This article discusses the contribution of the pediatric cerebellum to locomotion, ocular motor control, speech articulation, cognitive function, and behavior modulation. Hypotheses on cerebellar function are discussed. Clinical features in patients with cerebellar disorders are outlined. Cerebellar abnormalities in cognitive and behavioral disorders are detailed.
Key points
- •
The pediatric cerebellum is important for processing, controlling, and modulating movement, cognition, and behavior.
- •
Pediatric cerebellar dysfunction causes poor coordination, increased variability, impaired accuracy, and tremor manifesting during limbs movements, walking, stance, talking, and eye movements.
- •
Pediatric cerebellar dysfunction results in cognitive and behavioral dysregulation.
- •
Cognitive and behavioral disorders, such as developmental dyslexia, attention deficit hyperactivity disorder, autism spectrum disorder, and schizophrenia, display cerebellar abnormalities/dysfunction.
Introduction
The cerebellum, Latin for small brain, weighs only about 10% of the adult human brain; however, it contains four times as many cerebral neurons. The cerebellum has undergone a rapid size increase in humans and apes that has been even faster than the rapid change in neocortex size. This disproportionate increase in size is unlike that seen in other anthropoid primates where the neocortex and the cerebellum underwent similar expansion rates. Such expansion underscores the relative importance of the cerebellum in humans. Nevertheless, despite more than 100 years of scientific research, the function of the cerebellum remains elusive, but there is no shortage of possible theories on how the cerebellum works.
The anatomy of the cerebellum is discussed elsewhere in this issue. For the interested reader, physiologic cerebellar anatomy has been reviewed recently. Basically, mossy fibers and climbing fibers provide excitatory inputs to the cerebellum via the superior, middle, and inferior cerebellar peduncles. Mossy fibers form synapses with granule cells, whereas climbing fibers synapse on Purkinje cells. Both fibers also send collaterals to the deep cerebellar nuclei. Granule cells form synaptic contacts with Purkinje cells via parallel fibers. Inhibitory outputs from Purkinje cells innervate the vestibular nuclei and the deep cerebellar nuclei. The latter constitutes the main output of the cerebellum and makes excitatory synapses on their targets. Other cells in the cerebellar cortex include Golgi cells, Lugaro cells, unipolar brush cells, stellate cells, and basket cells. They form interneurons within the cerebellar cortex.
Different cerebellar regions have important roles in voluntary control of limb movements, ocular motor control, balance, walking, and nonmotor higher cognitive functions. How does the cerebellum provide these functions?
The answer to this question has been addressed indirectly based on studies in patients with cerebellar damage. Computational modeling and experimental animal studies using pharmacologic, lesion, and genetic manipulations have also contributed further important insights. More recently, cerebellar transcranial direct current stimulation has provided a noninvasive approach to investigating cerebellar functions in health and disease.
Movement abnormalities resulting from cerebellar impairment include poor coordination, increased variability, impaired accuracy, and tremor manifesting during limbs movements, walking, stance, talking, and eye movements.
Two popular proposals on how the cerebellum functions are actively debated. The first proposal states that the cerebellum contributes to motor and nonmotor control by acting as a timer. The second proposal states that the cerebellum functions by updating and/or storing an internal model of body dynamics. In this model, the cerebellum essentially predicts the consequences of motor command on the body and its surroundings (eg, how a motor command will change the state of a limb or object position and velocity). A feedforward (ie, predictive and planned in advance) signal provides a fast response instead of relying on a visual or peripheral sensory feedback system with its attending long delay to ensure a real-time, correct, and appropriate motor response. In addition, the cerebellum improves proprioception (ie, sensing the position of a limb in space in the absence of visual input) during active but not passive limb movement, through prediction.
Other theories on cerebellar function include temporal and spatial sequence detection within the feedforward control mechanism, tonic facilitation providing fine tuning of downstream target structures, and the initiation of coordinated compound movements.
Introduction
The cerebellum, Latin for small brain, weighs only about 10% of the adult human brain; however, it contains four times as many cerebral neurons. The cerebellum has undergone a rapid size increase in humans and apes that has been even faster than the rapid change in neocortex size. This disproportionate increase in size is unlike that seen in other anthropoid primates where the neocortex and the cerebellum underwent similar expansion rates. Such expansion underscores the relative importance of the cerebellum in humans. Nevertheless, despite more than 100 years of scientific research, the function of the cerebellum remains elusive, but there is no shortage of possible theories on how the cerebellum works.
The anatomy of the cerebellum is discussed elsewhere in this issue. For the interested reader, physiologic cerebellar anatomy has been reviewed recently. Basically, mossy fibers and climbing fibers provide excitatory inputs to the cerebellum via the superior, middle, and inferior cerebellar peduncles. Mossy fibers form synapses with granule cells, whereas climbing fibers synapse on Purkinje cells. Both fibers also send collaterals to the deep cerebellar nuclei. Granule cells form synaptic contacts with Purkinje cells via parallel fibers. Inhibitory outputs from Purkinje cells innervate the vestibular nuclei and the deep cerebellar nuclei. The latter constitutes the main output of the cerebellum and makes excitatory synapses on their targets. Other cells in the cerebellar cortex include Golgi cells, Lugaro cells, unipolar brush cells, stellate cells, and basket cells. They form interneurons within the cerebellar cortex.
Different cerebellar regions have important roles in voluntary control of limb movements, ocular motor control, balance, walking, and nonmotor higher cognitive functions. How does the cerebellum provide these functions?
The answer to this question has been addressed indirectly based on studies in patients with cerebellar damage. Computational modeling and experimental animal studies using pharmacologic, lesion, and genetic manipulations have also contributed further important insights. More recently, cerebellar transcranial direct current stimulation has provided a noninvasive approach to investigating cerebellar functions in health and disease.
Movement abnormalities resulting from cerebellar impairment include poor coordination, increased variability, impaired accuracy, and tremor manifesting during limbs movements, walking, stance, talking, and eye movements.
Two popular proposals on how the cerebellum functions are actively debated. The first proposal states that the cerebellum contributes to motor and nonmotor control by acting as a timer. The second proposal states that the cerebellum functions by updating and/or storing an internal model of body dynamics. In this model, the cerebellum essentially predicts the consequences of motor command on the body and its surroundings (eg, how a motor command will change the state of a limb or object position and velocity). A feedforward (ie, predictive and planned in advance) signal provides a fast response instead of relying on a visual or peripheral sensory feedback system with its attending long delay to ensure a real-time, correct, and appropriate motor response. In addition, the cerebellum improves proprioception (ie, sensing the position of a limb in space in the absence of visual input) during active but not passive limb movement, through prediction.
Other theories on cerebellar function include temporal and spatial sequence detection within the feedforward control mechanism, tonic facilitation providing fine tuning of downstream target structures, and the initiation of coordinated compound movements.
Limbs motor control
Parallel fibers link Purkinje cells and deep cerebellar nuclei, where single muscles are represented, thus providing a way of linking movements involving many muscles (ie, complex sequence of movements). It is not known what signal the cerebellum uses to exert its modulatory control on movements. Candidate signals that may be used by the cerebellum include sensory information from the periphery or copies of the movement commands (efference copy) from the primary motor cortex. Another possibility that has not been proven is that the cerebellum is capable of generating motor commands that could lead a limb toward a desired target.
Smooth and accurate execution of voluntary movements and adaptation to changing motor tasks depend on a healthy cerebellum. Through trial and error, the cerebellum can learn and store different combinations needed for precise compound movements. Motor learning in children is not similar to adults. Prior experience but not error size improves motor learning in young children. Various types of motor learning are achieved at different ages. In children up to 11 years of age, spatial adaptation matured at a slower rate than temporal adaptation in locomotion tasks that demanded walking on a split-belt treadmill with each leg’s speed controlled independently. Temporal adaptation (learning a new timing change) of a locomotor task matures by the age of 3 years.
Patients with cerebellar lesions can perform simple motor tasks, with incoordination and impaired initiation of movement appearing when compound complex movements are performed, especially at a rapid pace. Cerebellar impairment cause greater dysfunction in predictive movements than in movements that require feedback (ie, visual or somatosensory feedback information). However, the mechanism uses peripheral feedback functions suboptimally as the demand on it increases. Patients with cerebellar disorders have been shown to have proprioceptive deficits during active but not passive limb movements. Cerebellar dysfunction affects fast movements to a greater extent than slow movements. In addition, the ability to adapt to novel changes in movements is impaired. Imprecise movements and errors in perception during active predictable movements following cerebellar impairment have been attributed to a malfunction in the internal models of body dynamics. Table 1 shows a list of signs seen in patients with cerebellar impairment.
Sign | Comment |
---|---|
Dysmetria | Inaccurate movement trajectory with undershooting or overshooting a target. It can be observed during finger-nose-finger examination |
Intention (kinetic) tremor | Oscillation of a limb especially when approaching a target during voluntary movements |
Dysdiadochokinesia | Irregular and slow movements observed during rapid alternating movements of the hand involving successive pronation and supination |
Action tremor | Oscillations observed during postural tasks, for example, maintaining the heel of one foot over the contralateral knee for a few seconds |
Essential tremor | Oscillations of a body part, for example, the head or outstretched arms during a maintained posture |
Palatal tremor | Rhythmic oscillations of the palate |
Dysrhythmokinesia | Abnormal rhythm observed during tapping of a limb |
Hypotonia | Decreased resistance to passive stretch |
Motor delay | Slow acquisition of motor milestones |
Rebound | Abnormally large displacement of an outstretched arm following a tap on the wrist with overshooting followed by few oscillations around the primary position |
Ataxia of stance | Swaying of the body while standing up |
Ataxia of gait | Wide-based gait with staggering and swaying |
Head tilt | Lateral displacement of the head |
Titubation | Involuntary rhythmic oscillations of a body part, for example, head or trunk |
Pendular reflexes | Excessive oscillations of a limb (like the swing of a pendulum) observed after eliciting a deep tendon jerk |
Ocular motor control
The cerebellum is important for all types of eye movements and for ensuring fixation stability. The vestibulocerebellum is essential for gaze stability, vestibulo-ocular reflex, and smooth ocular pursuit. Various types of nystagmus, for example, gaze-evoked nystagmus and saccadic intrusions, which are abnormal fast eye movements that take the fovea off the target, occur following cerebellar damage and result in fixation instability. The oculomotor cerebellum, which includes the fastigial nuclei and dorsal vermis lobules VI and VII, is mostly important for saccade processing; however, it also participates in smooth ocular pursuit initiation, horizontal alignment of the 2 eyes, and vergence processing.
The cerebellum fine-tunes eye movements and reduces their baseline variability to ensure that the 2 eyes are stable and working together. These actions are important for bringing and maintaining objects of interest on the fovea, which in turn leads to the best visual acuity whether the person is moving or not. This fine-tuning occurs online and also over time by a process called adaptation.
Adaptation ensures the best calibration of the ocular motor responses, for example, the amplitude and direction of the vestibulo-ocular reflex, the velocity of smooth ocular pursuit relative to target velocity. In addition, the amplitude of saccades can undergo adaptation (ie, change in size), for which the cerebellum provides an essential role. Table 2 shows a list of ocular motor signs seen in patients with cerebellar diseases.
Sign | Comment |
---|---|
Gaze-evoked nystagmus | Ocular oscillations observed while trying to hold gaze eccentrically (ie, off center), horizontally, and/or vertically. The fast phase of the nystagmus is toward the direction of gaze |
Downbeat nystagmus | Ocular oscillations observed with the eyes in central position (ie, the eyes are located in the primary mid orbital position). The fast component beats downward. The nystagmus is exacerbated in downgaze and lateral gaze |
Upbeat nystagmus | Ocular oscillations observed with the eyes in central position. The fast component beats upward. The nystagmus is exacerbated in upgaze |
Rebound nystagmus | Transient ocular oscillations observed with the eyes in central position after returning from a maintained eccentric gaze |
Periodic alternating nystagmus | Horizontal ocular oscillations observed with the eyes in central position that change direction gradually after a silent phase. It occurs in a periodic manner, usually every 1–2 min |
Opsoclonus | Conjugate, random, involuntary, and multidirectional back-to-back fast eye movements observed during attempted fixation or movement of the eyes |
Ocular flutter | Conjugate, random, involuntary, and horizontal back-to- back fast eye movements observed during attempted fixation or movement of the eyes |
Ocular bobbing | Fast downward displacement of the eyes followed by slow return back to the central orbital position |
Square wave jerks/macrosaccadic oscillations | Fast, intruding, unwanted, involuntary, and conjugate eyes movements, which take the eyes off fixation. They may occur repetitively |
Saccadic dysmetria | Inaccurate fast eye movement that either undershoot (hypometria) or overshoot (hypermetria) a visual target |
Saccade initiation delay (ocular motor apraxia) | Increased latency of fast eye movements that can usually be overcome with a head thrust or a blink |
Slowing of smooth pursuit velocity (especially initiation) | Jerky (instead of smooth) eye movements that are observed during visual tracking |
Impaired vestibulo-ocular reflex cancellation | The ability to fixate objects moving in the same direction of the head requires cancellation of the vestibulo-ocular reflex, which normally drives the eyes contralateral to the direction of the head movement. Patients with cerebellar disease may not be able to cancel the vestibulo-ocular reflex |
Abnormal optokinetic nystagmus | Fast ocular oscillations (jerk nystagmus) are normally observed while tracking a rotating drum with alternating white and black stripes. The nystagmus generated with such a stimulus may be exaggerated with chronic cerebellar disease or dampened with acute cerebellar lesions |
Impaired adaptation of eye movements | Motor learning (adaptation) of the ocular motor system usually occurs physiologically or following disease to repair and improve the accuracy or velocity of eye movements. Adaptation may be impaired in cerebellar disease. |
Skew deviation | Vertical misalignment of the eyes (ie, one eye is higher than the fellow eye), which changes as a function of horizontal gaze position |
Abnormalities in the control of torsion | Abnormal rotational control of the eye around an axis perpendicular to the center of the pupil |
Speech control
Spoken language broadly consists of 2 different neurologic functions with different anatomic correlates. Language is discussed in a separate section of this article. Here, the production of speech is focused on, which is a complex process that involves several networks located in the cerebrum and cerebellum. The cerebellar contribution to speech control is likely similar to its control of limb movements. The production of speech involves the coordination of a large number of muscles, in particular the tongue and orofacial muscles. Specifically, the cerebellum plays an important role in speech articulation, prosody (ie, characteristics of speech style including rhythm, speed, emphasis, and pitch), and planning and processing of speech and language. Inputs from premotor, auditory, and somatosensory areas to the cerebellum provide important information for choosing motor commands for speech.
Cerebellar impairment can cause ataxic dysarthria. Box 1 shows the characteristics of speech abnormalities in patients with cerebellar disorders. Abnormalities in speech motor programming through impaired timing and deficits in speech execution are implicated in ataxic dysarthria. It is hypothesized that processing abnormalities in the feedforward motor commands result in abnormal speech production because it becomes more reliant on sensory feedback with its attendant delays. Such a delay may slow speech, produce pauses, and disrupt speech rhythm.
Scanning speech (eg, hesitation, accentuation of some syllables, omission of appropriate pauses, addition of inappropriate pauses)
Explosive speech
Slowness of speech
Syllables or words are not understandable with lack in speech clarity
Slurring of speech
Loss of intonation (abnormal rhythm and emphasis)
Voice tremor
There is some evidence for specific sites within the cerebellum that are important for speech articulation. Increased signal intensity on functional MR imaging over the medial parts of the anterior lobe of the cerebellum has been described in association with tongue and lip movements in healthy volunteers. Lesions caused by stroke involving the superior paravermal area of the right cerebellar hemisphere may lead to dysarthria.
A role beyond motor systems
With the transition from the nineteenth to the twentieth century, work from such distinguished neurologists as Babinski and Holmes postulated exclusive roles for the cerebellum in motor control with isolated cerebellar lesions resulting in deficits of motor coordination, motor speech output, and ocular motor function. Over the next three-quarters of a century, as cerebellar circuitry was further elucidated and theories postulated regarding its mechanisms of actions, few studies challenged this view of a motor cerebellum.
The first challenge to this idea of a strictly motor cerebellum was postulated by Leiner and colleagues, after examination of the development of the dentate nucleus during evolution. From more primitive species to primates, dentate nuclear development occurred most dramatically in the ventral dentate, an expansion mirrored by growth in the prefrontal cortex. By comparing dentate-thalamic and thalamic-cortical projections, they postulated that these newly expanded areas of the dentate were connected to nonmotor areas of the cortex and thus were likely to have roles outside of strict motor constructs.
These hypotheses were supported over the next decade by the work of Schmahmann and Pandya and Middleton and Strick. These 2 groups used new developments in anterograde and retrograde tracing methodologies to define afferent and efferent circuits connecting the cerebellum to the cortex. Although these studies detailed connections to cortical motor areas as expected, these studies also identified extensive connections to nonmotor cortical areas.
These tracing studies have since been supported by imaging studies examining both anatomic and functional connectivity of the human cerebellum. From functional MR imaging and resting state connectivity studies, extensive connections between nonmotor cortex and cerebellum have been established. In fact, these studies have remarkably demonstrated that most of cerebellum appears to be connected, not to motor, but to nonmotor cortical areas.
In addition, cerebellar abnormalities and dysfunction have been identified in cognitive and neuropsychiatric disorders. Moreover, clinical evidence, functional imaging, and targeted preclinical studies further support nonmotor roles for the cerebellum in multiple areas of cognition and behavior, which are detailed in later discussion with a specific emphasis on the pediatric population.
Language
Roles for the cerebellum in speech initially revolved around motor control of speech. However, a role for the cerebellum in nonmotor aspects of language has emerged. Initial functional imaging studies demonstrated activation of the cerebellum, predominantly in lateral cerebellum on the opposite side to cortical language domains, in tasks of verbal fluency. Moreover, cerebellar lesions have been demonstrated to result in verbal fluency deficits. The cerebellum has also been implicated in production and comprehension of syntax, prosody, and grammar, with cerebellar dysfunction resulting in disturbances in these domains. Furthermore, clinical case studies have produced a picture of a cerebellar aphasia with difficulties in syntax, anomia, perseveration, reduced speech output, and speed. Although these deficits again correlate mostly with cerebellar lesions localized to the opposite side of the dominant language cortex, evidence has emerged for a more complicated role and involvement for both lateral cerebellar hemispheres.
Most of these studies, however, have involved adult patients. In the pediatric population, a role for the cerebellum in language has been postulated since the initial description by Daly and Love, of posterior fossa syndrome, a syndrome characterized by mutism, behavioral, and affective disturbance, and executive dysfunction in children after posterior fossa surgery. Although the mutism often improves, children frequently continue to have persistent language dysfunction. This syndrome has since been shown to involve disruption of afferent and efferent tracts of the cerebellum. Similar findings have been identified in patients with acute disseminated encephalomyelitis involving the cerebellum, while residual language disturbance has also been identified in pediatric multiple sclerosis in patients with cerebellar lesions. Similar to adults, in pediatric patients with epilepsy, language appears to localize mostly to the cerebellar hemisphere contralateral to cortical language areas.
Cerebellar language involvement has also been implicated in reading. The cerebellum has been implicated in brain networks critical for reading on functional MR imaging. Furthermore, abnormalities in structural cerebellar (volumetric and diffusion) imaging have been found in individuals with developmental dyslexia with functional MR imaging demonstrating significant differences between children with dyslexia and age matched controls. In addition, motor deficits have been identified in individuals with dyslexia, prompting the generation of a cerebellar developmental dyslexia hypothesis. Further supporting a role for the cerebellum in reading, individuals with cerebellar disorders demonstrate significant reading impairments.
Cognition
As with language, studies investigating cortical-cerebellar connections support roles for the cerebellum in cognitive processes. Functional imaging has revealed cerebellar activation during numerous cognitive tasks. One of the more consistent processes associated with cerebellar activation involves tasks related to working memory or executive function. Cerebellar activation has also been identified with tasks of attention and timing. As with the cerebellar role in language, studies on a cerebellar contribution to cognition largely support a role for the lateral cerebellar hemispheres in supporting cognitive processes. Clinical and preclinical studies have corroborated these findings from imaging studies. Preclinical models and individuals with cerebellar lesions display diverse cognitive deficits: executive function deficits; deficiencies in procedural memory, declarative memory, and associative memory such as eye blink conditioning; and deficits in timing/attention.
In the pediatric population, a similar picture emerges with significant cognitive disruption associated with pediatric cerebellar disruptions ranging from cerebellar developmental abnormalities to inflammatory insults, ischemic injury, and oncologic and postsurgical injury. These cognitive deficits involve disruptions in executive dysfunction, working memory, procedural memory, and processing abilities in addition to impacting intellectual quotient and visuospatial abilities.
Behavior
In addition to cognitive and language processes, the cerebellum has been proposed to play roles in regulating behavior. Schmahmann and Sherman, in their initial description of cerebellar cognitive affective syndrome described significant behavioral disruption in 20 patients with cerebellar disruption with behaviors ranging from affective changes to disinhibited behaviors. In fact, Daly and Love, in their initial description of the posterior fossa syndrome in 1958, described a similar dysfunction with loss of affective responses, marked apathy, social disinterest, and emotional blunting. Subsequent studies of cerebellar lesions have supported these initial descriptions with numerous associated behavioral disruptions, including affective disruption, alterations in attention, anxious behaviors, emotional and social blunting, and obsessive and compulsive behaviors. Conversely, examination of behavioral disorders has further supported a contributory role for the cerebellum in behavioral regulation with significant cerebellar abnormalities identified in several neurodevelopmental and behavioral disorders. Here, cerebellar involvement in 3 such disorders: attention deficit hyperactivity disorder (ADHD), schizophrenia, and autism spectrum disorders (ASD), are highlighted.
Attention Deficit Hyperactivity Disorder
ADHD is estimated to affect 5% of the pediatric population, with estimates approaching 50% of these individuals having persistent symptoms into adulthood. Cerebellar lesions as noted previously are often accompanied by impaired attention and disinhibited behavior.
As in developmental dyslexia, children with ADHD manifest high rates of motor abnormalities of up to 50% with motor deficits correlating with worse outcomes. Multiple studies have reported decreased size of the cerebellum in patients with ADHD, with many studies demonstrating decreases in the posterior cerebellum (lobules VIII–X). Reductions in the posterior cerebellar volumes themselves correlated with severity of illness. Longitudinal studies of ADHD demonstrated persistent reductions in cerebellar size, whereas initial changes in the caudate resolved with time. Compared with typically developing siblings, individuals with ADHD demonstrated reductions in right cerebellar volumes, whereas prefrontal regions showed changes in both individuals with ADHD and their typical developing siblings. White matter abnormalities have also been identified in ADHD with involvement of bilateral middle cerebellar peduncles, whereas functional connectivity is also significantly disrupted. As further evidence of cerebellar involvement, changes in cerebellar activation was visualized after just a single dose of the stimulant methylphenidate, one of the mainstays of ADHD therapy, whereas cerebellar volumes in patients receiving methylphenidate therapy were significantly larger than volumes in untreated cohorts.
Schizophrenia
Schizophrenia is a devastating psychiatric disorder characterized by a constellation of symptoms including hallucinations, delusions, impaired judgment, disorganized speech and behavior, motor disruptions, and negative symptoms (decrease/absence of thoughts, actions, affect). The disorder is associated with impairment in cognitive domains, including memory, learning, and executive function.
The cerebellum has been implicated in the pathogenesis of schizophrenia. Cerebellar lesions result in psychiatric disturbances applicable to schizophrenia, including both positive and negative symptoms. As with ADHD and developmental dyslexia, schizophrenia is also accompanied by elevated rates of motor abnormalities. Studies have revealed numerous structural changes in the cerebellum of patients with schizophrenia, including reduced posterior vermis size, reductions in Purkinje cell size, and abnormal white matter connectivity, whereas reduction in cerebellar size correlates with symptom severity. Functional studies also have revealed disruptions in corticocerebellar connections, with alterations in cerebellar-cortical activity during performance of many cognitive tasks, including memory, executive function, and working memory, with other studies demonstrating abnormalities in cerebellar activity with theory of mind tasks. Intriguingly, treatment of a small cohort of treatment-resistant patients with cerebellar vermal theta burst stimulation demonstrated significant improvement in mood and negative symptoms in addition to improvements in cognitive measures. The exact mechanism underlying these benefits is not understood, although modulation of cortico-cerebellar loops has been postulated.
Although onset of the schizophrenia occurs mostly in adolescence or early adulthood, less commonly, symptoms will emerge in childhood. Childhood-onset schizophrenia tends to portend a more severe diagnosis and is often accompanied by more severe premorbid symptoms, such as language, social, and motor disturbance. In this pediatric population, studies have documented reductions in cerebellar volume as seen in adult-onset schizophrenia. Longitudinal studies demonstrated that volumes were initially comparable to controls with volume decreases noted only on subsequent studies. Disruptions in functional connectivity have also been identified in the cerebellum during a verbal working memory task in childhood-onset schizophrenia.
Autism Spectrum Disorders
ASDs are prevalent neurodevelopmental disorders (affecting 1 in 68 children in the United States from latest estimates) that are characterized by social dysfunction and repetitive behaviors/restricted interests. Numerous studies have implicated the cerebellum in the pathogenesis of the disorder. As documented earlier, cerebellar activation is demonstrated in social paradigms. As with the above disorders, motor dysfunction is prominent in ASDs with prevalent motor apraxias, alterations in tone, and abnormalities in eye movements affecting most patients (reviewed recently by Mosconi and colleagues ).
Pathologic findings have also implicated the cerebellum in these disorders. In fact, the most consistent pathologic feature identified in postmortem tissue from individuals with ASD is cerebellar Purkinje cell loss and/or reductions in Purkinje cell size. ASD pathologic specimens also reveal evidence of increased oxidative stress in the cerebellum. Abnormal gene networks associated with ASD have also been identified in the cerebellum in animal models.
Structural changes have also been demonstrated in ASD with initial studies focused on alterations in vermis volume. Subsequent studies have been able to narrow that focus to more specific areas, most localized to the posterior cerebellum. Abnormalities in structural connectivity have also been identified in patients with ASD, while studies have also revealed abnormalities in functional connectivity. In addition, studies demonstrate reduced function in the cerebellum in patients with ASD.
Supporting a critical role for the cerebellum in ASDs, isolated cerebellar injury also significantly increases the risk of developing ASD symptoms. Limperopoulos and colleagues demonstrated that approximately 37% of children with isolated cerebellar hemorrhage demonstrated autistic behaviors. When location of hemorrhage was considered, involvement of cerebellar vermis resulted in an increased rate ranging from 80% to 100%. Furthermore, in children with developmental malformations of the cerebellum, rates of social dysfunction and positive autism screening are elevated and associated with vermian involvement. Children with posterior fossa syndrome also demonstrate increased rates of autistic behavior.
Preclinical models similarly suggest cerebellar dysfunction in ASDs. Cerebellar abnormalities have been identified in multiple rodent models of ASD. The cerebellar-mediated associative learning paradigm, eye blink conditioning, was also found to be abnormal in multiple ASD rodent models. Moreover, in mouse models of tuberous sclerosis complex, a neurodevelopmental disorder with high rates of ASD, genetic disruption limited to cerebellar Purkinje cells resulted in these mice displaying autistic-like behaviors with social impairments, repetitive behaviors, and behavioral inflexibility. These models also displayed abnormality consistent with clinical abnormality with ASD loss, increased markers of oxidative stress, and abnormalities in dendritic spines, while also displaying on electrophysiology studies, impaired Purkinje cell function and excitability, consistent with functional imaging in ASD. Thus, whereas previous studies were unable to address whether cerebellar dysfunction can result in ASD behaviors or whether cerebellar abnormalities are secondary to other distal processes, these studies demonstrated that cerebellar dysfunction by itself was sufficient to generate abnormal behaviors seen in patients with ASD.
Other Disorders
In addition, the cerebellum has been implicated in a diverse array of additional behavioral disorders, including bipolar disorder, depression, and anxiety (reviewed by Phillips and colleagues ).
However, for the purposes of this review, the authors have tried to focus on those disorders that affect the pediatric population and for which sufficient data generated from children were available.
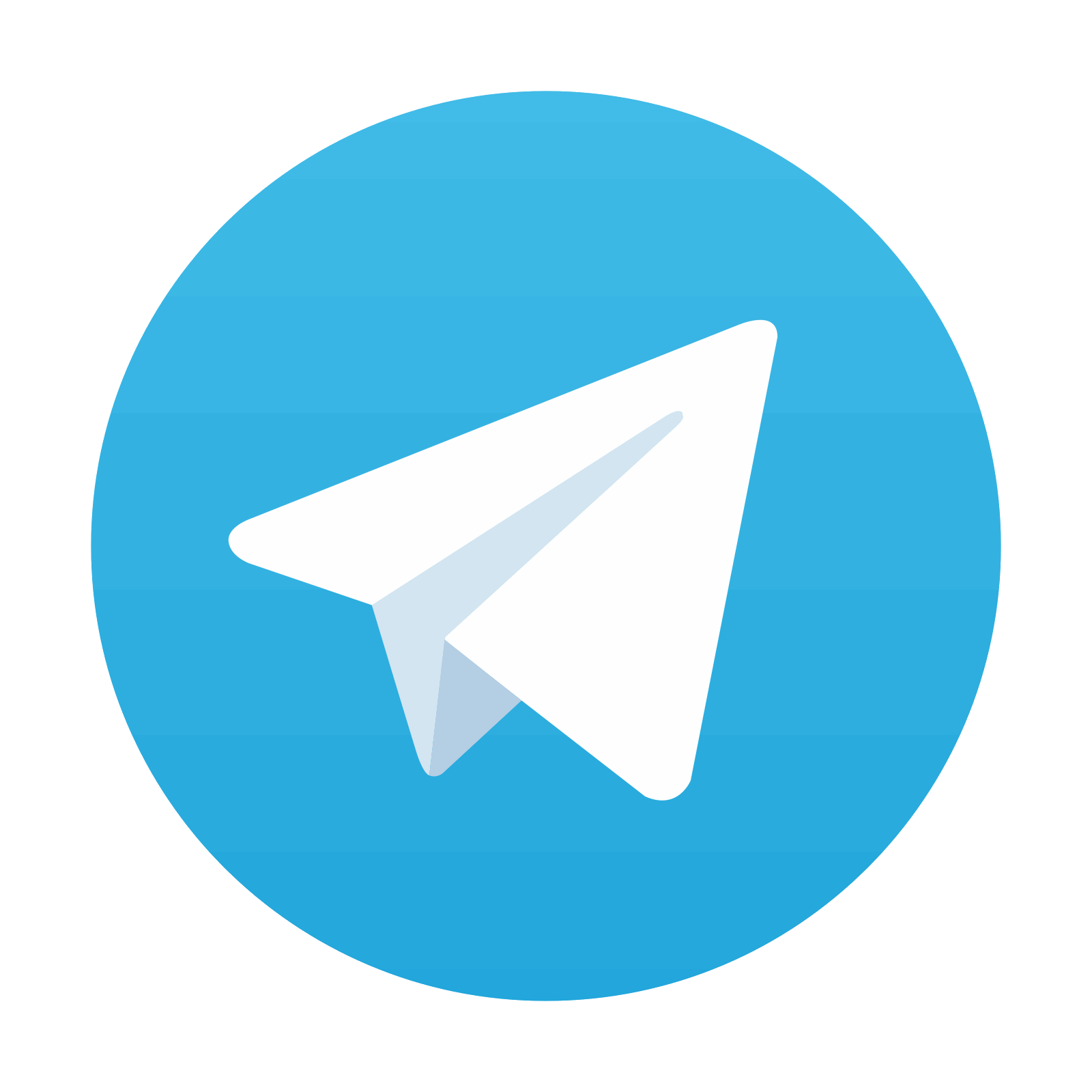
Stay updated, free articles. Join our Telegram channel

Full access? Get Clinical Tree
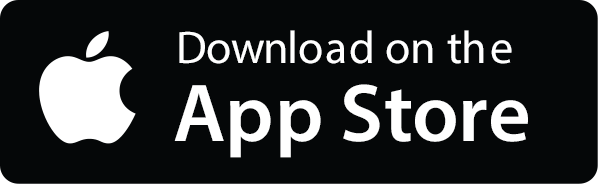
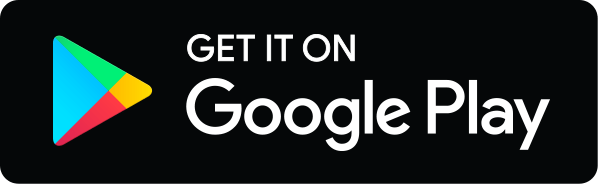