© Springer International Publishing Switzerland 2017
Luis Martí-Bonmatí and Angel Alberich-Bayarri (eds.)Imaging Biomarkers10.1007/978-3-319-43504-6_11. The Shift in Paradigm to Precision Medicine in Imaging: International Initiatives for the Promotion of Imaging Biomarkers
(1)
Department of Biomedical Imaging and Image-guided Therapy, Center of Excellence for High Field MR, Vienna, Austria
1.1 Background
Qualitative, subjective interpretations of medical images, which have been the backbone of image interpretation for the past century, provide useful information to the treating physician. This procedure will continue for some time into the future, since the alternative method of automatic reading of images by artificial intelligence is not developed enough to replace the trained and experienced observer with his/her ability to interpret and judge during image reading sessions. Nevertheless, subjective and, therefore, qualitative interpretations are observer dependent and highly variable, and variability inevitably degrades outcomes in healthcare in general. Extracting objective, quantitative results from medical images is one way to reduce the variability associated with medical image interpretation and, thus, obtain relevant and useful quantitative results, which will improve patient outcomes.
During the past two decades, advances in medical imaging technology have offered the possibility to extract high-resolution anatomic, physiologic, functional, biochemical, and metabolic information from clinical images, all of which reflect the molecular composition of the healthy or diseased tissue of organs imaged in the human body. With appropriate calibration, most of these imaging technologies can provide quantitative information about specific properties of the tissues being imaged.
The computed tomography (CT) signal is linearly proportional to atomic density and has high spatial resolution. It can provide accurate distance measurements (e.g., tumor dimensions or volumetry [1]), basic tissue characterization, quantitative physiologic information related to perfusion [2, 3] or necrosis [4], angiographic information, and quantitative functional information using dynamic contrast techniques.
The positron emission tomography (PET) signal is linearly proportional to atomic decay events and has high sensitivity. If the physiology of the labeled substance being detected is known, it can be related to the PET signal magnitude of the molecular substrates of interest. [5] PET radiopharmaceuticals can be developed to assay a wide variety of physiologic or molecular functions. CT provides attenuation correction data for PET imaging with PET/CT, although contrast-enhanced CT will also give diagnostic information [6].
Magnetic resonance imaging (MRI) signals are complex: they are quantitatively, but nonlinearly, related to T1 and T2 relaxation phenomena, as well as proton density distribution and/or other physiologic and functional features, such as flow, permeability, diffusion, and cell density, among others. Nevertheless, with appropriate calibration and standardization, MRI techniques can be devised in which the signal response is a quantitative indicator of tissue structure and function [7–11].
Ultrasound data are also complex, but, again, with appropriate calibration and standardization, quantitative information about attenuation, refraction, reflection, bulk tissue properties, and shear wave speed of the tissues being imaged can be calculated. In addition, quantitative distance, elasticity, and Doppler flow measurements can be extracted from ultrasound signals [12].
Therefore, these imaging methods can be considered useful as imaging biomarkers and are comparable to biomarkers from laboratory assays. The term “quantitative imaging” has recently been formally defined as “the extraction of quantifiable features from medical images for the assessment of normality, or the severity, degree of change, or status of a disease, injury, or chronic condition relative to normal” (QIBA).
Fundamentally, quantitative imaging involves the measurement of some variables using medical images, and, most meaningfully, quantitative imaging is based on an underlying biophysical model for the tissue of interest. It involves the measurement of image-related properties that are analyzed according to that model. This results in an unbiased, quantitative estimate of a physical or biological parameter [13].
In general, a biomarker represents a characteristic that is objectively measured and evaluated as an indicator of normal biologic processes, pathogenic processes [14], or biological responses to a therapeutic intervention. Measurements of anatomical, physiological, functional, biochemical, and metabolic characteristics of the body through medical imaging are referred to as imaging biomarkers and have gained increasing attention from the medical community in the last several years. Imaging biomarkers are becoming increasingly used in clinical research for drug development and clinical decision-making [15].
Imaging biomarkers include the development, standardization, and optimization of imaging acquisition protocols, data analyses, display methods, and reporting structures. These are requirements for the validation of accurately obtained image-derived metrics with anatomically and physiologically relevant parameters in different oncologic, as well as non-oncologic diseases, in different organs of the human body. These requirements are also necessary for the monitoring of treatment response. Therefore, the use of such metrics will play a crucial ever-increasing role in research and patient care [2].
The molecular bases of health and disease have become increasingly well understood in the past 20 years. In fact, the molecular characterization of disease has revealed that each patient is likely to have a unique combination of genotypic and phenotypic profiles for a specific disease. Healthcare delivery is now focused on trying to determine the most appropriate therapy for any patient’s molecularly unique version of a particular disease [16]. This concept is referred to as precision medicine, and it has recently been identified as a national priority in the USA, with a large initiative to explore and implement such practices that would result in the need for tests that can provide objective, reproducible information for clinical research and practice [17].
Biomarkers in general—both specimen and imaging—play an increasingly important role in healthcare by providing more information about molecular processes. Specimen biomarkers are typically obtained from body fluids or a tissue sample (biopsy). The main disadvantage, however, is that, in most cases, the sample represents only a small portion of the normal or diseased tissue of interest, and, therefore, spatial sampling bias is a serious problem. Imaging scans, on the other hand, typically cover a broad segment of tissue, or even the entire patient, and potentially provide more comprehensive information about heterogeneity. Another disadvantage of specimen biomarkers from biopsies is that they represent a single time point, whereas imaging data can be obtained dynamically and longitudinally in the individual patient without the need for repeated invasive procedures. For these reasons, imaging biomarkers are of considerable interest in evidence-based clinical decision-making, as well as for therapeutic development and treatment monitoring. Whereas for in vitro laboratory specimen assays, standard terminology and methods have become established to describe, evaluate, and validate assays for medical applications for many years, the same approach to standardization for imaging data has only begun to occur in an organized way in the past few years.
It is expected that the added value of objective, quantitative imaging biomarkers in both research and clinical applications will increase as healthcare initiatives place increased pressure on imaging physicians to provide evidence-based decisions and care. The demand for quantitative results from imaging studies will increase as treatment decisions are driven by such results. Fortunately, contemporary computing power and image processing methods are such that quantitative results can be obtained from current digital medical images at little or no increased cost, which will allow efficient integration of quantitative imaging into the radiologist’s workflow as an added value, with minimal or no increased cost to the healthcare system.
However, one of the basic requirements for establishing the link between quantitative imaging results and improved patient outcomes is the availability of robust, reliable, accurate, reproducible, and validated quantitative results from clinical trials. There are still substantial hurdles to the achievement of reproducible quantitative imaging measures in clinical practice, but particularly in clinical trials. There are several sources of bias and variance in the quantitative results obtained from clinical images, which can be grouped into three main categories: the image acquisition hardware, software, and procedures, the measurement methods used, and the reader variability. Thus, the entire chain involved in producing a clinical image must be approached from a systematic perspective. Considerable work is needed to validate specific metrics, improve standardization across vendor platforms, and educate imaging physicians about the reliability of quantitative imaging. Validating an imaging biomarker requires identifying and characterizing all of the sources of bias and variance that could affect the end measurement.
The process of acquiring a clinical imaging scan is complex; therefore, the goals of standardization and improved reproducibility require coordinated activity among imaging device and software manufacturers, regulatory organizations, healthcare providers, academic institutions, groups using imaging in clinical trials, and professional societies. An additional challenge in the efforts to improve reproducibility in quantitative clinical imaging is the continual technological advancement that occurs in medical imaging hardware and software. Thus, the quantitative accuracy of medical images must be continually reassessed, and the standardization requirements have to be periodically updated.
Examples for such a collaborative activity that attempts to address these issues are the Quantitative Imaging Biomarkers Alliance (QIBA) in the USA and the European Imaging Biomarkers Alliance (EIBALL) in Europe.
1.2 Quantitative Imaging Biomarkers Alliance (QIBA)
The Quantitative Imaging Biomarkers Alliance (QIBA) was organized in 2007 by the RSNA to unite researchers, healthcare professionals, and industry stakeholders in the advancement of quantitative imaging [18]. QIBA draws from the very successful precedents set by the DICOM and Integrating the Healthcare Enterprise (IHE) efforts, but has been adapted to the needs of imaging science. Strategic guidance supporting the development, qualification, and deployment of imaging biomarkers will lead to improved standardization of imaging tests, proof of imaging test performance, and greater use of imaging to predict tissue biologic behavior and to monitor therapy response.
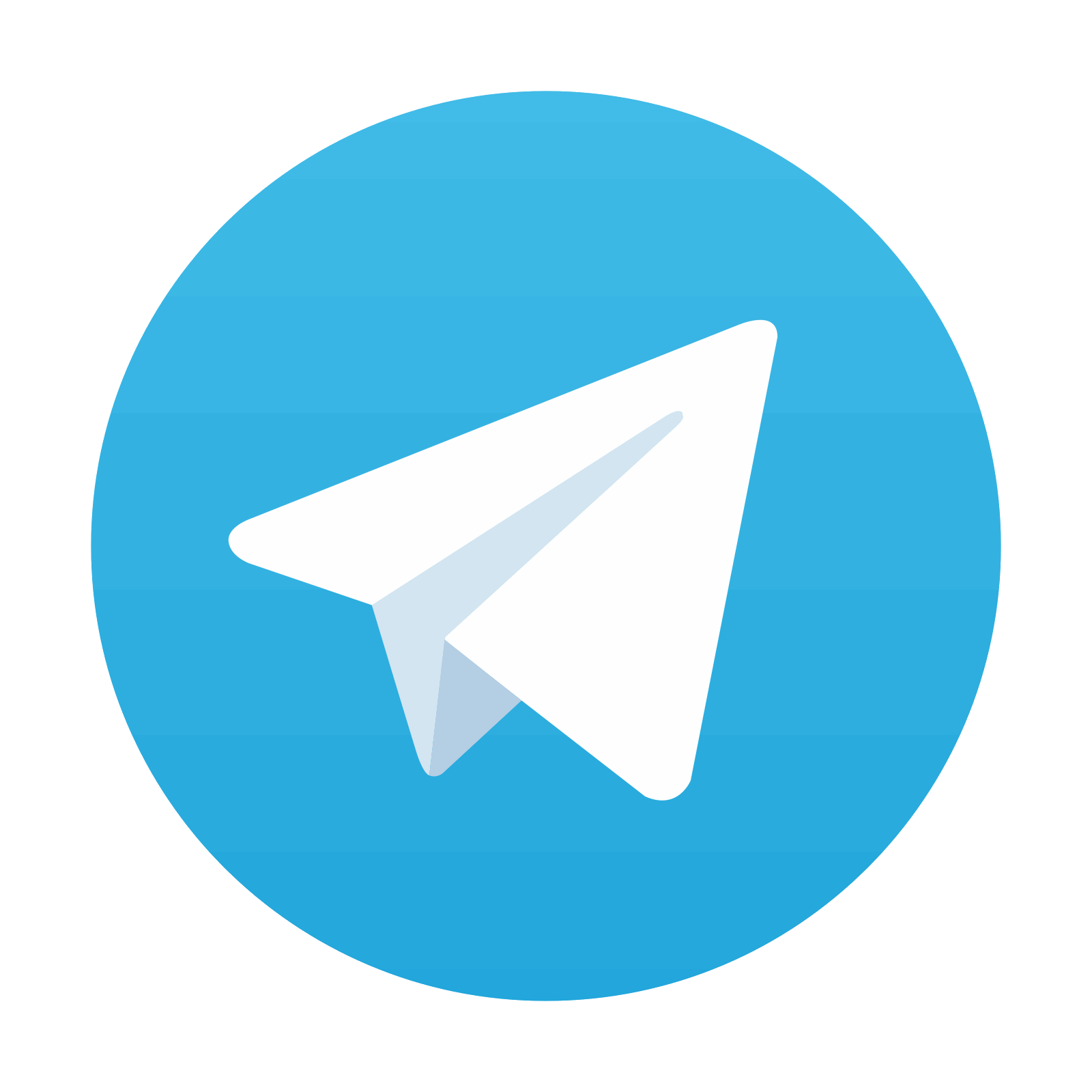
Stay updated, free articles. Join our Telegram channel

Full access? Get Clinical Tree
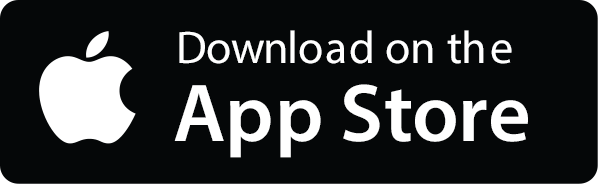
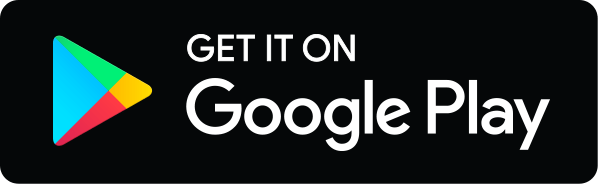