data Spectrum Corporation, Model # 5000) were performed to study how different reconstruction parameters influence the image quality, in particular to find the optimal number of subsets and iterations required to be used. In addition to the phantom images, also raw data for patients suffering from neuroblastoma or pheochromocytoma and investigated with 123I-metaiodobenzylguanidine (MIBG) were collected. Images were reconstructed for different equivalent number of iterations (defined as the number of subsets times the number of iterations), using the Siemens iterative algorithm Flash 3D. The algorithms ReSPECT (iterative) and OPED (analytic) were adapted for 123I-MIBG examinations and implemented in the “scientific visualizer” platform. Details of this analysis are given in the following section.
Also the new noise reduction methods developed in the MADEIRA project and used in combination with OPED were implemented in ReSPECT. Denoised patient images were reconstructed with ReSPECT and OPED.
10.3 Rank-Order Study
No current image quality criteria are established for nuclear medicine examinations as there are for X-ray images. ROC studies or derivatives thereof are widely used in medical X-ray imaging to define differences in imaging procedures, but ROC studies are time-consuming and require a large patient cohort with normal and pathological subjects, and the right diagnosis for each case should be known. However, if the diagnostic performances are comparable or only slightly different, performing a side-by-side review (rank-order study) may be desirable [16, 17]. In the frame of the MADEIRA project, such a rank-order study was performed for SPECT imaging with 123I-MIBG [18].
SPECT is indeed afflicted with relatively poor spatial resolution and high statistical noise compared to other medical imaging systems. This limitation is partly explained by the restricted amount of reasonable activity, photon attenuation within the patient, scatter, reasonable acquisition time, light scatter in the detector, detector photomultiplier tube configuration, and the trade-off between efficiency and the spatial resolution of the collimator. By choosing an appropriate reconstruction method and optimal reconstruction parameters, opportunities exist for improving image quality and lesion detectability.
To find the optimal conditions for a reconstruction algorithm, several parameters need to be optimized. The number of equivalent iterations (EI) was optimized for the Flash 3D (Siemens Medical Solutions, Forchheim, Germany) [19] reconstruction algorithm and compared to two recently developed reconstruction algorithms, ReSPECT (Scivis GmbH, Gottingen, Germany) [20] and orthogonal polynomial expansion on disc (OPED) [12, 21].
Flash 3D and ReSPECT are iterative algorithms based on the OSEM technique, and OPED is an analytic algorithm. A rank-order study was performed, and the SPECT images were interpreted by three experienced observers, all nuclear medicine physicians, by showing the image sets in the software package “scientific visualizer” installed on one of the department’s regular PACS workstations. The software was adapted for observer studies with the possibility of showing up to eight unlabeled image sets side by side.
The images used for the study were obtained for 11 patients who had underwent SPECT 4 h after intravenous injection of approximately 200 MBq. Additionally, images acquired for 14 patients at 24 h postinjection (p.i.) were also included in the study. The SPECT data for each patient were presented side by side in sagittal, coronal, and transversal views and as a maximum intensity projection in random order and unlabeled. The images were presented in a black-and-white scale, and the observers were free to change the window-level settings. No time limit was imposed on the observer’s evaluation. In the first visual assessment, images reconstructed at eight different EI numbers for Flash 3D were displayed (8, 16, 32, 64, 80, 96, 128 and 256 EI). It should be noted that using too few EI is undesirable as the algorithm may not reach convergence everywhere in the reconstructed volume and lesions will not be visible.
Three observers were asked to rank the three best image sets according to their overall impression of the image quality with regard to noise level, ability to discriminate uptakes in anatomical structures (e.g., liver, adrenal glands, kidneys, and spleen), introduction of artifacts, and if possible delineation of suspected pathology. The rank order was 1 (best) to 3, and the remaining image sets obtained a rank order of 4. This procedure was performed for images acquired 4 h and 24 h after injection. The average distribution of image quality ranking for all observers was calculated for the different EI numbers. In addition, the rank order was obtained based on the calculated average of scores a given image set received, i.e. the lower the value the better the image. In the second visual assessment, the two best considered EI numbers for Flash 3D were compared to ReSPECT and OPED (Fig. 10.2).
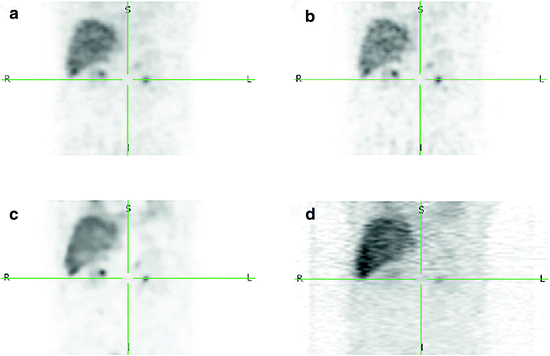
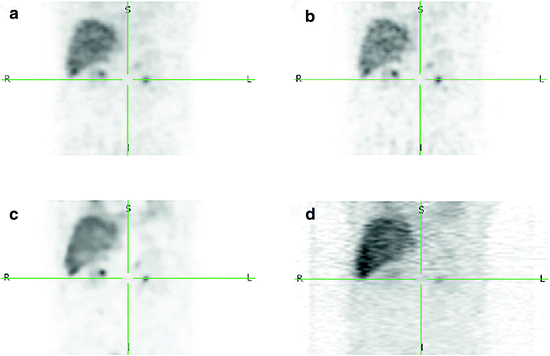
Fig. 10.2
Coronal images acquired 4 h after the injection of approximately 200 MBq 123I-MIBG and reconstructed using different reconstruction methods: Flash 3D32 (a), Flash 3D64 (b), ReSPECT (c), and OPED (d)
In the same manner as the first assessment, the observers were asked to rank the image sets according to their overall impression of the image quality. A rank order of 1 was assigned to the image set judged to have the best overall image quality, and a rank of 4 was assigned to the worst one. The procedure was performed for images acquired 4 h and 24 h after injection. The average distribution of image quality ranking for all observers was calculated for the different reconstruction methods. In addition, the rank order was obtained based on the calculated average of scores that a given image set received.
The optimal EI number for Flash 3D was found to be 32 for both acquisitions at 4 h and 24 h p.i. The average rank order for the different reconstruction algorithms was Flash 3D32 > ReSPECT > Flash 3D64 > OPED for acquisitions at 4 h. Similarly, for acquisitions at 24 h, the order was Flash 3D16 > ReSPECT > Flash 3D32 > OPED.
The algorithm developed the frame of the project shows apparently still potential for improvement. It should however be considered that neither attenuation nor scatter correction was applied in the OPED algorithm. The reconstructed images using OPED were noisy and had streaklike artefacts due to the geometry of the SPECT data. Actually, OPED is more suitable for use in PET and CT [22], since it requires sinusoidal lateral sampling. Instead, the measured SPECT data are parallel in the classical sense, i.e. uniformly distributed projections with equi-spaced lateral sampling, due to the collimation used, and consequently, they need to be resampled for an optimal elaboration with OPED.
The results obtained in this study, although specific for the scanner and parameter settings and depending on the amount of radionuclide activity used, acquisition parameters, acquisition time, and examined body area, give however an indication of preferred reconstruction parameters and algorithms, which need to be further investigated with a larger patient cohort. Therefore, they are useful for future optimization of reconstruction methods and parameter settings.
10.4 The MADEIRA Phantom
In the course of the project, the need of a specific phantom for evaluation of spatial resolution in PET and SPECT imaging was acknowledged. Nowadays, the majority of phantoms used to characterize a SPECT or a PET system are cylinders containing fillable inserts, e.g., the Jaszczak PET/SPECT phantom [23] and NEMA IEC body phantom setTM [24], or phantoms consisting of multiple discrete disks or sheets, e.g., the Hoffman phantom [25] and the porous phantom [26]. Tissue-equivalent anthropomorphic phantoms, as the RSD Alderson heart/thorax phantom, are also available [27]. These phantoms however cannot give detailed and precise information regarding spatial resolution and specifically the onset of the so-called partial volume effect (PVE).
An ad hoc phantom was therefore devised. A preliminary version of the phantom (named MADEIRA phantom) (see Fig. 10.3, left) consisted of 21 cones with separate valves for filling with radioactive solution. The cones were placed within a half-cylindrical surrounding vessel, which is also fillable with radioactive solution for simulating a low activity background. The phantom was constructed of acrylic glass for visual inspection of bubble-free filling.
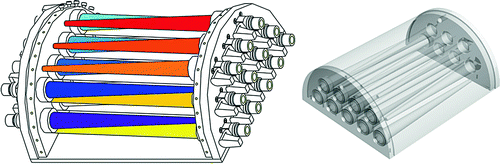
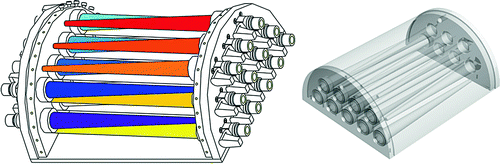
Fig. 10.3
The MADEIRA phantom. Left: first design; right: design used for the tests shown here
The phantom was developed by Scivis in cooperation with the Medical Radiation Physics Department in the Skåne University Hospital Malmö as a clinical partner. It was designed to specifically fit into the RSD Alderson thorax phantom, so that it could be used for a standardized testing in a clinically relevant situation.
Preliminary tests highlighted some construction and leakage problems, which were corrected in the second version of the phantom, consisting of 16 cones of 19 cm length and with an inner diameter linearly decreasing from 16 mm to 2 mm. The wall thickness of the cones is 1 mm. Ideally, the cone walls should have non-zero thickness to prevent the so-called wall effect, i.e. the fact that a shell of zero activity divides the cone solution and the background solution. This may affect quantitative measurements and evaluation of lesion detection, but it is believed to be negligible concerning the evaluation of PVE. The chosen thickness of 1 mm was a practical compromise to guarantee the fabrication of the phantom and at same time to minimize the “wall effect”.
The phantom was employed for measurements in a clinical environment. SPECT (99mTc) and PET (18F) measurements were performed, with the cones filled with activity levels differing by a factor of 3/4 from one to the other. The external vessel was filled with a background activity concentration which was one-tenth of the concentration in the most active cone and ten times the concentration in the less active cone. Consequently, eight cones contained activity below background and eight cones activity above background. Initial activities at the start of acquisition were 530 MBq for 99mTc and 145 MBq for 18F.
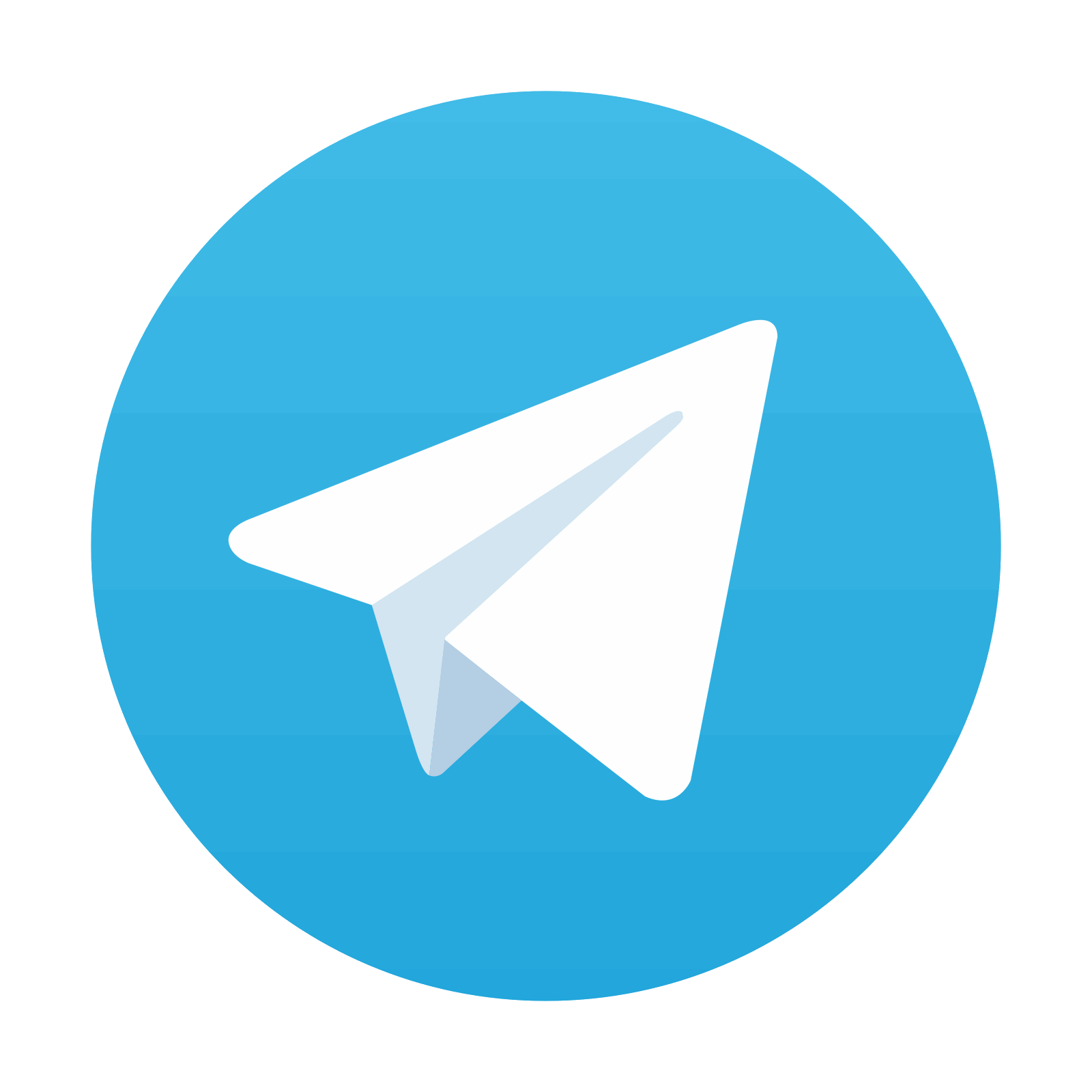
Stay updated, free articles. Join our Telegram channel

Full access? Get Clinical Tree
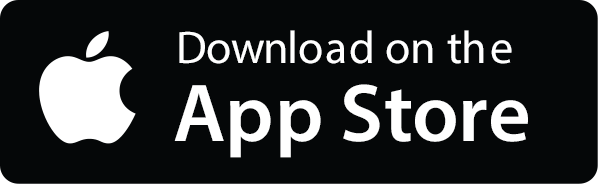
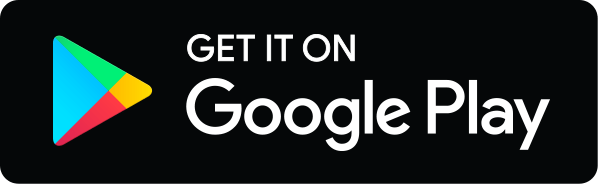