Incidence
Cause
American study
Italian study
HCM
36.3
2.0
Coronary artery anomalies
17.2
12.2
Myocarditis
8.3
6.1
ARVD
5.9
22.4
Ion channel
4.3
MVP
3.6
10.2
Myocardial bridge
3.5
4.1
Coronary artery disease
3.3
18.4
Aortic rupture
3.3
2.0
Aortic stenosis
2.8
Dilated cardiomyopathy
2.5
2.0
Congenital heart disease
1.2
Myocardial infarction
1
Conduction system disease
2.3
8.2
Possible HCM
17.2
44.1.1 Myocardial Function/Dysfunction in Athletes in Endurance and Power Sport
The heart is a striated muscle with similarities and dissimilarities compared to the striated skeletal muscle. Although both muscle cell types contain actin and myosin filaments, there is a large difference in the structural organisation of the cells. Cardiac myocytes in contrast to skeletal myocytes form a syncytium. This implies a propagation of action potentials after the activation of one of its cells.
Adaptation of the heart muscle in response to exercise has been extensively described. Different forms of sport may imply a different strain to the heart. In this context endurance sports are considered to induce a dynamic, isotonic workload, which is accompanied by an increase in heart rate and stroke volume and a reduction in peripheral vascular resistance. Strength sports on the other hand induce a static, isometric workload, which is accompanied by only a small increase in heart rate and stroke volume and a high increase in systolic and diastolic blood pressure. In short, endurance sports result predominantly in a volume load and strength sports in a pressure load for the heart. Despite the differences in load, it is generally accepted that all forms of high-level sport which result in an athletes’ heart are characterised by left ventricular hypertrophy, which may be eccentric or concentric. In the eccentric form of LV hypertrophy, the LV cavity sizes tend to be larger than controls. Wall thickness in strength athletes tend however to be higher than in endurance athletes assigned as the concentric form (Prior and La Gerche 2012). Interestingly, the adaptation of the cardiac muscle in response to exercise is regarded as physiological remodelling. This means that the hypertrophy is characterised by a normal myocardial architecture with a normal myocyte–capillary ratio and without fibrosis.
The effect of strenuous exercise on the right ventricle. In contrast to LV function, RV function has long been underexposed. Maybe this was caused by the limited modalities to image and evaluate RV function. Retrospectively it is very curious that RV function was long neglected; most ventricular arrhythmias originate from the RV and an excessive increase of cardiac output (CO) is not only by an increase of the stroke volume of the LV but also of the RV. Studies of Corrado stressed the importance of arrhythmogenic RV cardiomyopathy (ARVC) as the main cause of SUD in athletes in Italy (Corrado et al. 2006). The group of La Gerche, demonstrating the development of RV dysfunction and structural remodelling, performed important work on the aetiology of RV dysfunction (La Gerche et al. 2011). Important results were generated by imaging techniques, not only echocardiography but especially with cardiac magnetic resonance imaging (CMR).
The RV is wrapped around the LV septum and is differently shaped. The shape and the thin wall make it difficult to study RV function by traditional imaging techniques. In contrast to the LV, pressure is lower and filling partly dependent on the negative intrathoracic pressure during inspiration facilitates venous return from venous (low pressure) system. Although pressure is much lower, the stroke volume (SV) is generally equal to the LV. In maximal exercise SV can increase from 60–80 cc to 100–125 cc in untrained but in athletes up to even a 200 cc (Gurtner et al. 1975). This high volume can be accommodated in the systemic circulation by arterial vasodilation especially in the muscles and the skin. Due to a decrease in afterload, the increase of LV pressure and strain on the OV wall is limited. In contrast, the capacity of vasodilation in the pulmonary circulation is limited and its maximum will be reached soon, already at submaximal exercise intensity levels. A substantial increase in pulmonary arterial pressure is a consequence of the large increase of SV during maximal exercise, leading to a substantial increase not only of preload but especially of afterload. The La Gerche group has demonstrated the consequences of this volume and pressure overload. After intense endurance exercise, they demonstrated acute dysfunction of the RV (in contrast with a persistent normal LV function) and a significant rise of serum troponin levels. With DGE-CRM, they demonstrated myocardial fibrosis of the RV in endurance athletes (La Gerche et al. 2012).
Using modern echocardiography techniques, it has become clear that the right ventricle in athletes shows a profound adaptation (La Gerche et al. 2011). Right ventricular mass as well as volumes increase especially in endurance athletes (Aaron et al. 2011). Right ventricular abnormalities found in athletes may actually mimic right ventricular arrhythmogenicity (Ector et al. 2007). All in all, left as well as right ventricular adaptations and abnormalities may develop in athletes.
44.1.2 Anabolic–Androgenic Steroids (AAS)
Anabolic–androgenic steroids (AAS) are abused for enhancing muscle mass, strength growth and improving athletic performance. In recent years many observational and interventional studies have shown important adverse cardiovascular effects of AAS abuse.
Cardiovascular adverse effects of AAS abuse have been reported sporadically as case reports of hypertension (Stergiopoulos et al. 2008), myocardial infarction (MI) and stroke (Stergiopoulos et al. 2008), dysrhythmia (Angelilli et al. 2005), cardiomyopathy (Ahlgrim and Guglin 2009) and sudden cardiac death (Fineschi et al. 2007) in bodybuilders with long-term AAS abuse in the recent years. Case reports on hard atherosclerotic end points (sudden cardiac death, MI or stroke) comprise young AAS abusers without pre-existent cardiac risk factors, suggesting that a high AAS dose imposes additional independent risk to conventional cardiovascular risk factors.
Growth hormone (GH) has also a significant role in cardiovascular function. Acromegaly, a state of endogenous GH excess, results in myocardial hypertrophy and decreased cardiac performance with increased cardiovascular mortality. Additional insight into the role of excess GH on the cardiovascular system has been gained from data collected in athletes doping with GH. Likewise, however important, GH abuse and cardiovascular risk in athletes will be beyond the scope of this chapter.
44.1.3 Imaging with Nuclear Medicine with or Without Combined CT Techniques and CMR
Imaging with nuclear medicine with or without combined CT techniques and CMR is a promising method to target and image certain biomarkers in the process of cardiovascular pathology that can be used for early detection of sport- and also AAS-associated adverse effects. A number of modalities and tracers are currently being developed that may become useful to delineate functional abnormalities in several pathways involved in sport- and AAS-induced cardiovascular injury at the preclinical and clinical level. Single-photon emission computed tomography (SPECT/CT) and positron emission tomography (PET/CT) are nuclear imaging cameras for the evaluation of several cardiovascular processes using different radiopharmaceuticals. Cardiovascular magnetic resonance (CMR) can be used for anatomical structures related to cardiovascular diseases and also for functional imaging. Important cardiovascular imaging items are myocardial ischemia, viability, left and right ventricular ejection fraction (LVEF and RVEF, respectively), myocardial innervation, atherosclerosis, valve abnormalities (CMR) and flow velocities (CMR).
This book chapter discusses the physiological background of myocardial (dys)function in athletes and established and novel nuclear molecular imaging techniques, including MRI, that may serve as potential tools for early detection of sport- and AAS-associated cardiovascular disorders and reducing cardiovascular sudden deaths in athletes.
44.2 Nuclear Medicine and CMR Imaging of Heart Disorders in Athletes
Nuclear medicine techniques such as SPECT/CT, PET/CT and gated blood pool MUGA can be used for function, perfusion, viability and molecular imaging. Myocardial perfusion imaging (MPI) SPECT using 99mTc-labelled myocardial perfusion tracers is an established imaging technique that is already an integral part of managing several heart diseases and is included in a number of professional guidelines. For instance, many studies have assessed the diagnostic accuracy of MPI for the detection of coronary artery disease using a combination of rest and (adenosine or bicycle) stress MPI SPECT (Hendel et al. 2010; Kapur et al. 2002; Koh et al. 2011). The widespread application of 99mTc-labelled myocardial perfusion tracers and the data processing capacity have made ECG-gated SPECT imaging part of the clinical routine in nuclear imaging laboratories (Germano et al. 1995). ECG-gated tracers using 99mTc-labelled perfusion tracers permit assessment of regional myocardial wall motion and wall thickening throughout the cardiac cycle, due to their high count rates and stable myocardial distribution with time. The automatic quantification of LV volumes, ejection fraction (EF), regional myocardial wall motion and thickening from gated SPECT is rapid and accurate, with minimal operator interaction, and is therefore widely used. In a large cohort of unselected patients with known or suspected ischemic heart disease, the values of LVEF routinely measured by echocardiography, gated SPECT and LV angiography were closely correlated and provided a powerful prognostic information that was incremental to clinical variables for gated SPECT (Gimelli et al. 2008). PET imaging is an established technique for absolute myocardial perfusion quantification (Slart et al. 2011; Tio et al. 2009a, b) and to distinguish viable from scarred myocardium (Slart et al. 2005, 2006); however its dependency of cyclotrons and high costs have limited the widespread use in clinical practice. PET has however several technical advantages over gamma-technique SPECT such as higher counting sensitivity, higher spatial resolution and absolute quantification of myocardial perfusion flow and metabolism by using 18F-fluorodeoxyglucose (18F-FDG) (Slart et al. 2004). Also LV function can be quantified by gated PET techniques, using myocardial perfusion tracers such as 13N-ammonia, rubidium-82 (82Rb) or the viability tracer (18F-FDG). MUGA (multigated acquisition scan) is a relative old technique for LV function assessment but is highly reproducible (van der Vleuten et al. 2005). Myocardial perfusion SPECT and PET can be combined with calcium scoring angiography of the coronary arteries by adding CT technology. This can be performed on the hybrid SPECT/CT and hybrid PET/CT camera in a single procedure. Functionality (myocardial perfusion) and morphology (calcium deposition and coronary stenosis) will be combined in one session. Myocardial perfusion and calcium scoring are partly related in the diagnosis of cardiovascular disease and, combined, showed to be a strong prognostic predictor for cardiovascular events (Danad et al. 2012; Ghadri et al. 2012). Red blood cells are labelled with 99mTc-pertechnetate after the injection of stannous chloride (in vivo method most commonly used), and gamma camera acquisition is performed during ECG gating. The resulting images show that the volumetrically derived blood pools in the chambers of the heart and timed images may be computationally interpreted to calculate the ejection fraction and injection fraction of the heart.
Molecular imaging differs from traditional nuclear imaging in that probes known as biomarkers are used to help image particular targets or pathways. Biomarkers interact chemically with their surroundings and in turn alter the image according to molecular changes occurring within the area of interest. For instance, myocardial sympathetic innervation related to arrhythmias 123I-MIBG can be visualised and quantified with 123I-metaiodobenzylguanidine 123I-MIBG SPECT or 11C-metahydroxyephedrine (11C-mHED) PET and 11C-CGP-12388 PET for β-adrenoceptor quantification (Bengel and Schwaiger 2004; de Jong et al. 2005; Flotats et al. 2010). There is a growing body of literature on the utility of 123I-MIBG for patient risk stratification and its potential role in important management decisions such as for cardiac resynchronisation therapy and defibrillator placement (Kelesidis and Travin 2012).
Other cardiovascular targets that can be visualised and quantified with nuclear molecular imaging are angiogenesis, inflammation, proteolysis, apoptosis, fatty acid metabolism and stem cell therapy (Bengel et al. 2009; Majmudar and Nahrendorf 2012; Miyagawa et al. 2005; Stacy et al. 2012). For the assessment of cardiac function and morphology, cardiovascular magnetic resonance imaging (CMR) allows also a multifaceted approach to cardiac evaluation by enabling an assessment of anatomy, function, perfusion, viability, tissue characterisation and blood flow during a single comprehensive examination (Ibrahim 2012). Delayed-enhancement (DE)-CMR using gadolinium contrast agent provides a direct assessment of myopathic processes. This permits a fundamentally different approach than that traditionally taken to ascertaining the aetiology of cardiomyopathy, which is vital in patients with nonischemic cardiomyopathy and incidental coronary artery disease and patients with mixed, ischemic and nonischemic, cardiomyopathy. Precise tissue characterisation with DE-CMR also improves the diagnosis of left ventricular thrombus, for which it is the emerging clinical reference standard. Velocity-encoding CMR is used for measuring blood flow. Velocity-encoding images are used for evaluating the valvular and vascular conditions (Mohiaddin et al. 1991).
44.2.1 Ventricular Dysfunction
The athlete’s heart is commonly characterised by an increase in left ventricular mass because of an increase in the left ventricular diastolic cavity dimensions or wall thickness or both. A previous study examined the cardiac structure and function of a unique cohort of documented lifelong, competitive endurance veteran athletes (>50 year) (Wilson et al. 2011). Twelve lifelong veteran male endurance athletes [mean ± SD (range) age: 56 ± 6 years (50–67)], 20 age-matched veteran controls [60 ± 5 years (52–69)] and 17 younger male endurance athletes [31 ± 5 years (26–40)] without significant comorbidities underwent cardiac magnetic resonance (CMR) imaging to assess cardiac morphology and function, as well as CMR imaging with late gadolinium enhancement (LGE) to assess myocardial fibrosis. Lifelong veteran athletes had smaller left (LV) and right ventricular (RV) end-diastolic and end-systolic volumes (p < 0.05) but maintained LV and RV systolic function compared with young athletes. However, veteran athletes had a significantly larger absolute and indexed LV and RV end-diastolic and end-systolic volumes, intraventricular septum thickness during diastole, posterior wall thickness during diastole and LV and RV stroke volumes (p < 0.05), together with significantly reduced LV and RV ejection fractions (p < 0.05), compared with veteran controls. In six (50 %) of the veteran athletes, LGE of CMR indicated the presence of myocardial fibrosis (four veteran athletes with LGE of non-specific cause, one probable previous myocarditis and one probable previous silent myocardial infarction). There was no LGE in the age-matched veteran controls or young athletes. The prevalence of LGE in veteran athletes was not associated with age, height, weight or body surface area (p > 0.05) but was significantly associated with the number of years spent training (p < 0.001), number of competitive marathons (p < 0.001) and ultra-endurance (>50 miles) marathons (p < 0.007) completed. An unexpectedly high prevalence of myocardial fibrosis (50 %) was observed in healthy, asymptomatic, lifelong veteran male athletes, compared with zero cases in age-matched veteran controls and young athletes. These data suggest a link between lifelong endurance exercise and myocardial fibrosis that requires further investigation. Another study in endurance athletes examined the influence of exercise on the RV function (Schattke et al. 2012). After exercise, the RV volumes decrease significantly when measured by CMR compared to baseline. This was confirmed by a study of la Gerche et al., in which they found that exercise induces a relative increase in wall stress of the RV which exceeds LV wall stress in athletes (La Gerche et al. 2011). Also in athletes, greater RV enlargement and greater wall thickening may be a product of this disproportionate load excess.
Athletes show a significant decrease in ventricular volumes and LV wall mass with increasing age, which probably reflects decreasing training intensity rather than the effect of age (Prakken et al. 2011). Mature athletes form a distinct group requiring separate reference values as they have significantly lower ventricular volumes and wall mass as compared to young athletes, however, still significantly higher values than mature non-athletes. Cardiac MRI reference values showed also increased ventricular volumes, diameters, wall mass and wall thickness for endurance athletes compared with non-athletes. High training hours/week and male sex resulted in an increased overlap with standard thresholds for cardiomyopathy (Prakken et al. 2010).
44.2.2 Coronary Artery Evaluation
Several causes of sudden death in athletes arise from the coronary arteries. They may be classified as either congenital or acquired. Coronary arteries can be imaged with invasive conventional coronary angiography and reveals the location and severity of obstructive lesions using contrast agent. New advances in cardiac multi-detector computed tomography (MDCT) these days mean invasive angiography is no longer the initial investigation for suspected coronary artery anomalies. Technical difficulties were encountered related to the small size and almost constant motion of the coronary vessels throughout the cardiac cycle. The high spatial and temporal resolution of current cardiac CT generations overcomes many of these challenges, allowing coronary artery evaluation. A meta-analysis by Vanhoenacker et al. (2007) found that the pooled sensitivities and specificities of 64-detector coronary CT, 16-detector coronary CT and four-detector coronary CT for detecting a significant stenosis (>50 % per segment) were 93 and 96 %, 83 and 96 % and 84 and 93 %, respectively.
These days MDCT can be combined with functional PET or SPECT imaging, using hybrid PET/CT and SPECT/CT cameras (Kaufmann and Di Carli 2009). Whereas MDCT has the advantage of detecting coronary atherosclerosis at its earliest stages, thereby allowing initiation of appropriate therapeutic measures well before development of obstructive CAD, myocardial perfusion imaging (MPI)-SPECT can clarify the hemodynamic consequences of the anatomic findings on MDCT based on a functional assessment of myocardial blood flow.
The largest of these studies using integrated PET and 64-detector CT prospectively enrolled 107 patients with chest pain with intermediate likelihood of CAD and compared hybrid 15O-water PET and CT angiography (CTA) versus CTA alone in the detection of haemodynamically significant coronary stenosis (Kajander et al. 2010). Hybrid imaging was technically feasible and scan time remained short, because single 6-min PET scan during adenosine stress was used. The haemodynamic significance of stenoses was defined by quantitative coronary angiography including FFR measurement of intracoronary pressure gradient when feasible. Although both PET and CTA alone demonstrated high (97 %) NPV, CTA alone was suboptimal in assessing the severity of stenosis (PPV 76 %) and perfusion imaging alone could not separate microvascular disease from epicardial stenoses (PPV 77 %) in all patients. The use of PET/CTA significantly improved diagnostic accuracy to 98 %. Sensitivity, specificity, PPV and NPV were 93, 99, 96 and 99 %, respectively. This indicates that non-invasive hybrid PET/CTA imaging is a highly accurate diagnostic method for the detection of haemodynamically significant CAD compared with PET or CTA alone and may be beneficial in athletes.
Hybrid SPECT/CT may play also an important role in optimised risk stratification of patients with acute coronary syndrome (ACS) and providing an objective decision-making tool for tailoring the future therapeutic strategy as demonstrated in a study of Rispler et al. (Rispler et al. 2011). That study showed no negative effect on prognosis in patients with non ST-elevated ACS and also avoided unnecessary revascularisation procedures.
44.2.3 Microvascular Myocardial Perfusion in Athletes
It is possible to make quantitative measurements of myocardial blood flow (MBF) and myocardial perfusion reserve (MPR) with the use of PET. Owing to high temporal resolution and correction of photon attenuation, PET provides accurate delineation of regional tracer kinetics in the blood pool within the left ventricle cavity and myocardium, which are used in combination with tracer kinetic models to quantify MBF in mL/g/min of tissue. Recently, continued improvements in PET technology have contributed to the growing interest in translation of quantitative flow and flow reserve using PET from mainly a research tool to routine clinical practice. Routine list-mode acquisition of myocardial perfusion PET scan enables easy combination of flow quantification with the traditional MPI parameters, which facilitates implementation of quantitative analysis. Because of technical challenges, very little is known about absolute myocardial perfusion in humans in vivo during physical exercise. In the present study positron emission tomography (PET) was applied in order to (1) investigate the effects of dynamic bicycle exercise on myocardial perfusion and (2) clarify the possible effects of endurance training on myocardial perfusion during exercise. Myocardial perfusion was measured in endurance-trained and healthy untrained subjects at rest and during absolutely the same (150 W) and relatively similar [70 % maximal power output (Wmax)] bicycle exercise intensities. On average, the absolute myocardial perfusion was 3.4-fold higher during 150 W (p < 0.001) and 4.9-fold higher during 70 %W (max) (p < 0.001) than at rest. At 150 W myocardial perfusion was 46 % lower in endurance-trained than in untrained subjects (1.67 ± 0.45 vs. 3.00 ± 0.75 ml × g(−1) × min(−1); p < 0.05), whereas during 70 %W (max) perfusion was not significantly different between groups (p = not significant). When myocardial perfusion was normalised with rate–pressure product, the results were similar. Thus, myocardial perfusion increases in parallel with the increase in working intensity and in myocardial work rate. Endurance training seems to affect myocardial blood flow pattern during submaximal exercise and leads to more efficient myocardial pump function (Laaksonen et al. 2007). In the study of Sörensen et al. (2010), 11C-acetate PET applied was used at rest for measuring absolute myocardial blood flow (MBF) and oxidative metabolic rate (k(mono)) of the coronary arteries and microvascular capillaries. They evaluated the feasibility of quantitative 11C-acetate PET during exercise. Five endurance athletes underwent dynamic PET scanning at rest and during supine bicycle stress. Exercise was maintained at a workload of 120 W for 17 min. The rate–pressure product (RPP) was recorded repeatedly. MBF, k(mono) in the left (LV) and right (RV) ventricular wall, cardiac output (CO), cardiac efficiency and a lung uptake value reflecting left heart diastolic pressures were calculated from the PET data using previously validated models. MBF increased from 0.71 ± 0.17 to 2.48 ± 0.25 ml min(−1) per ml, LV-k(mono) from 0.050 ± 0.005 to 0.146 ± 0.021 min(−1), RV-k(mono) from 0.023 + 0.006 to 0.087 + 0.014 min(−1), RPP from 4.7 ± 0.8 to 13.2 ± 1.4 mmHg × min(−1) × 10(3) and cardiac output from 5.2 ± 1.1 to 12.3 ± 1.2 l min(−1) (all p < 0.001). Cardiac efficiency was unchanged (p = 0.99). Lung uptake decreased from 1.1 ± 0.2 to 0.6 ± 0.1 ml g(−1) (p < 0.001). That study showed that a number of important parameters related to cardiac function can be quantified non-invasively and simultaneously with a short scanning protocol during steady-state supine bicycling. This might open up new opportunities for studies of the integrated cardiac physiology in health and early asymptomatic disease based on coronary and microvascular angiopathy (Sorensen et al. 2010).
44.2.4 Hypertrophic Cardiomyopathy
Echocardiography (echo) and CMR has a central role in the structural and functional assessment of HCM. Several parameters can be examined: (1) the presence/absence of LVH together with its extent and degree, (2) the mitral valve, (3) the left ventricular outflow tract, (4) systolic function and (5) diastolic function. Echocardiography can suffer from poor acoustic windows, incomplete visualisation of distant areas of the left ventricular wall and poor assessment of left ventricular mass in those with focal asymmetric hypertrophy. CMR can overcome these limitations and more accurately assess wall thickness, distribution of hypertrophy and ventricular mass and function. It can also utilise the diagnostic power of delayed enhancement following gadolinium contrast administration and accurately differentiate athlete’s heart from HCM (Fig. 44.1). Diagnosing HCM in the athlete population poses difficulty as it needs to be distinguished from cardiac hypertrophy that usually occurs physiologically in the same population (“athlete’s heart”) (Maron et al. 1995). Although there is no minimum wall thickness that excludes HCM, the commonest diagnostic criterion of HCM is a left ventricular wall thickness of ≥15 mm (Maron et al. 2003). In the majority of athletes, wall thickness is normal or less than 12 mm, while in a small few it can be up to 16 mm (Spirito et al. 1994). Of the population of patients who have HCM, an important minority has mild hypertrophy of 13–15 mm. This area of overlap leads to a diagnostic dilemma as to whether the observed wall thickness is pathological HCM or physiological (Maron 1986). Clinical information, echocardiography and, in particular, CMR have an important role to play in answering this question (Maron et al. 1995; Petersen et al. 2005). The pattern of hypertrophy in the cardiac wall is important. In trained athletes, thickening of all wall segments are similar (within 1–2 mm) with the dominant area being the anterior septum (Maron et al. 1995). HCM can sometimes be distinguished from athlete’s heart purely on the end-diastolic volume of the ventricles (Maron et al. 1995). An enlarged end-diastolic cavity (greater than 55 mm) is present in a third of elite athletes (Spirito et al. 1994), while, in contrast, in HCM, the cavity is usually small (less than 45 mm) unless in the end stages of heart failure where dilatation occurs (Maron et al. 2009). CMR has been demonstrated in a single-centre study to be able to differentiate between athlete’s heart and other causes of ventricular hypertrophy (Petersen et al. 2005). In an eloquent study using a variety of geometric measurements from CMR, Petersen et al. (2005) determined that left ventricular diastolic wall thickness divided by left ventricular end-diastolic volume was the best parameter to distinguish athlete’s heart from HCM. A ratio of <0.15 was 80 % sensitive and 99 % specific for identifying athlete’s heart with a positive predictive value of 95 % and a negative predictive value of 94 %. No single geometric index was 100 % accurate in identifying athlete’s heart. However, using all volumetric and geometric parameters recorded (nine in total) in multiple logistic regression analyses, CMR could identify athlete’s heart with 100 % accuracy (Petersen et al. 2005). An important aspect in the evaluation of athlete’s heart is the importance of the particular sport engaged in as the type and degree of changes in ventricular parameters vary greatly between sports (Baggish et al. 2008; Pelliccia et al. 1991, 1993). Baggish et al. (2008) examined cardiovascular changes observed in those involved in endurance training (rowing) or strength training over 90 days. Their findings in the endurance-training group showed left and right ventricular dilation, enlargement of both atria and enhanced left and right ventricular diastolic function (Baggish et al. 2008). Those involved in strength training showed increased left ventricular thickness (all less than 12 mm) and reduced left ventricular diastolic function (Baggish et al. 2008). Pelliccia et al. (1991) in a study of 1.000 athletes in Italy demonstrated that only 2 % had left ventricular thickness of greater than 12 mm of which all except one case were attributable to rowing or canoeing (the remaining case was a cyclist). Absolute increases in wall thickness were not beyond 12 mm in any other disciplines including those involving strength training, such as weight lifting (Baggish et al. 2008; Pelliccia et al. 1993). Patients with HCM might have epicardial coronary artery disease, and many HCM patients with chest pain undergo stress perfusion SPECT imaging to detect ischaemia. Treadmill, bicycle exercise, atrial pacing, dipyridamole and adenosine were examined in this population. Defects, both reversible and fixed, were noted from 10 to 100 % of the patients imaged. Most defects involve the septum but can occur in other walls. Although the general principles of SPECT interpretation apply to HCM patients, cardiologists should be cautious of “hot spots”. These have increased count activity and are most frequently noted in the septum in patients with asymmetric LVH. The increased count activity might be related to LVH and/or increased regional blood flow. Irrespective of the aetiology of “hot spots”, if the tomographic slices are normalised to this area of increased count activity, regions adjacent to and distinct from the “hot spot” will appear relatively less intense, thereby creating spurious perfusion defects. Inaccurate image interpretation can be avoided by paying attention to the location and type of perfusion defects. The lateral wall is most frequently involved, and perfusion defects are usually fixed. Furthermore, on gated SPECT images, normal regional function will be noted despite the apparently reduced perfusion (Nagueh and Mahmarian 2006). Abnormal myocardial perfusion in HCM patients, despite normal coronary angiography, has been linked to sudden cardiac death (Dilsizian et al. 1993). Anterior and inferior wall defects are so common in healthy athletes with physiological LVH that the specificity of myocardial SPECT for the detection of CAD, in contrast to exercise ECG, seems to be too low for evaluation of chest pain in this group. The mechanism of anterior and inferior defects may be related to hot spots (papillary muscles) in the lateral wall. The specificity of SPECT for the detection of CAD is maintained in athletes without LVH (Bartram et al. 1998).
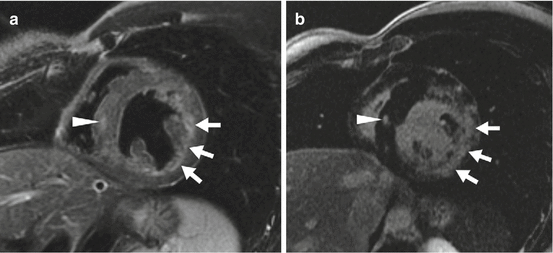
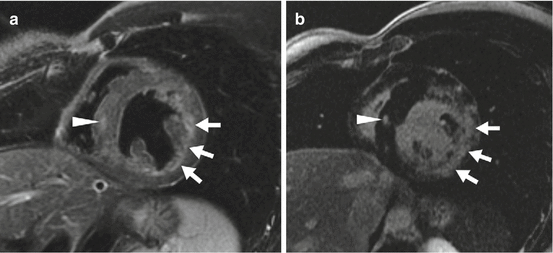
Fig. 44.1
Hypertrophic cardiomyopathy. A 58-year-old man presented with worsening chest pain. Coronary angiography showed non-obstructive coronary artery disease but systolic obliteration of the distal and apical portions of the left ventricle. (a) A vertical long-axis view from cardiac CMR image demonstrates hypertrophy of the apical ventricular segments (arrows) with (b) demonstrating corresponding delayed gadolinium enhancement within the same region (arrows) compatible with myocardial fibrosis. Such fibrosis depiction on CMR has been shown to have prognostic implications for patients with HCM (Arrigan et al. 2011)
More recently, worse prognosis was noted with microvascular dysfunction as determined by myocardial blood flow and coronary flow reserve measured by 13N-labelled ammonia (Cecchi et al. 2003). Impaired coronary reserve (CR) with angiographic coronary arteries has been demonstrated in patients with left ventricular hypertrophy (LVH) in response to valvular heart disease or hypertension. To determine if adaptive LVH induced by intensive training may alter myocardial blood flow (MBF) and CR, 8 highly trained endurance triathletes (29.6 ± 4.0 year, with echographic LVH) were compared with 6 control subjects (33.0 ± 7.9 years, with a normal echographic examination). Triathletes entered the study if they had a left ventricular mass >120 g/m2 at 2-D echocardiographic measurements (mean = 148.6 ± 19.8 g/m2). MBF was assessed using positron emission tomography (PET) with 15O-water. Subjects underwent an intravenous bolus of 17–25 mCi of 15O-water at baseline and after intravenous infusion of 0.80 mg/kg of dipyridamole; 15O-water examination was followed by an (18F-FDG) myocardial imaging. CR was determined as the ratio of maximal to basal myocardial blood flow. In comparison with controls, triathletes with LVH showed normal MBF values (0.74 ± 0.1 vs. 0.8 ± 0.2 ml/ml/min, p = 0.2) but an increased CR (3.8 ± 0.7 vs. 6.1 ± 1.9, p < 0.05). In contrast with other forms of LVH, CR is not altered in LVH due to intense physical training. These results suggest that LVH due to intensive physical training is associated with an increase in coronary blood flow capacity (Toraa et al. 1999).
Aside from perfusion defects, additional abnormalities have been reported, including increased washout rates of 99mTc-tetrofosmin, which is positively correlated with New York Heart Association functional class and wall thickness (Buyukdereli et al. 2005). A number of interesting observations were made with cardiac metabolic and (123I-BMIPP) neurotransmission imaging. 123I-beta-methyl-iodophenylpentadecanoic acid, a marker of fatty acid metabolism 123I-BMIPP, imaging was investigated in Japanese patients with HCM. BMIPP uptake was decreased, whereas its washout was increased in HCM (Zhao et al. 2003), with regional BMIPP dynamics related to regional function and perfusion. Intriguing observations were noted with 123I-MIBG, which tracks the presynaptic uptake and storage of neurotransmitters. In one study, HCM patients with ventricular tachycardia had a significantly higher MIBG global washout rate, which was the most powerful predictor of ventricular tachycardia on multiple, regression analysis (Terai et al. 2003a). Interestingly, a progressive decrease in 123I-MIBG uptake and an increase in its washout rate are noted in HCM patients, as LV size increases and EF decreases (Terai et al. 2003b). Other studies (Lefroy et al. 1993; Schafers et al. 1998) have explored changes in beta-adrenergic receptor density, with the radioactive tracers 11C-mHED and 11C-CGP-12177. These studies demonstrated the presence of reduced beta-adrenergic receptor density with reduced norepinephrine reuptake by presynaptic terminals. The reduction in beta-adrenergic receptor density seems to be particularly prominent in patients with heart failure (Choudhury et al. 1996a, b). Collectively, the aforementioned findings suggest that autonomic dysfunction might play a role in sudden death, disease progression and the development of heart failure in HCM. However, additional studies are needed in a larger number of patients to corroborate these observations.
44.2.5 Idiopathic Dilated Cardiomyopathy
Diagnostic criteria on echo for idiopathic dilated cardiomyopathy (IDC) are (1) left ventricular ejection fraction <45 % and/or fractional shortening of <25 % (both of which correspond to two standard deviations from the mean) and (2) left ventricular end-diastolic diameter of 117 %, predicted for age and body surface area (corresponds to two standard deviations +5 % from the mean) (Pelliccia et al. 1999). The features of dilated cardiomyopathy can also be clearly shown by CMR. Compared with echocardiography, CMR provides improved visualisation of the right ventricle and low amounts of variation between functional measurements. CMR can also be of assistance in determining whether the underlying cause is acquired or idiopathic. In one prospective study, McCrohon et al. (2003) showed that CMR with gadolinium delayed enhancement had a superior ability to differentiate between heart failure associated with dilated cardiomyopathy and a previous ischaemic event in comparison to coronary angiography. In that study of 90 patients labelled as having IDC based on a negative coronary angiogram, they found gadolinium-enhanced CMR demonstrated a subendocardial pattern of enhancement in 13 patients consistent with subendocardial infarction.
SPECT and PET imaging is predominantly used in the area of differentiation between ischaemic and non-ischaemic dilated cardiomyopathy rather than diagnosis of the presence of dilated cardiomyopathy itself. Studies have demonstrated that where the underlying aetiology is unclear, 99mTc-sestamibi or 13N-ammonia PET imaging can accurately differentiate between the underlying causes through perfusion scores and segmental wall motion abnormalities (Danias et al. 1998; Tio et al. 2009b). Those patients with ischaemic disease have the most severe focal regional wall motion abnormalities (Tio et al. 2009b). This compares with non-ischaemic disease where there is uniform systolic dysfunction and uniform mild reductions in perfusion (Danias et al. 1998). It is known that in patients with IDC the increased sympathetic activity owing to chronic congestive heart failure leads to an imbalance of cardiac autonomic tone, as reflected by decreased heart rate variability (HRV). 123I-MIBG, which has the same affinity for sympathetic nerve endings as norepinephrine, can be used to assess the integrity and function of the cardiac sympathetic nervous system. In the study of Lotze et al. (1999), they measured the cardiac sympathetic activity assessed by 123I-MIBG uptake compared with HRV in patients with 123I-MIBG, IDC. In 12 patients with IDC and mild to moderate heart failure, myocardial 123I-MIBG uptake was calculated from the myocardial (M) to left ventricular cavity (C). A significant correlation between the M/C ratio and mean RR interval (r = 0.52; p = 0.016) or M/C ratio and HRV triangular index (r = 0.76; p = 0.003), respectively, was found. Thus, the significant correlation between the M/C ratio and HRV indicate that they are both suitable non-invasive methods for evaluating cardiac sympathetic activity in patients with IDC. Long-term treatment with beta-blockers in IDC causes also a recovery of the cardiac adrenergic nervous system concomitantly with a clinical and haemodynamic improvement (Lotze et al. 2001).
44.2.6 Valvular Abnormalities
Aortic stenosis (AS) and mitral valve prolapse make a combined contribution of over 6 % to the cause of sudden athlete cardiac death (Maron et al. 2009).
The standard assessment of aortic stenosis is with echocardiography and Doppler analysis. Classification of the disease is according to aortic valve area with mild >1.5 cm2, moderate 1–1.5 cm2 and severe <1 cm2. Multisection CT studies have shown good correlation with echo for the evaluation of AS. A study of 30 patients demonstrated a sensitivity of 100 % and specificity of 93.7 % in identifying AS. Measurement of the mean aortic valve area by CT was 0.94 cm2 compared with 0.90 cm2 by echo using the continuity equation; this gave a correlation coefficient of 0.89 (Vanhoenacker et al. 2007).
A comparison of CMR with echo and cardiac catheterisation in the assessment of aortic valve area in a group of 40 patients found a mean aortic valve area of 0.91 cm2 by CMR compared with 0.89 cm2 by echo with a correlation coefficient of 0.96 (p < 0.0001). Valve area measurement by catheterisation was calculated using the Gorlin equation and was 0.64 cm2, giving a correlation of 0.44 which, although less favourable, was comparable to that between echo and catheterisation (0.47), leading the authors to conclude that CMR was an accurate alternative to invasive techniques and echocardiography in the assessment of aortic stenosis (John et al. 2003).
The prevalence of mitral valve prolapse has been reported at approximately 2.5 % of the population (Freed et al. 1999). It is most commonly caused by myxomatous degeneration of the valve. When leaflet thickening of greater than 5 mm occurs, it is known as “classic” mitral valve prolapse and is associated with a greater risk of sudden death (Hayek et al. 2005). Leaflet thickness less than 5 mm is known as “non-classic” (Hayek et al. 2005). The mechanism of sudden cardiac death in mitral valve prolapse is unknown and there is no evidence of increased atrial or ventricular arrhythmias on ECG monitoring (Grigioni et al. 1999). However, when mitral prolapse occurs in association with mitral regurgitation, there is an increase in ventricular arrhythmias and the risk of sudden death is increased further, especially if the associated mitral regurgitation is severe and associated with a flail leaflet (Grigioni et al. 1999).
Notably, however, more recent studies suggest that CMR may be just as valuable (Grigioni et al. 1999). However, a recent study of 25 patients found that compared with echo, CMR had a sensitivity and specificity of 100 % each in detecting leaflet incursion of greater or equal to 2 mm into the left atrium and that it also accurately identified greater leaflet thickness in those patients with MR than controls (Grigioni et al. 1999).
44.2.7 Myocarditis
Myocarditis has been found in a number of studies to be a moderate contributor to sudden athlete cardiac death in the range of 6–8 % (Corrado et al. 1998; Maron et al. 2009). Sudden death from myocarditis results from atrial or ventricular arrhythmias, complete heart block or an acute myocardial infarction-like syndrome (Cooper 2009).
Several diverse and non-specific findings have been found on echocardiography in biopsy-proven myocarditis. These include findings which are consistent with associated cardiomyopathies including hypertrophic, restrictive, dilated and ischaemic forms (Cooper 2009; Skouri et al. 2006). Wall motion abnormalities (hypokinesia, akinesia and dyskinesia) simulating acute ischaemia also occur. Echocardiography can also be used to investigate for right ventricular dysfunction, ventricular thrombi and pericardial effusions (Pinamonti et al. 1988). Progression in echocardiographic technology allowing measurement of strain rates, tissue characterisation and the detection of low-level diastolic dysfunction has also increased its role.
CMR is likely to become the non-invasive standard in the investigation of myocarditis (Magnani and Dec 2006). Different CMR sequences have the ability to identify different components of the pathophysiology of the disease. Myocardial global relative enhancement (gRE) and late gadolinium enhancement (LGE) are reflective of hyperaemia and increased capillary permeability, myocyte necrosis and increased extracellular space (Friedrich et al. 2009). T2-weighted sequences detect myocardial oedema (Fig. 44.2). Friedrich et al. (2009) pooled data from multiple studies (130 patients) and found that the most accurate methodology for diagnosing myocarditis was by using any two of gRE, LGE and T2-weighted imaging. This combined approach had sensitivities and specificities of 67 and 91 %, respectively, and a diagnostic accuracy of approximately 80 % (Friedrich et al. 2009). Anti-myosin antibodies are directed against the contractile myosin filaments within the cardiac myocyte. Cellular necrosis enables antibody binding to the protein, and detection of indium labelling of the antibody allows non-invasive assessment of myocarditis (Magnani and Dec 2006). A cohort study by Dec et al. (1990) of 111Indium-labelled anti-myosin uptake in suspected myocarditis showed a sensitivity and specificity of 83 and 53 %, respectively, and a negative predictive value of 92 %. A more recent study by Margari et al. (2003) examining anti-myosin antibody imaging in conjunction with a non-dilated left ventricle found a sensitivity and specificity of 45 and 88 %, respectively, in suspected cases of active myocarditis. Myocarditis may also be identified at (18F-FDG) PET by the presence of increased metabolic activity in the myocardium. Diffuse increased metabolic activity in the right and left ventricles may be seen at (18F-FDG) PET (James et al. 2011). This condition must be differentiated from congestive heart failure, right ventricular strain and hypertrophy due to elevated pulmonary artery pressure, which also can lead to increased (18F-FDG) uptake in the right ventricular myocardium.
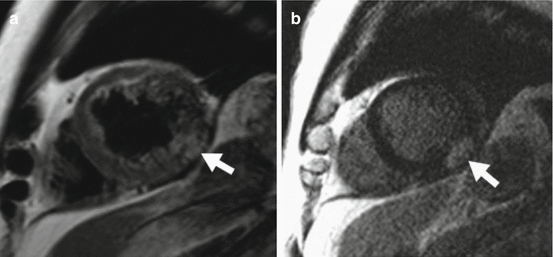
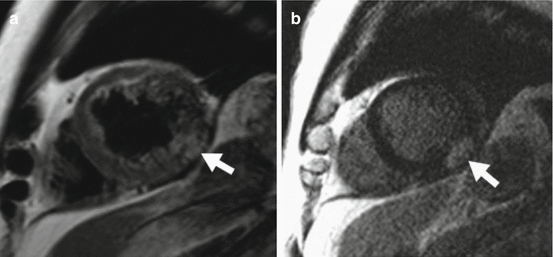
Fig. 44.2
Myocarditis. A 22-year-old man presented with central chest pain following recent viral infection. (a) T2-weighted cardiac magnetic resonance short-axis view shows epicardial and mid-wall high signal consistent with myocardial oedema. (b) Delayed, enhanced CMR image short-axis view demonstrated subepicardial and mid-wall enhancement typical of myocarditis (Friedrich et al. 2009)
44.2.8 Arrhythmias
Arrhythmogenic right ventricular cardiomyopathy (ARVC) is a substantial contributor to sudden athlete cardiac death. It is characterised by fibrofatty replacement of the myocardium, which progresses from the epicardium or mid-myocardium to become transmural (Basso et al. 2009). This leads to thinning and aneurysm of the cardiac wall classically evident in the triangle of dysplasia that involves the inferior, apical and infundibular walls (Basso et al. 1996; Thiene and Basso 2001). The left ventricle may be involved usually limited to the postero-lateral subepicardium (Basso et al. 1996; Thiene and Basso 2001). Echocardiographic examination is of great importance in the assessment of ARVC and findings can be used in the assessment of severity of disease (Nava et al. 2000). The largest study of familial ARVC by Nava et al. (2000) classified the disease as mild, moderate or severe according to right ventricular end-diastolic volume (ranging from <75 ml/m2 for mild to >120 ml/m2 for severe) and the extent of hypokinesia or akinesia (localised in mild, increasing to widespread in severe).
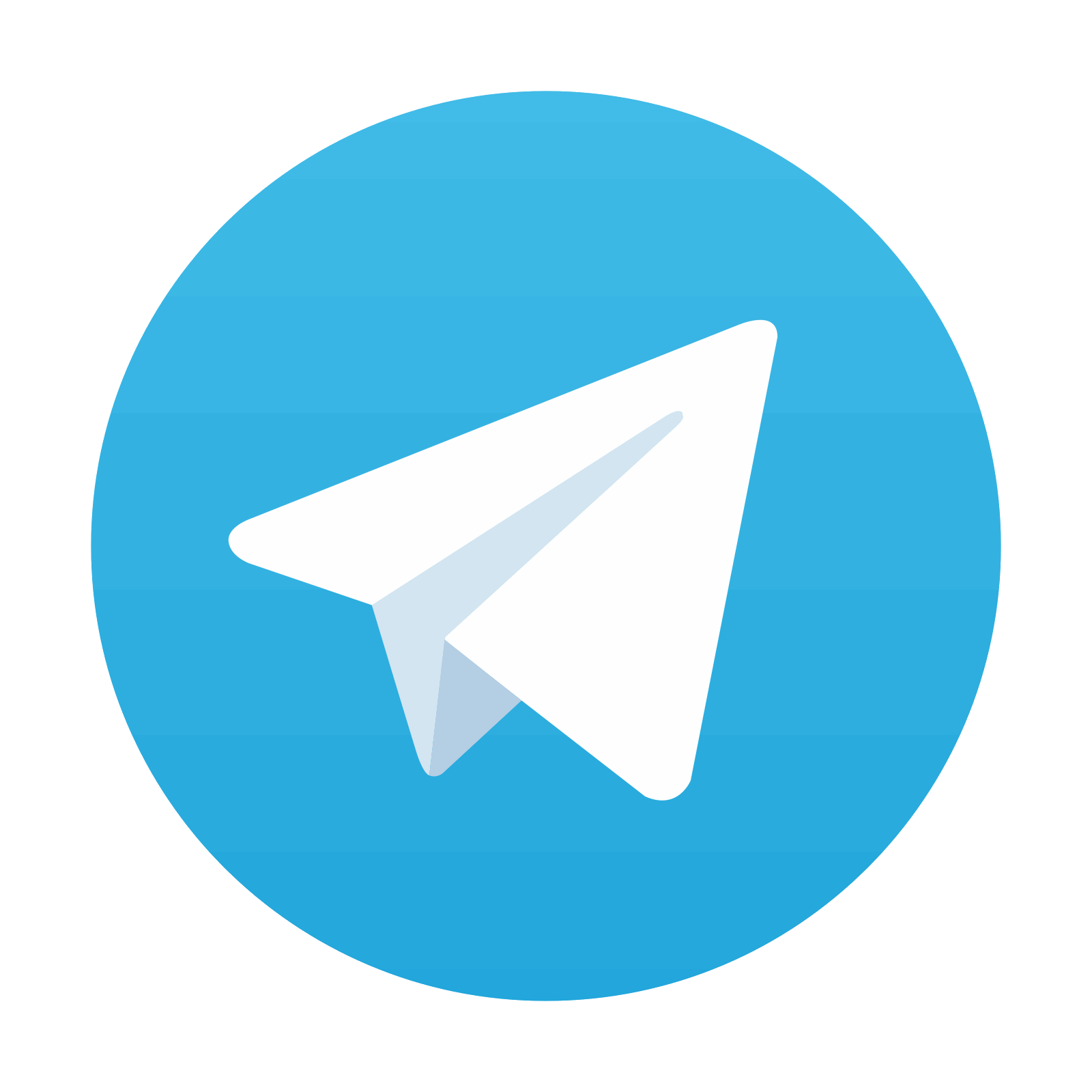
Stay updated, free articles. Join our Telegram channel

Full access? Get Clinical Tree
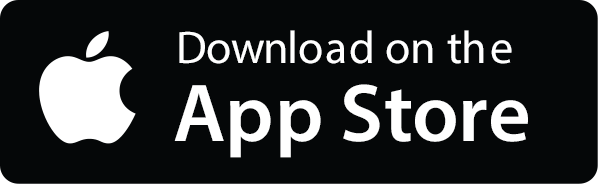
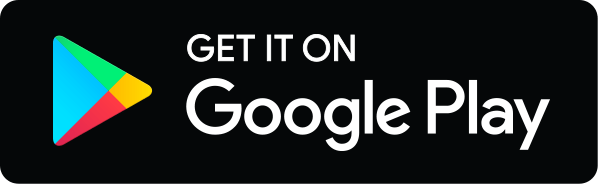