5 The Role of Ultrasound in the Management of Cerebrovascular Disease
Transcranial Doppler (TCD) in particular provides a wealth of information, including real-time bedside assessment of pathophysiologic changes in patients with cerebrovascular disease, including monitoring of thrombus dissolution or reocclusion, collateral development, and cerebral embolization, as well as the progress of therapies.1–15
Cerebrovascular ultrasound can be used for rapid detection and quantification of the severity of arterial occlusive disease, thus facilitating patient selection for reperfusion therapies, invasive angiography, and urgent interventional treatment, and for assessment of the short- and long-term prognosis. No other imaging method in wide use today offers the same potential for continuous real-time monitoring of arterial blood flow. In addition, ultrasound waves can have a therapeutic effect through the transmission of mechanical energy directly to the soft tissues. The therapeutic application of ultrasound has been shown to augment residual blood flow and to speed up thrombolysis, allowing acute stroke patients to recover more rapidly.16–17
Prerequisites to a successful practice of cerebrovascular ultrasound include knowledge of anatomy, physiology of cardiovascular and nervous systems, fluid dynamics, and pathologic changes in a variety of cerebrovascular disorders, as well as basics of ultrasound physics and instrumentation. The accuracy of the practice of ultrasound (both performance and interpretation) varies between practitioners of different skill, knowledge, and experience. Besides credentialing, constant learning and improvement through consistent application, local validation of ultrasound testing and interpretation, and continuing quality improvement are the keys to successful practices.18
It is also desirable that each neurovascular ultrasound laboratory validates its own diagnostic criteria in order to reduce variability and improve consistency of ultrasound interpretation. This requirement in the United States is endorsed by the Intersocietal Commission for Accreditation of Vascular Laboratories (ICAVL)19 (www.icavl.org).
• Differentiate normal from diseased vessels and quantify the degree of the arterial stenosis and plaque burden
• Identify the disease process, including acute occlusions or dissections in the major extracranial and intracranial vessels, including lesions amenable to interventional treatment (LAITs)
• Identify collateral flow pattern and flow direction for assessing the ability of collateral circulation to maintain cerebral blood flow
• Detect, localize, and quantify cerebral embolism, particularly in the context of intraoperative monitoring of carotid revascularization procedures
• Detect and grade right-to-left shunts
• Assess recanalization and reocclusion with thrombolysis
• Monitor and even augment thrombolysis
• Predict stroke risk in sickle cell disease (SCD)
• Identify subclavian steal syndrome
• Evaluate cerebrovascular autoregulation or vasomotor reactivity
• Identify intracranial steal and reversed Robin Hood syndrome
• Detect and monitor arterial spasm
• Indirectly detect excessive intracranial pressure and assess cerebral circulatory arrest
Finally, ICAVL recommends consistent use of and adherence to standardized scanning protocols. This helps identify common sources of error in the accuracy of ultrasound testing compared to other modalities and eliminate systematic sources of error through quality improvement.19 Published examples of extracranial carotid, vertebral, and intracranial ultrasound protocols are available.
Carotid Atherosclerotic Disease: Clinical Implications of Early Detection
Up to 15% of cerebral infarctions are associated with embolic debris and thrombi originating from atherosclerotic plaques at the carotid bifurcation.20 The risk for stroke in patients with carotid atherosclerosis is closely associated with the severity of luminal stenosis. For asymptomatic patients with less than 75% stenosis, the yearly risk for stroke is less than 1%, but this risk increases to 2% to 5% for patients with stenosis greater than 75%.21,22 The risk is much higher in symptomatic patients (i.e., those who have had previous transient ischemic attacks or strokes) at 10% in the first year for those with severe lesions, with risk rising to 30% to 35% over the next 5 years.23
On the other hand, carotid atherosclerotic disease is one of the major potentially preventable causes of stroke. Three pivotal studies (the North American Symptomatic Carotid Endarterectomy Trial [NASCET], the European Carotid Surgery Trial [ECST], and the Veteran Affairs Cooperative Studies Program Trial) have clearly shown the benefits of carotid endarterectomy (CEA)compared with medical treatment in recently symptomatic patients with severe carotid stenosis.24–26 The pooled analysis of these three trials showed significant benefits of surgery in the group with severe stenosis (70%-99% stenosis in NASCET; absolute risk reduction of 16%, P <.001) after 5 years of follow-up.27 In patients with mild stenosis (50% or less in NASCET), the risks incurred during CEA outweighed the benefits of surgery.27 Patients with moderate stenosis (50%-69% in NASCET) still benefitted from surgery, although the overall gains were more modest than in patients with severe stenosis, with an absolute risk reduction of 4.6% after 5 years.27 In asymptomatic carotid artery disease, the efficacy of CEA is also poorly defined. Results from the Asymptomatic Carotid Atherosclerosis Study (ACAS) showed a 47% relative reduction in the risk for ipsilateral stroke and perioperative death in patients randomized to surgery despite a 5-year risk for ipsilateral stroke without the operation of only 11%.28 The UK Medical Research Council Asymptomatic Carotid Surgery Trial (ACST) Collaborative Group confirmed a modest benefit of CEA in asymptomatic patients who were younger than 75 years and who had at least 70% carotid stenosis on ultrasound: immediate CEA halved the net 5-year stroke risk from about 12% to about 6%.29 There is a growing body of evidence from observational and epidemiologic studies that the risk for subsequent stroke in patients with carotid stenosis is highest in the first few weeks after onset of transient ischemic attacks (TIAs) or minor strokes, and this risk declines rapidly thereafter.30–32.This finding implies a short time window for effective stroke prevention, necessitating the rapid identification of patients with substantial carotid stenosis and the swift initiation of medical treatment or revascularization procedures.
Furthermore, during the last decade, several clinical trials have tested and compared the efficacy and safety of different carotid revascularization procedures. Carotid stenting has emerged as a safe alternative to CEA, leading to safely treating patients with carotid artery disease who have contraindications to surgery or when surgery is not a suitable rescue. One of the largest randomized stroke prevention trials ever, the Carotid Revascularization Endarterectomy vs Stenting Trial (CREST) took place at 117 centers in the United States and Canada over a 9-year period. The overall safety and efficacy of the two procedures was largely the same with equal benefits for both men and for women, and for patients who had previously had a stroke and for those who had not.33 It appears that CEA could be more beneficial in older patients, whereas stenting is associated with higher likelihood of periprocedural strokes. These findings should be interpreted with caution. First, confidence intervals on procedure benefit vs age were wide, and secondly the definitions of myocardial infarction (more common after CEA) and stroke were not the same in terms of clinical significance and severity. Overall, both procedures offered similar secondary stroke recurrence rates and remained viable options for stroke prevention.33
The mainstay of carotid imaging is, therefore, to enable accurate prediction of the severity of stenosis expressed as clinically relevant ranges of the NASCET study34 and to facilitate delivery of treatment within a short time window.30
Randomized trials24,25,28 used DSA as the diagnostic test to measure the degree of carotid stenosis expressed as the percentage linear diameter reduction of the internal carotid artery (ICA) determined by specific methods. To apply these methods, only one view of the narrowest residual lumen (d) should be selected and the measurement sites (n) should be chosen differently for each method (Figure 5-1). The stenosis is calculated using the formula
where d and n are the diameter measurements made in mm.
The European (E) method, or the “local” degree of stenosis, requires drawing an imaginary outline of the ICA bulb to estimate the normal dimensions of the vessel at the site of the tightest narrowing. Although there is no objective way to decide where exactly the normal vessel wall is supposed to be on the DSA image, the E method has a good reproducibility between the experienced observers and provides stenosis values closer to anatomic stenosis than the N method. For instance, a 70% N stenosis is equal to 84% E stenosis and 90% area stenosis. This is largely due to the fact that the ICA bulb diameter estimate is greater than the diameter of the distal ICA in the normal vessel and its segment beyond the stenosis.
Like the N method, the E method is in wide use and gives consistent prognostic data regarding the risk for stroke and benefit of CEA. The E method has good reproducibility despite the subjective nature of the bulb diameter estimation, but this depends on the interpreter’s experience. In the United States, national pay-for-performance quality requirements indicate that an ultrasound (and angiography) report should specifically refer to the NASCET range or percent stenosis. DSA is an invasive, potentially hazardous, labor- and time-intensive, and expensive technique.35 The risk for groin hematoma has been reported as high as 8% in large series, although these hematomas rarely cause considerable morbidity or delay hospital discharge.36 DSA requires skilled operators and is usually done by specialists at neurovascular centers; it remains less readily available to community physicians, and this may cause delays in management of patients with an acute cerebrovascular presentation.
Delay in access is a problem given the impetus to treat patients with TIAs or minor strokes rapidly in the first 1 or 2 days after the event, when the risk for subsequent cerebrovascular accident is the highest.37 Thus, DSA—the historical gold standard in carotid luminal stenosis assessment—has now mostly been replaced by noninvasive carotid imaging techniques such as carotid Doppler ultrasound and magnetic resonance angiography (MRA), or at least less-invasive techniques such as computed tomographic angiography (CTA) or contrast-enhanced MRA. These noninvasive imaging modalities are now widely available, although access for patients varies between hospitals. Most centers now consider noninvasive techniques, alone or in combination, to be sufficiently accurate to replace DSA in the routine assessment of carotid disease.
This approach is supported by a recent meta-analysis38 in which the use of noninvasive diagnostic strategies enables more patients to receive endarterectomy more quickly than does the use of DSA, together with the evidence that rapid access to sensitive noninvasive carotid imaging prevents most strokes, thereby producing a greater net benefit.39
Carotid Stenosis Measured by Ultrasound
Using B-mode imaging, a normal arterial wall can be visualized, and the presence of early stages of carotid atherosclerosis can be detected, including the intima-media thickness (IMT), fatty streak or soft plaques, and small nonstenosing plaques (Figure 5-2). It has been suggested that a thick (i.e., >1 mm) IMT complex is strongly predictive of future vascular events.40–43
In our opinion, IMT should be routinely checked during carotid ultrasound assessment and reported when it is abnormally thick. In our laboratory, this represents the value of IMT greater than or equal to 1 mm. We anticipate the future availability of standardized measurement methods with cutoffs for reporting IMT values as validated in large prospective studies. The percentage diameter reduction of the vessel due to the plaque protruding into the vessel lumen can be measured on the longitudinal views in the absence of shadowing. When an atherosclerotic plaque is detected on a B-mode image, its presence, location length, texture, and surface should be described in the final report.44
Most importantly, the report should also say if the distal end of the plaque has or has not been clearly visualized. The reason is that a plaque extending beyond the B-mode imaging range in the neck makes the lesion not entirely accessible to the surgeon during endarterectomy. In other words, if the distal end of the plaque is not visualized, the plaque likely extends beyond the jaw level. This may lead to a cross-clamp being placed across the plaque during the endarterectomy procedure and its incomplete removal. Along with the B-mode visualization of a lesion and a decision whether it is less or greater than 50% diameter reduction, the three major Doppler velocity parameters should be reported and analyzed in terms of prediction of the NASCET range of the stenosis. These main parameters are the following:
• Peak systolic velocity (PSV)—determined from the spectrum obtained at the point of maximal narrowing
• End-diastolic velocity—determined from the spectrum obtained at the point of maximal narrowing
• Peak systolic velocity ratio—which compensates for interpatient and instrumentation variability
The PSV is mainly a function of the radius of the residual lumen as well as the length of the stenosis and the cardiac output.45–47 It represents the best single predictor of the stenosis severity.48 A variety of circulatory conditions influence the flow volume (FV) and velocity in the CCA and the ICA. In practice, individual variations of PSV and their influence on grading carotid stenosis can be reduced if the highest PSVs in the ICA and CCA are used to calculate the ICA/CCA PSV ratio. A multidisciplinary panel of experts was invited by the Society of Radiologists in Ultrasound to attend a 2002 consensus conference on diagnostic criteria to grade carotid stenosis with duplex ultrasound.34 The consensus panel determined a set of criteria most suitable for grading a focal (short and unilateral) stenosis in the proximal ICA (Table 5-1).
As the degree of the stenosis increases, the PSV increases as well as the ICA/CCA ratio. However, when the resistance across the stenosis starts to impede the velocity, causing its paradoxical decrease, these lesions are often termed hemodynamically significant (Figure 5-3). Severe stenotic lesions cause a poststenotic drop in the FV at 80% or greater diameter stenosis as shown by Spencer’s curve45 (developed for axis-symmetric and focal stenoses) (see Figure 5-3, A). The development of such a significant blood pressure gradient occurs with the lesions “on the other side” of Spencer’s curve (see Figure 5-3, B, right ICA), where the volume of blood flow is decreased through the lesion and requires compensation via distal vasodilatation and development of collaterals.
Hemodynamically significant ICA lesions are usually in the 80% to 99% diameter reduction range by the NASCET method or appear as elongated stenoses of variable diameter reduction, tandem lesions, near occlusions, or occlusions. Note that FV starts to drop at 70% narrowing according to Spencer’s curve but it becomes significant at 80% diameter reduction (see Figure 5-3, A). Often, these lesions can be discovered only by using indirect criteria for grading carotid stenosis that include both extracranial and intracranial ultrasound studies.
The indirect criteria for hemodynamically significant carotid stenosis include the following:
• Decreased end-diastolic velocity (EDV) in the CCA and/or ICA in the presence of a distal lesion
• Color flow findings such as narrow and elongated residual lumen
• Internalization of the external carotid artery (ECA; low resistance and high-velocity flow in the extracranial ECA) and reversed flow direction in the ophthalmic artery (OA)
• Anterior cross-filling via anterior communicating artery (ACoA)
• Posterior communicating artery (PCoA) flow
• Increased flow pulsatility in the unilateral CCA
• Decreased flow pulsatility in the unilateral middle cerebral artery (MCA)
• Abnormal flow acceleration and pulsatility transmission index (unilateral MCA)
These findings can also be accompanied by evidence of microembolism, particularly in the acute phase of cerebral ischemia when tandem ICA/MCA lesions and artery-to-artery embolization are common (Figure 5-4).
Identifying Vulnerable Carotid Plaques: The Role of Ultrasound
Selection for carotid revascularization therapies in recently symptomatic patients with severe carotid stenosis is largely determined by assessing the degree of luminal stenosis.49 However, there are patients who are asymptomatic or have moderate symptomatic stenoses in whom the choice between revascularization or medical intervention is less clear and for whom better methods of risk stratification are needed.50
This has led many investigators to search for markers of plaque vulnerability, instability, or thromboembolic potential as complementary to the degree of the luminal stenosis in stroke risk prediction.51 Certain morphologic features of carotid plaques are increasingly believed to be one of those markers that could carry further prognostic information, and early recognition of these plaques features may identify a high-risk subgroup of patients who might particularly benefit from aggressive interventions.52 Histologic analysis of CEA specimens suggests that vulnerable plaques are characterized by a large lipid-rich necrotic core, a thin overlying fibrous cap, an inflammatory infiltrate, neovasculature growth, and intraplaque hemorrhage.53,54 Ultrasound imaging can directly display the plaque texture and surface that would be reflective of these processes. B-mode imaging is ideally suited to determine whether or not atherosclerotic plaques are acoustically homogeneous or heterogeneous (Figure 5-5).
Depending on its echo-reflective property, a so-called “vulnerable” or “unstable” plaque is a plaque that appears predominately hypoechoic (echolucent) (Figure 5-6)—where echoes are uniform throughout all regions of the plaque—with irregular surface and possibly containing a thrombus attached to its ruptured surface. Clearly homogeneous plaques are most likely to be purely cellular in nature with little evidence of becoming complex (when calcifications, significant cholesterol deposition, or hemorrhage appear). Homogenous plaques are commonly associated with intimal hyperplasia.
Visual evaluation of plaque echogenicity has only fair reproducibility, whereas objective characterization is more reliable and less observer dependent.56 Ultrasound images can be evaluated objectively by computer-assisted gray-scale median (GSM) measurements55; however, even computer-assisted GSM measurements assess only the median brightness of the entire plaque. Regional instability, such as hemorrhage, may exist within a plaque even with a high GSM value. This may explain why there is no consensus yet on which GSM threshold value is most sensitive to distinguish vulnerable from stable plaques. A stratified gray-scale measurement of carotid plaque echogenicity57 or pixel segmentation with tissue mapping58 may be a better method to characterize plaque composition. Another limitation of conventional B-mode imaging in evaluating plaques is that interpretation of images may be hampered by artifacts. This can be minimized by applying real-time compound ultrasound imaging, which uses multiple scanning angles to improve image quality. Indeed, compared to conventional B-mode imaging, real-time compound ultrasound imaging is superior for determining plaque echogenicity, possible surface irregularities, and vessel wall demarcation with good reproducibility and high interobserver agreement.59 Another aspect of atherosclerotic plaque imaging that has been studied is its irregularity. Irregular carotid plaque surface has shown to be an independent predictor of ischemic stroke, increasing the risk nearly threefold.60,61 The exact mechanisms between irregular plaque surface and the occurrence of ischemic stroke are not yet clear. Plaque surface irregularity may represent a potential embolic source but may also be a general marker of the severity of atherosclerosis in intracranial small vessels. Recently emerged as important markers of plaque instability and higher stroke risk are microembolic signals and diminished vasomotor capacity on TCD.62,63 Both these phenomena can be detected and evaluated by ultrasound (see later). Furthermore, ultrasound can show the presence of tandem lesions in the carotid circulation that point to a high stroke risk. Published series have suggested that tandem lesions do not affect hemodynamics as a simple summation of separate degrees of stenosis.64,65 Tandem lesions and an increased risk for perioperative stroke should be considered when carotid revascularization is planned.66
Pitfalls of Carotid Duplex
• Only 15% to 25% of all strokes are attributable to a significant carotid stenosis.
• When there is high bifurcation, the carotid bulb and distal ICA cannot be fully visualized.
• A shadow longer than 2 cm can preclude sampling the highest-velocity jet and underestimate stenosis severity.
• With tandem and bilateral lesions, current criteria are unable to identify hemodynamic significance of the disease.
• ICA lesions distal to the accessible segments cannot be evaluated.
• Vertebral artery (VA) assessment is limited in patients with suspected vertebrobasilar disease, particularly intracranial vessels.
Assessment Following Carotid Endarterectomy and Carotid Stents
An important goal of the evaluation procedures following carotid revascularization is to rule out stenosis recurrence (or restenosis). B-mode imaging of carotid arteries reconstructed after CEA 67 can show changes in the vessel wall consistent with sutures, patches, stent material, early intimal proliferation, or late atherosclerotic plaque formation (Figure 5-7).
Placement of a stent in the carotid artery alters its biomechanical properties, which may cause an increase in the ultrasound velocity measurements in the absence of a technical error or residual stenotic disease. Adjustment of the velocity criteria to identify a significant restenosis is needed.68,69
Of note, the specific velocity cutoffs have been recently proposed by AbuRahma and colleagues69 for detecting in-stent restenosis of 30% or more, 50% or more, and 80% or more as follows: PSVs of 154, 224, and 325 cm/sec, respectively. PSV can increase throughout the patent stent area up to 150 cm/sec (the adopted cutoff in our laboratory). In addition to any velocity increase across the stent, we use at least a 2:1 ratio within the stent or to prestent and poststent segmental values to identify any degree of restenosis.
Our criteria for stent deformity or restenosis that include some previously published findings69–71 include the following:
• B-mode evidence for equal to or greater than 30% narrowing of the stent/vessel lumen (note that if a calcified plaque is present outside the stent with parallel walls, it may produce shadowing and false impression of vessel narrowing)
• Focal velocity increase at the point of maximal narrowing greater than 150 cm/sec and stenotic to prestenotic (pre-stent) PSV ratio of 1:≥2
• Additional evidence of plaque or thrombus formation at the site of stent deformity or at the proximal or distal ends of the stent (note that low velocities and high-resistance waveforms can be found with a subtotal obstruction of the stent)
Our criteria for stent or postsurgical thrombosis or occlusion include the following:
• B-mode evidence of hypoechoic or hyperechoic filling of the reconstructed vessel lumen (Figure 5-7) (A “crescent moon” appearance of an intraluminal thrombus without significant velocity and waveform changes could also be diagnostic and will be discussed in detail in the section on arterial occlusion.)
• An abnormal residual flow signal (i.e., stenotic, blunted, minimal, or reverberating) at the longitudinal view of the reconstructed vessel or just proximal to a flow void zone
Carotid Artery Occlusion and Dissection
With current ultrasound technologies, one could be uncertain of the diagnosis of a complete (particularly acute or “fresh” vs chronic [Figure 5-8]) carotid artery occlusion. When a patient appears to have a complete occlusion at a first-ever carotid ultrasound examination, the “benefit of the doubt” could be given by reporting “occlusion or 99% stenosis” or “near occlusion.” If there is a minimal residual lumen and flow in the distal ICA, this can change patient management (i.e., revascularization may be possible). In these circumstances the diagnostic accuracy of ultrasound in differentiation of complete occlusion from subtotal stenosis may be improved with contrast agents, and sensitive flow-imaging techniques72 and other imaging modalities are required to obtain confirmation of findings.
Our criteria for carotid artery occlusion73–75 are as follows:
1. Absent flow signal in the distal ICA on flow imaging and spectral analysis
2. High-resistance “stump” waveform with absent or reversed end-diastolic flow just proximal to the flow void area or structural lesion in the ICA
3. Drumbeat sounds of lesion motion and vessel wall covibration (usually systolic spikes of low frequency)
4. Decreased arterial wall pulsations on real-time B-mode imaging compared to the contralateral side
5. Delayed systolic flow acceleration, blunting of the MCA waveform, or evidence of flow diversion to a branching vessel (i.e., ECA), and/or collateralization of flow via OA, ACoA, or PCoA
In a patient with new-onset stroke symptoms, or a recent TIA, ultrasound can detect an acute thrombosis or an embolus in the ICA.76,77 This can be suspected in the following instances:
• Flow signal void over a lesion with hypoechoic or anechoic intraluminal appearance (possible fresh clot on B-mode) (see Figure 5-8)
• “Crescent moon” appearance of the residual lumen on color flow imaging, indicating that the hypoechoic structure is an intraluminal inclusion
• High velocity in the ECA or contralateral vessels, indicating flow diversion (in cases of flow-limiting thrombi)
• Presence of intracranial collaterals (in cases of flow-limiting thrombi)
• Microemboli found in the MCA on the side of the ICA lesion and symptoms
An acute thrombosis associated with carotid plaque usually shows an underlying atheroma that may be hyperechoic and have shadowing, whereas an acute embolus from the cardiac source may appear mostly hypoechoic and mobile.76–79 Carotid artery dissection can be detected sonographically directly in the CCA or proximal ICA, or indirectly for the distal ICA due to its common location at the entrance to the skull (Figure 5-9). The sonographer should focus on finding an intimal flap and hemodynamic effects of an upstream lesion.80,81
Carotid dissection can be suspected with the following findings:
• Intimal flap is visualized in the carotid artery with abnormal flow waveforms (differentiate B-mode artifacts caused by jugular vein walls, its valves, or other bright reflectors) (see Figure 5-9, bottom).
• High-resistance pulsatile flow signals are found proximal to hemodynamically significant dissections without evidence for an atheromatous lesion (see Figure 5-9, bottom).
• Two waveforms are identified, reflecting flow through the true and false lumens.
• Reversed OA or other intracranial collaterals could be found.
• Microemboli could be found in the MCA on the side of the suspected dissection.
• If a dissection is found in the CCA, suspect its origin in the aortic arch and check other proximal precerebral vessels.
• Recovery of end-diastolic flow in the distal ICA (low-resistance flow) or proximal to the lesion
• Return of normal systolic flow acceleration in the MCA without collateralization of flow
Vertebral Artery Stenosis or Occlusion
Compared to carotid imaging, fewer validation studies are available for detection and quantification of the VA lesions.82,83 VA stenosis occurs more often in V3-V4 segments followed by the origin of the VA (V0), and midcervical section (V1-V2). Direct assessment of the V3 segment with ultrasound is not possible.84
Our diagnostic criteria for VA stenosis (Figure 5-10) include the following:
1. Focal significant PSV increase with the ratio of the stenotic to the pre- or poststenotic peak systolic velocities ≥2 (usually the highest PSV exceeds 100 cm/sec to reach any significance of this finding)
2. When detectable, the presence of a structural lesion on B-mode, or turbulence, spectral narrowing, or additional abnormal waveforms on Doppler at the site of the lesion
3. Indirect prestenotic or poststenotic signs (abnormal pulsatility and waveforms)
Our criteria for VA stenoses do not include a PSV cutoff since tortuosity of the proximal VA segment, compensatory velocity increase with ICA lesions, and VA dominance may produce relatively high velocities.
Our diagnostic criteria for VA occlusion are as follows:
Normal Intracranial Flow Findings
When performing and interpreting transcranial Doppler or duplex examinations, sound, waveform appearance, blood flow direction, actual velocity, and pulsatility measurements should be considered (Table 5-2).
TABLE 5-2 Normal Depth, Direction, and Mean Flow Velocities at Assumed Zero Degree Angle of Insonation of the Arteries of the Circle of Willis
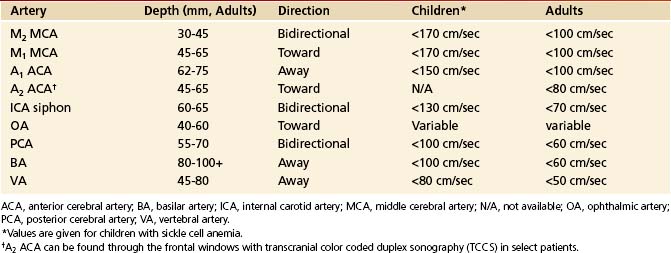
Our criteria for normal TCD examination (Figures 5-11 and 5-12) include the following:
• Direction of blood flow and depths of insonation
• The difference between blood flow velocities in the homologous arteries is less than 30%: 15% is attributable to the difference in angle of insonation and another 15% to breathing cycles. However, posterior cerebral and vertebral arteries may have 50% to 100% difference because of dominance, hypoplasia, and tortuous course. Similarly, M2 and M1 MCAs may have up to 100% variation in velocity depending on tortuosity.
• A normal M1 MCA mean flow velocity (MFV) does not exceed 170 cm/sec in children with SCD and 100 cm/sec in adults free of anemia.
• A normal velocity ratio: MCA is greater than or equal to anterior cerebral artery (ACA), which is greater than or equal to siphon, which is greater than or equal to posterior cerebral artery (PCA), which is greater than or equal to basilar artery (BA), which is greater than or equal to VA. Velocity values can be equal between these arterial segments or sometimes exceed by 5 to 10 cm/sec (i.e., ACA > MCA, or BA > MCA), likely due to the angle of insonation or common anatomic variations.
• Patients free of hypertension while breathing room air have a positive EDV of approximately 25% to 50% of the PSV values and a low-resistance pulsatility index of Gosling and King (PI)85 of 0.6 to 1.1 in all intracranial arteries. A high-resistance flow pattern (PI >1.2) is seen in the OAs only.
• High-resistance flows (PI >1.2) can be found in patent cerebral arteries with aging, chronic hypertension, and increased cardiac output and during hyperventilation.
The range of PI, described by Gosling and King,85 that can be found in the arteries supplying the brain is calculated as follows:
Besides PI, the resistance to flow can be expressed using the resistivity index (RI) described by Pourcelot.86 This index is calculated as the ratio of (PSV − EDV) / PSV with normal values below 0.75. There is a controversy about which index better describes the resistance to flow since PI may be more influenced by cardiac output whereas RI is more reflective of the distal resistance.87
Intracranial Atherosclerotic Disease
Intracranial atherosclerotic disease is now recognized as a serious risk factor carrying the highest risk for stroke recurrence.88
It is more prevalent in Asian, Hispanic, and African American populations. Patients who have had a stroke or TIA attributed to stenosis (50%-99%) of a major intracranial artery face a 12% to 14% risk for subsequent stroke during the 2-year period after the initial ischemic event, despite treatment with antithrombotic medications. The annual risk for subsequent stroke may exceed 20% in high-risk groups.88 The recent emphasis on identification of stenoses greater than or equal to 50% and greater than or equal to 70% prompted reevaluation of the previous criteria and development of new ones (Table 5-3).89
TABLE 5-3 Maximum Mean Flow Velocity Thresholds at Assumed Zero Angle of Insonation for a Focal (≥50%) Intracranial Arterial Stenosis on TCD
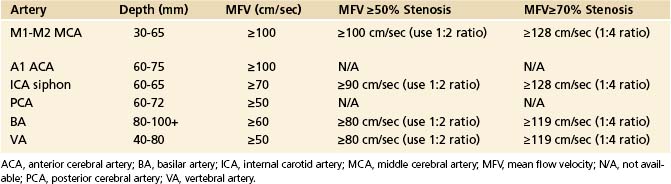
Middle Cerebral Artery Stenosis
Primary findings for any significant MCA stenosis (i.e., > 50% diameter reduction) include a focal significant MFV increase (MFV >100 cm sec) (Figure 5-13), or PSV increase (PSV >140 cm/sec), or interhemispheric MFV difference of 1:2 in adults free of abnormal circulatory conditions.90–92 A stenosis greater than or equal to 70% in the MCA could produce higher velocities, and in our laboratory we use an MFV cutoff of 120 cm/sec or greater (Figure 5-13). This velocity increase should further be associated with a ratio to a homologous segment of 1:4 or higher.
With MCA stenoses “on the other side” of Spencer’s curve, a paradoxical flow velocity decrease can be found in the presence of findings such as flow diversion that indicates hemodynamic significance of a lesion. A proximal M2-distal M1 MCA stenosis is present if the velocity increase is found at 30 to 45 mm. A proximal M1 MCA stenosis is usually found at 45- to 65-mm depths in adults.93,94
Chimowitz and colleagues95 in the prospective part of the Warfarin-Aspirin Symptomatic Intracranial Disease (WASID) study adopted criteria that the MCA MFV equal to or greater than100 cm/sec indicates M1 MCA diameter reduction of 50% or more. An algorithm was developed on how to measure intracranial stenosis on DSAs. A recent meta-analysis of available studies indicated that the 100 cm/sec cutoff yielded a mean weighted average sensitivity of 100% and specificity of 97%.92
To improve the predictive value of any chosen velocity threshold, we use the ratio with a homologous or proximal MCA segment.91 There is an increased interest in defining the most severe intracranial stenoses because these patients have the highest risk for stroke recurrence, and novel stenting technologies are now being tested as an adjunct to the best medical therapy for secondary stroke prevention.
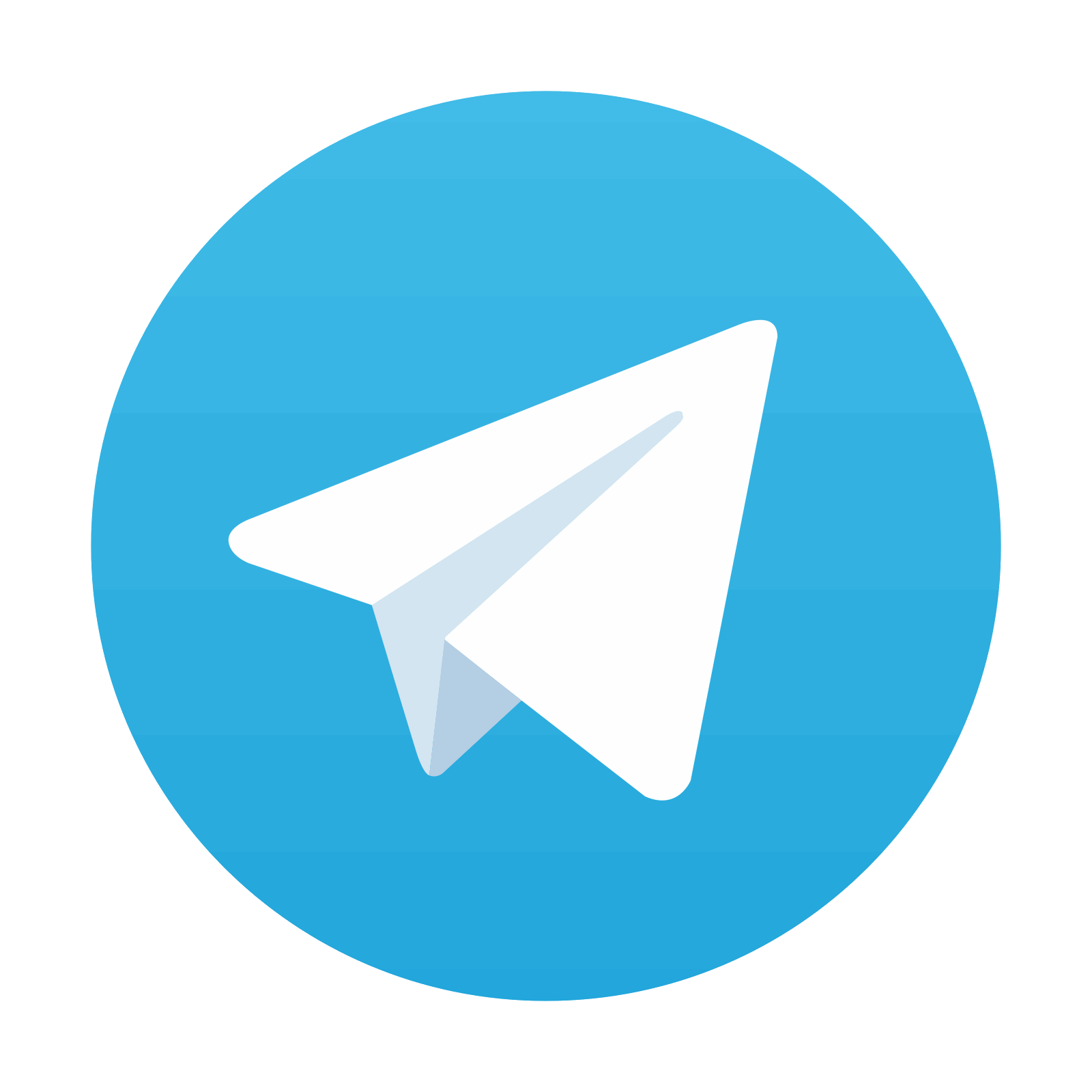
Stay updated, free articles. Join our Telegram channel

Full access? Get Clinical Tree
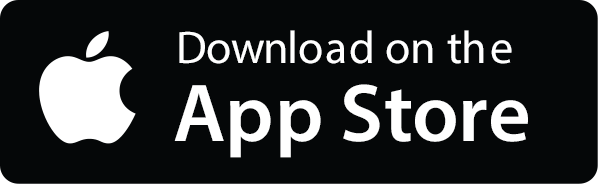
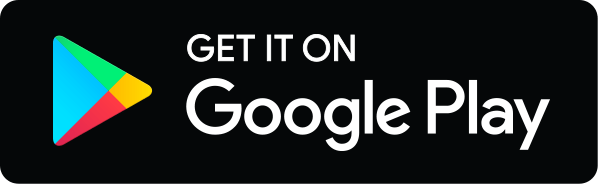