Made up of inorganic calcium hydroxyapatite (Ca 10 [PO 4 ] 6 [OH] 2) crystal and an organic matrix of collagen and blood vessels, the skeleton is constantly changing and remodeling. This physiological activity can be imaged with radioactive analogs of calcium, phosphate, or hydroxyl ions (OH – ) that can localize to the bone, with areas of growth or repair resulting in increased turnover. Whereas conventional imaging methods such as radiographs and computed tomography (CT) examine the anatomical features of structures, nuclear medicine techniques are often much more sensitive for disease because they evaluate function in an organ or system.
The bone scan uses a radiopharmaceutical composed of radioactive component, technetium-99m (Tc-99m), joined with a localizing carrier molecule, e.g., methylene diphosphonate (MDP). It is a highly versatile examination, able to assess the effects of tumor, infection, trauma, arthritis, and metabolic bone disease. Because of its ability to image the entire skeleton with high sensitivity at a reasonable cost, it remains widely used decades after its introduction, despite technological advances in CT and magnetic resonance (MR) imaging.
Scintigraphic image quality has improved dramatically over time, due to advances in camera detector technology and processing software. Accuracy is also improved through the use of three-dimensional (3-D) single-photon emission computed tomography (SPECT). In addition, as uptake may be the result of many different processes, CT correlation can be used to explain the etiology of nonspecific abnormal activity. Fusing the CT to the SPECT is especially helpful in correcting the low specificity of the bone scan. This fusion will very often be better when images are acquired on a hybrid SPECT/CT scanner.
Positron emission tomography (PET) images are generally superior to those produced from traditional single-photon imaging agents such as Tc-99m MDP. In recent years, the rapid spread of fluorine-18 fluorodeoxyglucose (F-18 FDG) PET/CT has changed how many diseases are assessed, particularly cancer. F-18 FDG examinations often complement or supplement the abilities of the bone scan. Another PET agent, F-18 sodium fluoride (F-18 NaF), is a highly sensitive bone-imaging radiopharmaceutical that can produce images in a much shorter time than is possible with Tc-99m MDP. Although uptake is not specific, occurring in benign and malignant processes, sodium fluoride PET/CT has also demonstrated greater accuracy than bone scan. Clinical use of F-18 NaF, however, has been limited in the United States because of issues with reimbursement.
This chapter examines common scintigraphic bone-imaging techniques, using Tc-99m MDP, radiolabeled white blood cells, Tc-99m sulfur colloid bone marrow scan, and F-18 NaF PET/CT (F-18 FDG PET is more thoroughly covered in Chapter 13 ). In addition, targeted radionuclide therapy of metastatic disease with bone-localizing beta-emitters (i.e., strontium-89 [Sr-89], samarium-153 [Sm-153], rhenium-186 [Rh-186], and phosphorus-32 [P-32]) and the more recently approved alpha-emitter radium-223 (Ra-223) is discussed. Finally, osteoporosis and bone-density measurement with dual-energy x-ray absorptiometry (DEXA) will be reviewed.
Radionuclide Imaging of the Skeleton: Radiopharmaceuticals
An ideal radiopharmaceutical must be inexpensive, remain stable, rapidly accumulate at the target, and quickly clear from background tissues. It should also have favorable imaging and dosimetry characteristics. For single-photon gamma camera imaging, Tc-99m meets these criteria, with its desirable 140-keV gamma photon and 6-hour half-life. In the case of PET, all agents emit two 511-keV annihilation photons that travel at 180 degrees from each other. Imaging is most commonly performed with fluorine-18. The 109.8-minute half-life is short enough to minimize patient dose yet long enough to allow for production and distribution from offsite cyclotron and radiopharmacy facilities in addition to the time required for the exam.
Technetium-99m MDP
The combination of Tc-99m with a phosphate analog carrier molecule creates an agent that can demonstrate skeletal turnover. Initially, pyrophosphates (Tc-99m PYP) were used, characterized by their P–O–P bond ( Fig. 6.1 ). However, agents containing a diphosphonate structure were ultimately found superior: Their P–C–P bond is more stable and allows faster background clearance by renal excretion. Tc-99m hydroxymethylene diphosphonate (Tc-99m HMDP or HDP) and Tc-99m methylene diphosphonate (Tc-99m MDP) are both able to demonstrate a high level of detail, although Tc-99m MDP is more commonly used ( Fig. 6.2 ).
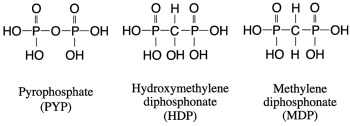
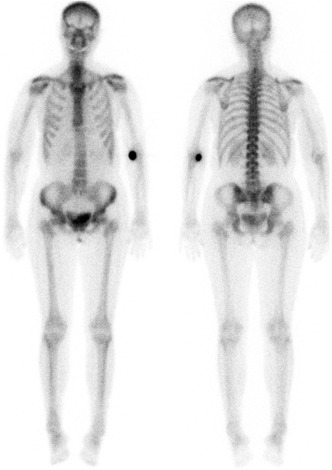
Preparation
Tc-99m MDP can be prepared from a simple kit. Tc-99m, in the form of sodium pertechnetate (Na 99m TcO 4 ) obtained from the technetium-molybdenum generator, is injected into a vial containing MDP, stabilizers, and stannous ion. Stannous tin (Sn II) acts as a reducing agent, allowing the Tc-99m pertechnetate to form a chelate bond with the MDP carrier molecule.
Incomplete labeling may occur if air is introduced into the vial because oxygen causes hydrolysis of the stannous ion (from Sn II to Sn IV). Insufficient stannous ion results in free technetium pertechnetate (“free tech”), causing image degradation with increased background soft tissue activity and uptake in the thyroid, stomach, and salivary glands. Tc-99m MDP should be used within 2 to 3 hours of preparation, or radiopharmaceutical breakdown may also yield free technetium pertechnetate. Excess alumina from the technetium generator eluate may lead to colloid formation, which can be seen as uptake in the reticuloendothelial system of the liver.
Uptake and Pharmacokinetics
The injected Tc-99m MDP rapidly distributes into the extracellular fluid and is quickly taken up into bone. Although accumulation relates to the amount of blood flow to a region, uptake is primarily the result of osteogenic activity, being much higher in areas of active bone formation and repair than in mature bone ( Fig. 6.3 ). Tc-99m MDP binding occurs by chemisorption in the hydroxyapatite mineral component of the osseous matrix. Accumulation in areas of amorphous calcium phosphate may account for the Tc-99m MDP uptake sometimes seen in sites outside the bone, such as dystrophic soft tissue ossification. Decreased activity is seen in areas of reduced or absent blood flow or infarction. Diminished uptake or cold areas are also often seen in lytic metastases.
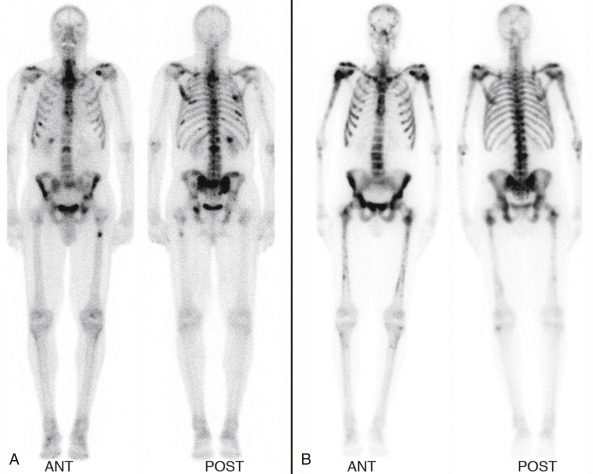
Approximately 50% of the dose is localized to the bone, with the remainder excreted by the kidneys. Although peak bone uptake occurs approximately 1 hour after injection, the highest target-to-background ratios are seen after 6 to 12 hours. Images are typically taken at approximately 3 hours to balance the need for background clearance with the relatively short 6-hour half-life of Tc-99m and patient convenience. Also, the radiotracer half-life limits imaging to a maximum of 24 hours after injection. Radiopharmaceutical dosimetry is discussed in Appendix 1 .
Imaging Protocol of Tc-99m MDP
The patient should be well hydrated and, after injection, should be instructed to drink several cups of fluid to improve background clearance. Frequent bladder voiding reduces the radiation dose. Care must be used because urinary contamination can cause confusion or mask potential lesion sites.
There are three main phases that can be imaged with a bone scan. The first phase involves rapid dynamic image acquisition immediately after injection to assess blood flow to an area of concern. This can be followed by the second, soft tissue, phase, which lasts a few minutes. Delayed images that visualize the bones make up the third phase. Occasionally, further delay, a so-called fourth phase, is still needed at 18 to 24 hours to clear soft tissue activity and maximize target-to-background ratios.
Before injection, a decision must be made as to whether or not blood flow and soft tissues need to be evaluated as part of a three-phase examination or if routine delayed images alone will suffice. Box 6.1 lists uses for both acquisition protocols. Metastatic disease and back pain assessment can be done by delayed imaging alone. The presence of increased arterial blood flow and abnormal soft tissue activity can aid in the diagnosis of several additional acute problems: osteomyelitis, painful joint prosthesis, fracture, avascular necrosis (or osteonecrosis), bone graft status, and complex regional pain syndrome (previously called reflex sympathetic dystrophy). A sample protocol for three-phase and routine delayed scanning is listed in Box 6.2 , and an example of the parameters used for SPECT imaging is summarized in Box 6.3 .
Three-Phase Bone Scan
Infection
Osteomyelitis
Cellulitis
Diabetic ulcers and Charcot joint with potential osteomyelitis
Septic joint
Painful joint prosthesis—infection or loosening
Fracture
Posttraumatic
Shin splints/stress fracture
Occult hip fracture in the elderly
Avascular necrosis/osteonecrosis
Regional pain syndrome (reflex sympathetic dystrophy)
Bone-graft status
Heterotopic bone maturity in paralyzed patient
Routine Bone-Scan Applications (delayed imaging only)
Metastatic disease
Evaluation of lower back pain (underlying vessel background limits use in early phases)
Spondylolisthesis (single-photon emission computed tomography [SPECT] highly important)
Discitis/vertebral osteomyelitis
Compression fracture assessment
Suitability for vertebroplasty
Investigate possible relationship to metastases, identify lesions elsewhere
Patient Preparation
Well-hydrated patient
Void bladder immediately before study (and frequently for next several hours).
Remove metal objects (jewelry, coins, keys) before imaging.
Radiopharmaceutical Administration
Select injection site to avoid possible sites of pathology.
Adult dose: 20 mCi (740 MBq), intravenously
Pediatric dose: 0.25 mCi/kg (9.3 MBq/kg), with a minimum dose of 1.0 mCi (37 MBq)
Acquisition
Low-energy high-resolution collimator
Energy window centered at 140 keV with 15% to 20% window width
If three-phase exam desired:
Phase 1: Perfusion
Camera over area of interest. Inject bolus, begin imaging as activity 1 to 3 sec/frame for 1 minute, 64 × 64 or 128 × 128 matrix.
Phase 2: Blood pool
Image immediately after flow (multiple areas possible for 3-5 minutes), 150,000 to 300,000 counts/image, 128 × 128 matrix.
Phase 3: Routine delayed images
Whole-body planar images: Camera detectors anterior and posterior to patient; detector speed set at 10 to 15 cm/min; matrix 1024 × 256
Planar spot views (optional): 4 to 10 minutes each (based on time required for 500,000 count images of the chest 300,000 to 1,000,000); <SPAN role=presentation tabIndex=0 id=MathJax-Element-1-Frame class=MathJax style="POSITION: relative" data-mathml='≥’>≥≥
≥
150,000 to 250,000 counts for images of the skull and distal extremities
Additional Options
Extended delay planar images (fourth phase): 6 to 24 hours postinjection
Pinhole collimator images: 75,000 to 100,000 count/image; zoom magnification
High resolution and magnified for children and small joints
SPECT
Detectors 360°degree circular orbit, 60 to 120 stops, 15 to 30 sec/stop, 128 × 128 matrix (or greater)
CT acquisition for dedicated SPECT/CT: multislice spiral or cone-beam, 512 × 512 matrix, tube 80 to 120 kV and 3 to 300 mAs (varies but recommend use of dose-reduction software)
Reconstruction
Two possible methods:
3-D iterative reconstruction with 3 to 5 iterations and 8 to 10 subsets; attenuation correction using the CT images; manufacturer’s resolution recovery software
Filtered back-projection
Postprocessing filter: Butterworth (cutoff 0.4, power 7) or gaussian
Display
2-D axial, coronal, sagittal planes, fused or unfused to CT
Patient Preparation
Hydrate (e.g., 1-2 cups water), voids bladder prior to going on scanner
Note: F-18 FDG rules concerning insulin and fasting do not apply.
Radiopharmaceutical Dose
Adults: 0.04 to 0.1 mCi/kg (1.5-3.7 MBq/kg) intravenously, typical dose 5 to dose 10 mCi (185 to 370 MBq); maximum dose considered for obese patients
Pediatric: 0.06 mCi/kg (2.2 MBq/kg); minimum 0.05 mCi (18.5 MBq), maximum 5 mCi (185 MBq)
Acquisition and Processing
30- to 60-min postinjection delay (90-120 min for extremities)
1- to 2-min/bed position (time-of-flight scanner); 128 × 128 matrix
CT for attenuation correction/localization: 30 mA/120 kVp, rotation 0.5 sec, and pitch 1.0
Reconstruction parameters similar to F-18 FDG PET.
If dynamic three-phase scanning is to be performed, a bolus of Tc-99m MDP is injected intravenously with the area in question under the camera ( Fig. 6.4 ). The injection site should be chosen to avoid any suspected pathological condition. For example, if comparison with the opposite hand may be needed at any time, injection in a site such as the foot should be considered. The first phase consists of serial 1- to 4-second dynamic blood flow images acquired for 60 seconds. Then blood pool or soft tissue second-phase images are obtained of the main region and any secondary areas of interest, such as in patients with arthritis or multiple stress injuries. The timing of delayed images may vary, from 2 hours in younger patients up to 3 to 4 hours in the elderly, obese, and in those with poor renal function.
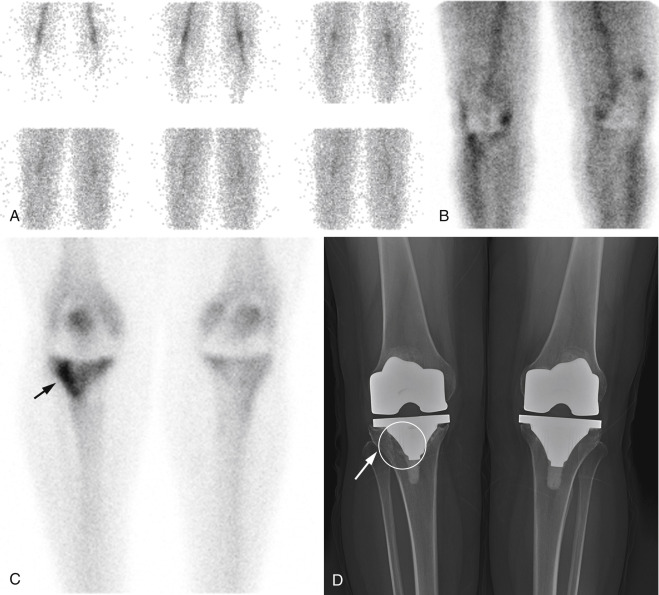
Using a low-energy, high-resolution collimator, delayed planar images can be obtained by whole-body scan or spot views. The whole-body scan allows rapid, seamless coverage as the camera moves over the patient at a predetermined rate. On the other hand, spot views, with the camera fixed over each area to be imaged, provide greater resolution and detail. In most centers, a whole-body scan is performed, with high-count spot views reserved for symptomatic areas or additional views of suspicious-appearing regions ( Fig. 6.5 ).
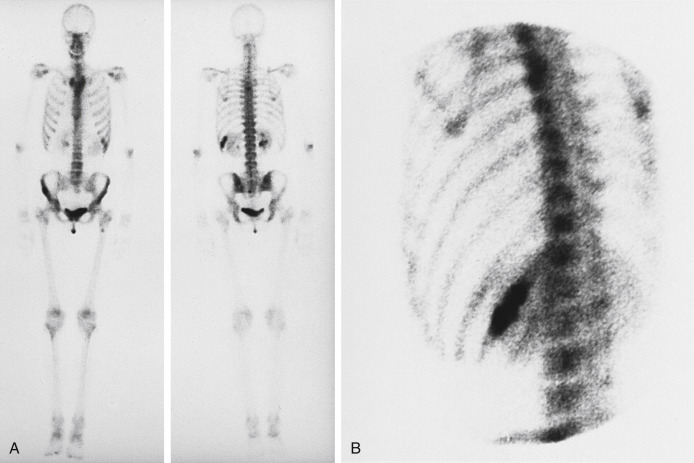
Other modifications can be performed. Magnified views with a pinhole collimator can better visualize joints in children, osteonecrosis of the hips, and trauma to the carpal bones. In SPECT, a volume of data is acquired from camera detectors orbiting the area of interest, and the reconstructed 3D images can be formatted in transaxial, sagittal, and coronal planes. SPECT images provide better resolution, more precise localization, and improved contrast of cold and hot lesions ( Fig. 6.6 ). Not only can this increase sensitivity, but more exact localization can increase specificity by avoiding confusion with benign uptake ( Fig. 6.7 ).
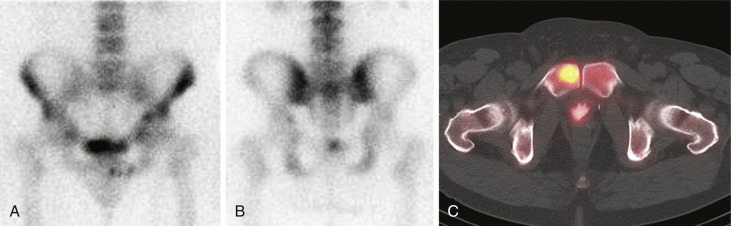
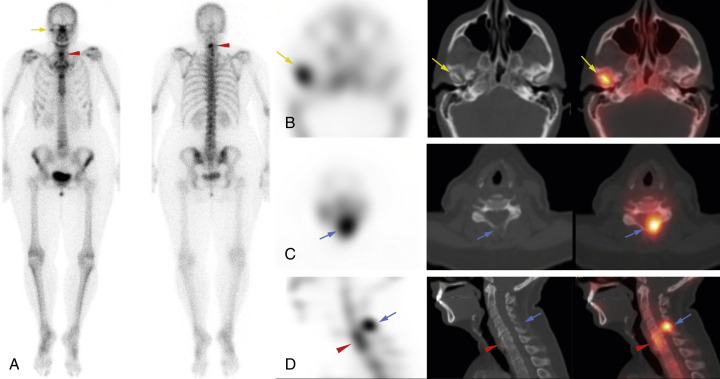
Correlating findings with CT is often key to making the correct diagnosis. When no radiographic abnormality is seen to explain activity, suspicion is increased that uptake is the result of early metastasis. Although commercially available software can fuse the SPECT with a CT obtained at another time, studies acquired on a hybrid SPECT/CT scanner usually result in superior results because of the more uniform slice thickness and positioning between the two examinations and because of the elimination of the chance that the pathology will change in the interval between studies.
F-18 Sodium Fluoride (F-18 NaF) PET/CT
F-18 NaF was originally approved as a bone-imaging radiopharmaceutical by the U.S. Food and Drug Administration (FDA) in 1972. However, the high-energy 511-keV photons of the PET tracer were not well suited for use with the gamma cameras available at the time, and F-18 NaF was replaced following the introduction of technetium-99m–labeled radiopharmaceuticals. F-18 NaF was listed as a discontinued drug in 1984, remaining out of general use until dedicated PET and then PET/CT cameras became widely available nearly two decades later. Reexamination of sodium fluoride PET/CT demonstrated high sensitivity for tumor involvement in bone ( Fig. 6.8 ).
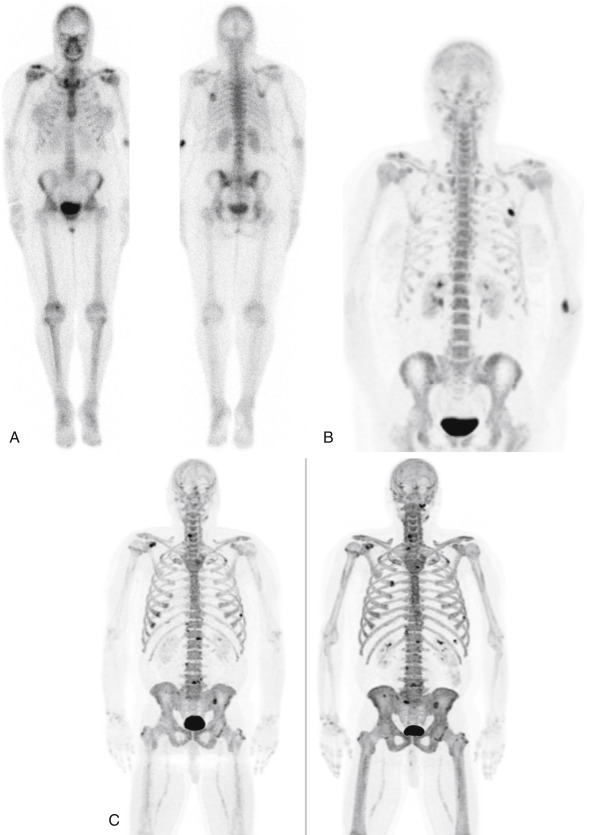
Clinical use of sodium fluoride has been limited by issues with reimbursement. The U.S. Centers for Medicare and Medicaid (CMS) recently ruled there was insufficient evidence to support reimbursement of these scans, despite a large multicenter trial performed as part of the National Oncologic PET Registry (NOPR) that showed a significant impact of F-18 NaF on patient management. Additionally, after shortages of Tc-99m occurred due to serious issues with the aging nuclear reactors used to produce many medical radioisotopes, F-18 NaF was touted as a potentially critical alternative to Tc-99m, as F-18 is made in a cyclotron rather than a reactor.
F-18 NaF Imaging Protocol
After intravenous injection, F-18 NaF localizes to areas of new bone formation through chemisorption, in a similar fashion to that of Tc-99m MDP. However, sodium fluoride is not highly protein bound in the blood, resulting in rapid renal clearance.This allows imaging to begin as early as 30 to 45 minutes (although a longer delay, 60-90 minutes, will result in superior images). Given the high target-to-background ratios and excellent resolution, PET images can be performed without CT attenuation correction. However, intensity is more uniform with attenuation correction, preventing bones in areas subject to less attenuation from falsely appearing more intense than those where photons are more likely absorbed or scattered. CT is also very useful to localize lesions to areas of bone pathology and differentiate malignancy from benign processes.
The low-dose whole-body CT requires only seconds. This is followed by the emission PET scan data, collected as the patient moves through the scanner in a series of bed positions, each covering several centimeters at 1 to 2 minutes per bed position. A few sites have advocated the use of a “cocktail” combining F-18 FDG and F-18 NaF in order to assess the bones and soft tissues in one session.
Patients should be well hydrated, void their bladders frequently, and continue to drink extra fluids for a few hours after injection to minimize radiation to the bladder. Although the higher energy of PET photons can lead to increased radiation doses to the patient, its relatively short half-life of 109.8 minutes helps limit exposure, which is outlined in Appendix 1 .
Image Interpretation
Normal and Altered Distribution
The appearance of the bones varies dramatically with age. Most notably, the growing skeleton will concentrate radiotracer at all active growth plates ( Fig. 6.9 ). These areas are also often the critical sites in trauma, primary bone tumors, and osteomyelitis. Therefore, it is essential that children are immobilized and positioned symmetrically in terms of rotation and distance from the camera face. By adulthood, growth-plate uptake diminishes and disappears. Normal activity can persist in some areas, such as residual ossification centers in the sternum and the sternomanubrial joint.
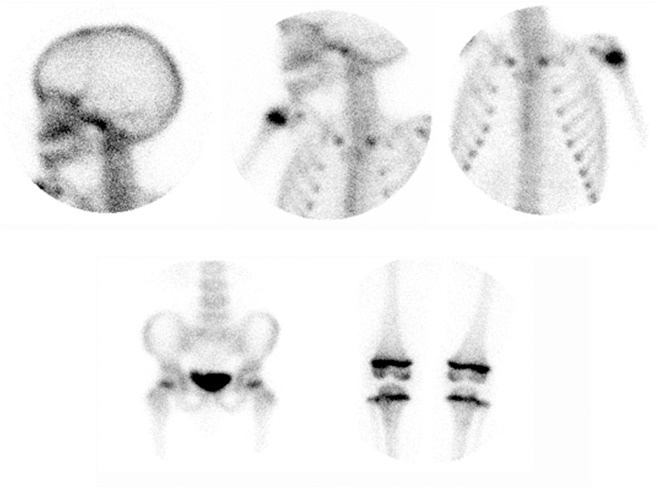
Some bones, such the sacroiliac joints, the iliac wings, or a lordotic spine, normally appear more intense because of their greater density or closer proximity to the camera. Increased uptake bilaterally in the frontal bones of the calvarium may occur from benign hyperostosis frontalis interna. Increased activity, sometimes asymmetrical, is occasionally seen where the sphenoid ridge meets the calvarium along the lateral orbits. The costochondral junction is another common site of benign uptake and is an unlikely location for metastasis unless uptake extends along the rib.
Osteoarthritic changes are routinely seen and usually identifiable by a classic distribution. Arthritis is frequently bilateral and often involves both sides of the joint. The areas typically involved are the spine, knee (particularly the medial compartment), feet, shoulder, wrist (especially at the base of the first metacarpal), ( Fig. 6.10 ). Uptake in the patella may result from chondromalacia and degenerative change. Mild asymmetry has been noted in the shoulders, apparently affected by handedness and use. Of note with F-18 FDG PET, arthritis rarely shows the high levels of uptake seen on bone scan. F-18 sodium fluoride, on the other hand, is often very abnormal in sites of arthritis and other benign lesions ( Fig. 6.11 ).
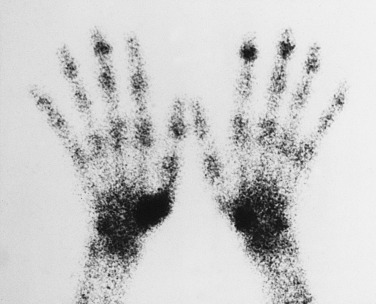
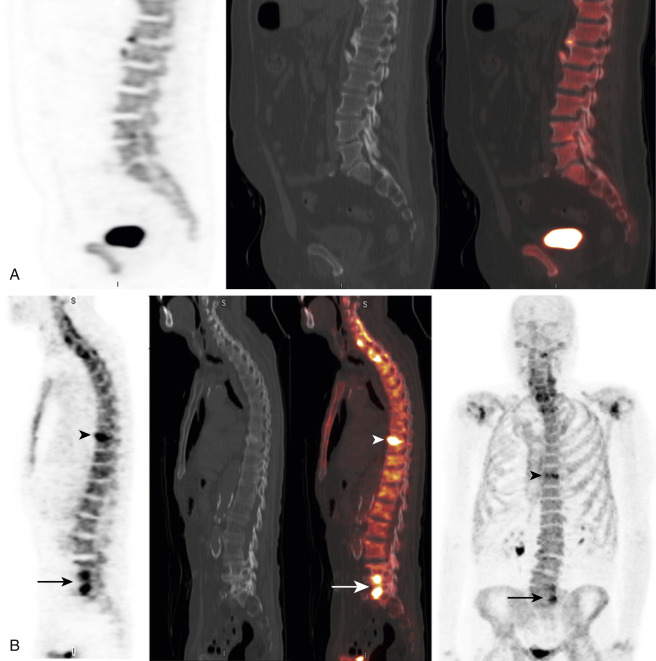
Assessment of spinal uptake frequently requires radiographic correlation with CT (or MR) in addition to SPECT. Some of the changes that can occur from degenerative arthritis include facet hypertrophy, disk space narrowing, osteophyte formation, and Schmorl’s nodes. Osteoporosis may result in classic insufficiency vertebral compression fractures ( Fig. 6.12 ). Abnormal uptake in the vertebra may be seen before radiographic changes occur and may not resolve, particularly in the elderly ( Fig. 6.13 ). Positive uptake has sometimes been used to help determine which patients might obtain symptomatic relief from the injection of bone cement (vertebroplasty). The H-shaped insufficiency fracture occurring in the sacrum ( Fig. 6.14 ) is frequently seen only on scintigraphic studies and not detectable on CT or MR.
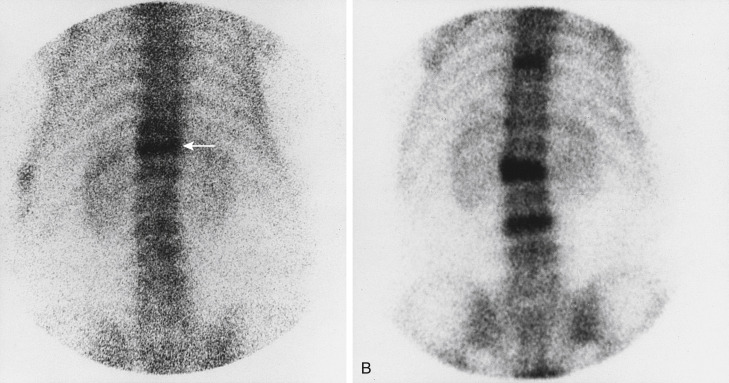
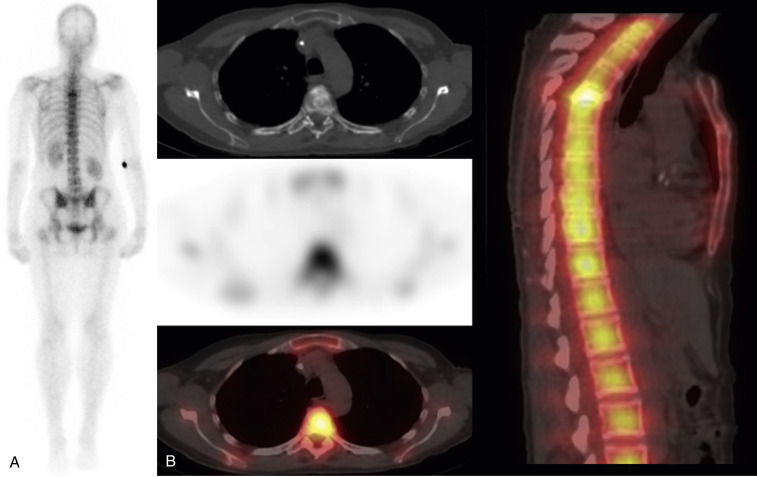
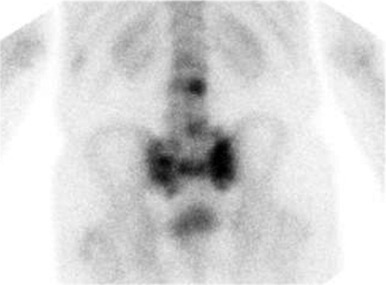
The effects of trauma are often identifiable on bone scan. In the ribs, vertically aligned focal uptake in multiple, often successive ribs is a classic finding ( Fig. 6.15 ). Metastatic lesions, on the other hand, tend to track along the bone, as shown in Fig. 6.5 . When fractures are present, a poorly defined lytic lesion or aggressive periosteal change favors a pathological fracture, whereas regular callous formation is seen in a healing benign posttraumatic fracture. In some cases, the cause of a fracture may be difficult to determine without follow-up.
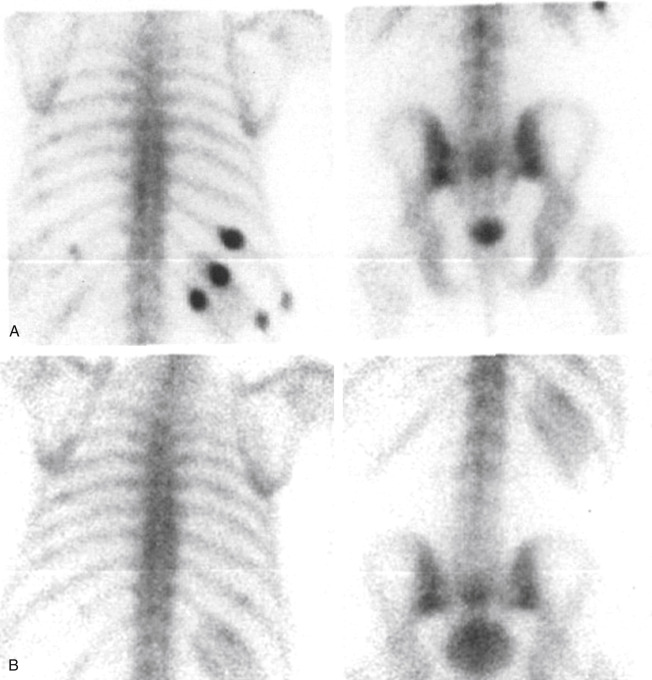
Soft Tissues
The appearance of the soft tissues on bone scan is of critical importance. Normally, the kidneys and bladder show excreted activity from radiotracer in the urine. Abnormal increased or decreased activity must be explained ( Box 6.4 ). Soft tissues (e.g., breast or abdominal fat) and implants attenuate the intensity of the underlying bones. Abnormal increased uptake outside of bone may be subtle or difficult to differentiate from true bone lesions.
Increased Uptake
Nephrotoxic antibiotics
Urinary tract obstruction
Chemotherapy (doxorubicin, vincristine, cyclophosphamide)
Nephrocalcinosis
Hypercalcemia
Radiation nephritis
Acute tubular necrosis
Thalassemia
Decreased Uptake
Renal failure
Superscan
Metastatic disease
Metabolic bone disease
Paget disease
Osteomalacia
Hyperparathyroidism
Myelofibrosis
Nephrectomy
Prolonged delays in imaging
In some instances, abnormal soft tissue uptake may be present from hemorrhage or necrosis, likely as a result of a combination of ossification and agent binding to macromolecules. The effects of recent surgery may be evident, and tumors (primary and metastatic) may be seen ( Figs. 6.16 and 6.17 ). In the right lower chest and upper abdomen, abnormal soft tissue activity may be a result of breast tumor, malignant pleural effusion, or metastatic adenocarcinoma of the breast or colon to the liver. Correlation with CT is helpful to uncover the disease that may be causing Tc-99m MDP accumulation ( Fig. 6.18 ). In addition to the effects of disease, abnormal activity in the liver may be the result of improper radiopharmaceutical preparation resulting in a colloid that is then taken up by the hepatic reticuloendothelial system. Radiopharmaceutical quality control tests can identify these cases.
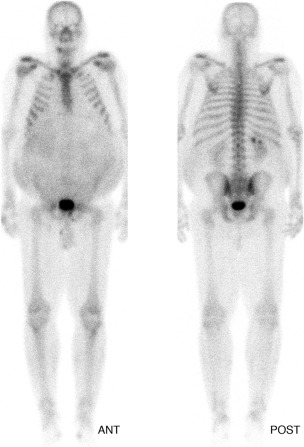
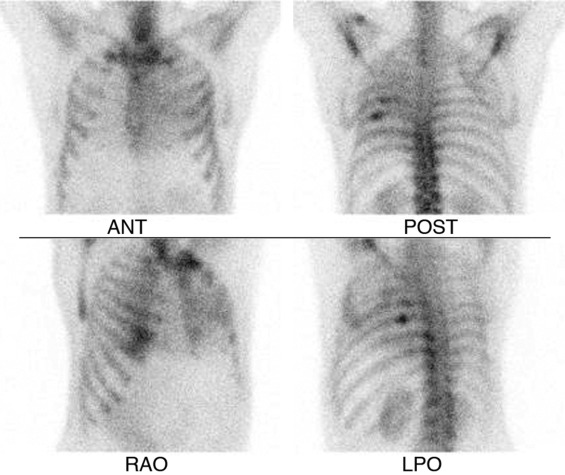
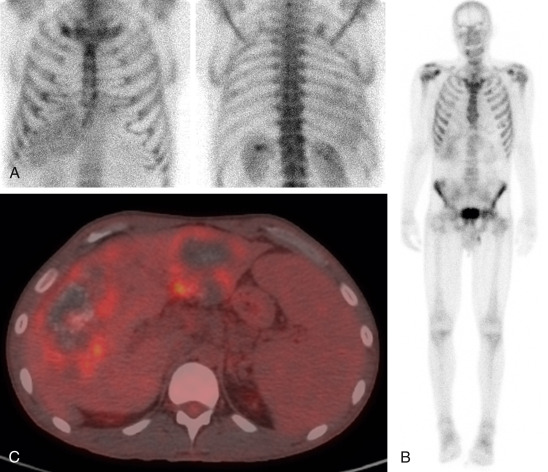
When a three-phase scan technique is used, markedly increased perfusion indicates that the disease or trauma affecting the area is acute because chronic processes cause little or no asymmetry in blood flow, as shown in Fig. 6.4 . Increased blood flow and blood-pooling activity can result from cellulitis, abscess, or synovitis in the soft tissues ( Fig. 6.19 ). However, the changes can also occur as a result of disease in the underlying bone or joint, such as fracture or osteomyelitis.
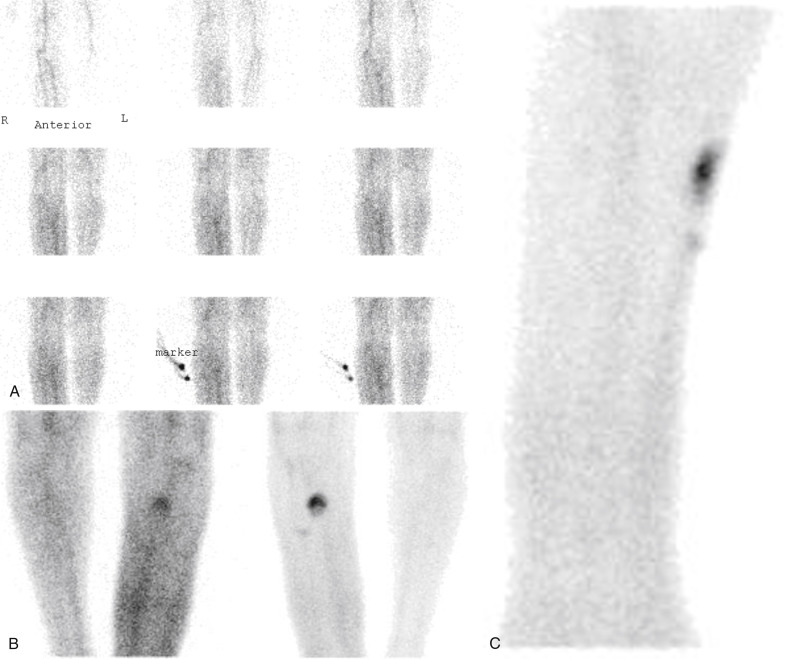
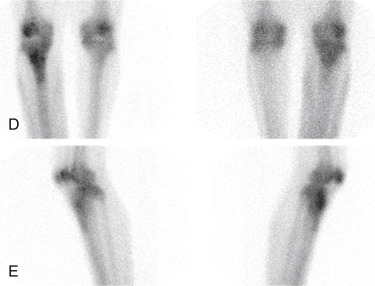
Comparing Different Imaging Methods
Comparing the sensitivity of scintigraphic imaging with traditional modalities, it should be noted that bone scan requires as little as 5% bone turnover for a lesion to be detected, compared with the 50% needed on x-ray. Therefore lesions are often visualized on bone scan but not on x-ray. Bone scan is also usually more sensitive than CT.
MR is more sensitive than planar bone scan and potentially more specific. Its high-resolution images are often able to directly visualize disease-related signal changes in the marrow, and it can optimally evaluate soft tissue structures. However, when SPECT/CT is performed, the accuracy of MR and bone scan for skeletal disease is comparable. It is also difficult for MR to replace bone scan in several situations: obese patients and those who have claustrophobia, difficulty lying on the camera table for lengthy examinations, implanted metallic devices incompatible with the powerful magnetic gradients (e.g., pacemakers), intravenous contrast sensitivity, or renal insufficiency. The whole-body scanning desirable for metastatic disease evaluation is not widely available or practical at this time with MR.
Several small studies have shown that SPECT is more sensitive than planar bone-scan images and better able to localize lesions. The studies have also shown that the use of SPECT/CT improves specificity, leaving fewer indeterminate lesions in the end. As previously noted, images performed with PET are superior to studies from a gamma camera. Compared with bone scan, F-18 NaF has demonstrated higher lesion sensitivity overall, and F-18 FDG PET better detects aggressive, lytic lesions. The resolution of single-photon agents is lower than with PET/CT, but the use of SPECT/CT narrows or eliminates the gap in accuracy between the methods. In some cases, additional tests or short-interval follow-up will be needed to establish a diagnosis, whether the examination is performed with PET/CT or one of the bone-scan techniques.
Clinical Uses of Skeletal Scintigraphy
Metastatic Disease
A significant fraction of patients with known malignancy will develop osseous metastasis . Patients may present with bone pain (50%-80%) and elevated alkaline phosphatase (77%), but these findings are nonspecific. The evaluation of osseous metastatic disease is the most common use of skeletal scintigraphy. Determining whether a bone scan is appropriate depends on factors such as tumor and stage, history of pain, and radiographic abnormalities.
More than 90% of osseous metastases distribute to the red marrow, which is found in the axial skeleton and the proximal portions of the humeri and femurs in adults (as shown in Fig. 6.3 , A ). As the tumor enlarges, the cortex becomes involved. Tc-99m MDP binds to areas of attempted repair, not the tumor itself. Bone scan is said to be up to 95% sensitive, but this sensitivity depends on several factors, such as tumor type and tumor stage.
Scintigraphic Patterns in Metastatic Disease
The scintigraphic patterns encountered in skeletal metastatic disease are summarized in Box 6.5 . Multiple focal lesions distributed randomly in the skeleton provide a high degree of clinical certainty in the diagnosis of metastases. However, other causes can also show multiple areas of uptake ( Box 6.6 ). Often, different features and patterns can help identify these causes. For example, Paget disease may be differentiated from metastasis by a coarse expansion of the bone.
Multiple focal lesions
Central skeleton (i.e., areas with red marrow) skull, spine, pelvis proximal long bones
Distal extremities uncommon but can be seen with lung cancer
Solitary focal lesion
Diffuse involvement (“superscan”)
Cold lesion (photon deficient)
Normal (false negative)
Flare phenomenon post chemotherapy treatment
Hypertrophic osteoarthropathy (formerly hypertrophic pulmonary osteoarthropathy)
Soft tissue lesions
Metastatic disease
Arthritis
Trauma
Osteoporotic insufficiency fractures
Paget disease
Fibrous dysplasia
Infarction (sickle cell disease)
Osteomyelitis
Multiple enchondromas
Metabolic bone diseases
The chance that a solitary lesion is a result of malignancy varies by location ( Table 6.1 ), and some features of primary bone lesions are outlined in Table 6.2 . Very focal uptake in one rib is likely from trauma. Even in a patient with known cancer, it has only a 10% to 20% chance of being malignant, whereas uptake in the central skeleton has a much higher likelihood of being from metastasis. Primary bone tumors, such as osteosarcoma, must be suspected, especially in younger patients, when long-bone involvement is seen. Common benign causes for a solitary lesion include arthritis and trauma. Some benign bone lesions, such as enchondroma, osteoma, fibrous dysplasia, osteomyelitis, and monostotic Paget disease, can also cause solitary abnormalities. Rarely, a benign bone island or a spinal hemangioma will accumulate some Tc-99m MDP. Stability over time can prove a lesion benign, and hemangiomas show a characteristic pattern on CT imaging, with striations or prominent trabecula.
Blastic Metastases: Sites that are predominantly osteoblastic are more easily seen with Tc-99m MDP and F-18 NaF PET ( Fig. 6.20 ). With F-18 FDG PET, blastic lesions show variable activity, but uptake is often very low or at background ( Figs. 6.21 and 6.22 ). In response to treatment, lesions will show decreasing uptake, often occurring along with increasing sclerosis on CT imaging.
Fig. 6.20
Sclerotic metastases usually show increased activity with technetium-99m (Tc-99m) methylene diphosphonate (MDP) and F-18 sodium fluoride (F-18 NaF) positron emission tomography (PET) when active, and this uptake will decrease in response to treatment. The treated lesion will show increasing sclerosis on CT. Care must be taken because uptake may be more intense with sodium fluoride than on bone scan. (A) Marked activity in a sclerotic right iliac metastasis from prostate cancer. (B) Follow-up images 6 months later show that despite some enlargement, once treated, uptake decreases in intensity from partial response. However, multiple new lesions were identified, as seen in the sacrum, from overall disease progression.
Fig. 6.21
Diffuse prostate metastatic disease shows marked technetium-99m (Tc-99m) methylene diphosphonate (MDP) activity (A) on whole-body bone-scan images. (B) Fluorine-18 fluorodeoxyglucose (F-18 FDG) positron emission tomography with CT (PET/CT) performed 2 weeks later shows largely absent activity in the sclerotic lesions, with only a few FDG-avid foci seen. When prostate cancer becomes aggressive and no longer controlled with hormone-blocking medications, then FDG PET becomes useful, showing marked uptake in lesions.
Fig. 6.22
Sagittal images of the T-spine from fluorine-18 fluorodeoxyglucose (F-18 FDG) positron emission tomography with CT (PET/CT) performed after chemotherapy for metastatic colon carcinoma show persistent active disease. Uptake is greater in a mildly sclerotic lesion, and below this, new uptake developed from disease progression in the posterior aspect of a vertebral body and its posterior elements, which are less sclerotic than treated disease anteriorly.
Cold Lesions: Lesions that are aggressive, purely lytic, or completely replaced by tumor may show decreased uptake or appear “cold.” A list of possible causes for cold defects is provided in Box 6.7 . These photon-deficient areas may be difficult to spot because of overlying or adjacent activity, although SPECT and SPECT/CT increase sensitivity. In some cases, Tc-99m MDP bone scan and F-18 FDG PET/CT may be complementary tests, with blastic lesions often better seen with bone scan while F-18 FDG is superior for lytic areas. ( Fig. 6.23 ). With high sensitivity for lytic and blastic lesions, F-18 NaF PET/CT may also be useful in difficult cases. However, false negatives can occur, particularly in small lytic lesions in the spine. Other causes for decreased uptake such as metal attenuation artifact, radiation ports, compromised blood flow early in a pediatric septic joint, or very early infarct or avascular necrosis should be considered in the differential.
Box 6.7
Differential Diagnoses for Cold Lesions on Bone Scan
Metal attenuation artifact
Radiation changes
Barium in bowel
Early osteonecrosis (or avascular necrosis)
Early infarct
Multiple myeloma
Osseous metastases
Renal cell carcinoma
Thyroid cancer
Anaplastic tumors
Neuroblastoma
Breast and lung cancer (often mixed lytic and blastic)
Tumor involvement in marrow
Lymphoma
Leukemia
Benign tumor, cysts
Fig. 6.23
(A) Bone-scan images show a barely perceptible cold lesion in the right ileum (arrow) corresponding to a large soft tissue mass involving bone on (B) fluorine-18 fluorodeoxyglucose (F-18 FDG) positron emission tomography with CT.
Superscan: A potentially problematic scintigraphic pattern is the “superscan” or “beautiful bone scan.” Fairly homogeneous increased activity is seen in the bones, with absent or only faint visualization of the kidneys and bladder (e.g., Fig. 6.3 , B ). The differential diagnosis of the pattern is provided in Box 6.8 , but most commonly, the cause is either diffuse prostate cancer metastases or metabolic change from severe renal failure causing hyperparathyroidism ( Fig. 6.24 ). Confusion from a superscan is a less common interpretive problem than in the past because of improved technology and image quality, and it should be pos sible to discriminate diffuse metastasis. Reviewing the available radiographs on each patient will help prevent mistakes.
Box 6.8
Causes for Superscan Pattern
Fig. 6.24
Severe hyperparathyroidism, most commonly from severe renal failure, can sometimes result in a superscan. (A) Anterior and (B) posterior whole-body images show more advanced changes from secondary hyperparathyroidism. Note the lack of renal cortex or excreted urine. In addition to increased uptake with a smooth appearance in many bones and activity in the skull, activity in the metaphyseal regions around the knees and ankles in this 52-year-old give an appearance that could be confused with images from a child.
Flare Phenomenon: When patients have a good response to chemotherapy, they sometimes experience increased pain, and the bone scan may paradoxically worsen, with a flare of increased activity ( Fig. 6.25 ). If these lesions are followed over 2 to 6 months, CT imaging shows increased sclerosis from an osteoblastic response as the bone begins to heal. Activity should regress by 4 to 6 months after the flare. This phenomenon reinforces the fact that tracer uptake is not in the tumor but rather in the surrounding bone.
Fig. 6.25
Flare phenomena on bone scan. (A) Metastatic lesions in a patient with breast cancer appear to progress during therapy on (B) bone scan 4 months later. However, this does not represent actual worsening disease, and marked improvement is seen (C) on a scan after 6 months. Pelvic CT images obtained at the time of the first bone scan (D) show lytic lesions initially but fairly diffuse sclerosis on a follow-up CT (E) performed after the third bone scan, corresponding to treatment response.
Site | Percentage (%) |
---|---|
Spine and pelvis | 60-70 |
Skull | 40-50 |
Rib | 10-20 |
Sternum (in breast cancer) | 55-75 |
Lesion Type | Most Common locations | Features |
---|---|---|
Multiple myeloma | Rarely confined to one bone; spine is common Areas of hematopoiesis: vertebra, skull, pelvis, chest wall, proximal long bones | Age: >45 years 80% initially have bone involvement: osteoporosis, punched-out lytic lesion, or fracture |
Osteosarcoma | Femur 42% (75% distal femur)Tibia 19% (80% proximal)Humerus 10% (90% proximal) | Age: Bimodal peak: 10-14 years and then >65 years Involves metaphysis May be a secondary cancer in Paget |
Chondrosarcoma | Common: pelvis up to 30%, femur, humerus More unusual: skull base, sacrum | Age: 30-70 years 85%-90% are primary tumor, 10%-15% arising from osteochondromas, enchondroma, fibrous dysplasia |
Ewing sarcoma | Pelvis 26% Femur 20% Tibia 10% Chest wall 16% May occur bones foot, hand, jaw, spine (all <10%) | Age: 10-20 years Involves diaphysis 70% of cases originate in bone |
Paget disease |
|
|
Fibrous dysplasia | Ribs, femur, skull (around 20%-28% each) Can occur any bone | Any age, monostotic; polyostotic usually < 10 years Ground glass, can also appear sclerotic, deformed, expanded |
Enchondroma | Metacarpals, metatarsals, femur, humerus, tibia |
|
Imaging Findings in Specific Cancers
Prostate Carcinoma
Skeletal scintigraphy is generally best for the detection of prostate cancer metastases, and until the introduction of the prostate-specific antigen (PSA) blood test, bone scan was considered the most sensitive technique for detecting bone involvement. Serum alkaline phosphatase measurement detects only half the cases detected by scintigraphy. Radiographs may be normal 30% of the time.
The likelihood of an abnormal scintigram correlates with clinical stage, Gleason score, and PSA level. In early stage I disease, scintigrams demonstrate metastases less than 5% of the time. The incidence increases to 10% in stage II disease and 20% in stage III. In patients with PSA levels less than 10 ng/mL, bone metastases are rarely found (<1% of the time). Even if the risk is lower, scintigraphy is still indicated to evaluate symptomatic patients and suspicious areas seen radiographically. With increasing PSA levels, the chance of detecting metastatic disease increases. In cases where recurrent prostate cancer is a concern, bone scan may be used to supplement the detection of soft tissue involvement performed with F-18 fluciclovine (Axumin). Although F-18 NaF PET may be useful, F-18 FDG is most often not.
Breast Carcinoma
Despite increased screening with mammography, a large number of patients with breast cancer are initially diagnosed with advanced disease. Autopsy studies have shown osseous metastases in 50% to 80% of patients with breast carcinoma. As in prostate cancer, the stage of disease correlates with the incidence of osseous metastases on bone scan: 0.5% in stage I, 2% to 3% in stage II, 8% in stage III, and 13% in stage IV. Bone scans are not generally performed in patients with stage I or II disease.
Skeletal scintigraphy is highly sensitive in breast cancer. Patients may show local invasion of the ribs or sternum or disseminated disease. Although activity in the sternum is most often benign, a high incidence of metastatic disease is seen in patients with breast cancer (>75%-80%). Abnormal soft tissue activity can be seen from tumor or surgery in the breast, in disease that is metastatic to the liver (see Fig. 6.18 , B ), and in malignant pleural effusions. F-18 FDG PET is also useful, particularly for lytic lesions and those in marrow but some blastic lesions may be harder to see.
Lung Carcinoma
Although up to 50% of patients who die from a primary lung cancer have osseous metastasis at autopsy, no complete agreement exists on when to use skeletal scintigraphy. Staging is generally done with CT, surgery (including mediastinoscopy and video-assisted thoracoscopic surgery), and in some cases with F-18 FDG PET/CT. Skeletal scintigraphy is useful in a patient who develops pain during or after treatment.
Interesting patterns of disease may occur on scintigrams in lung cancer. Because these tumors can easily invade the vasculature, arterial metastases are more common. These tumor emboli can reach the distal extremities. Thus appendicular involvement is more common with aggressive lung cancer than cancer of the breast or prostate. Also, increased cortical activity, prominent in the extremities, can be seen in lung cancer as a result of hypertrophic osteoarthropathy ( Figs. 6.26 and 6.27 ). In addition, patients exhibit a range of findings on physical examination, including clubbing of the fingers. Although lung adenocarcinoma is most commonly the cause of this change, other cardiopulmonary etiologies and occasionally hepatic and gastrointestinal disorders can cause similar findings ( Box 6.9 ).
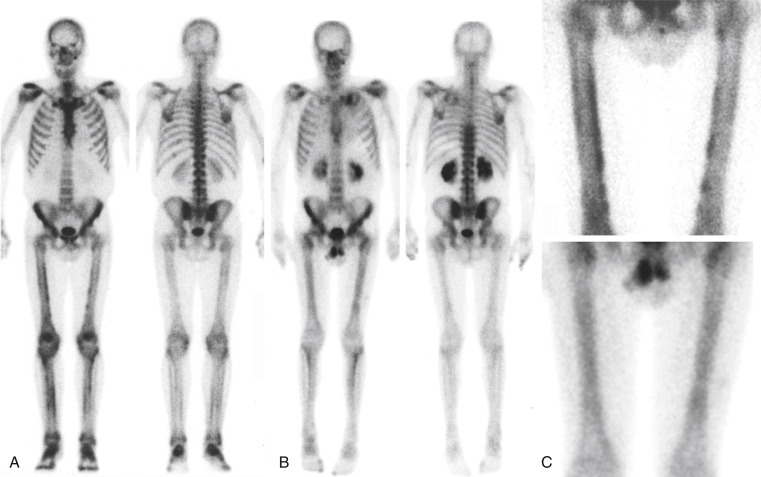
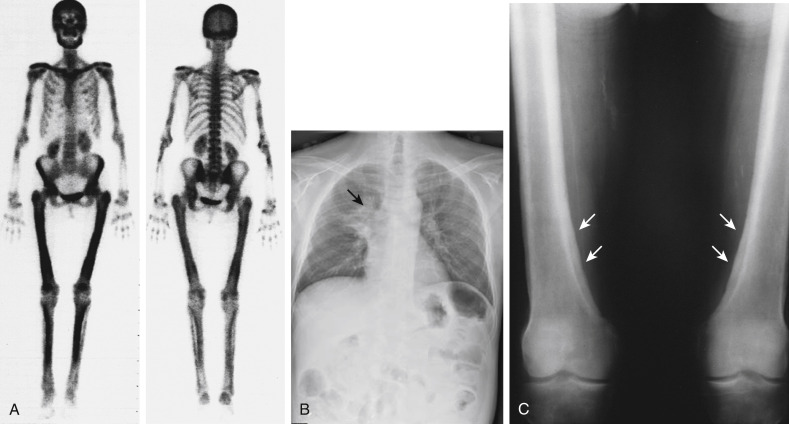
Pulmonary
Adenocarcinoma of lung (up to 53% of cases)
Mesothelioma
Cystic fibrosis
Interstitial lung disease
Cardiac
Cyanotic heart disease
Myoma
Subacute bacterial endocarditis
Aortic graft infection
Hepatic
Cirrhosis
Hepatopulmonary syndrome
Biliary atresia
Bowel
Inflammatory bowel disease
Amebic dysentery
Colonic polyposis
Esophageal cancer
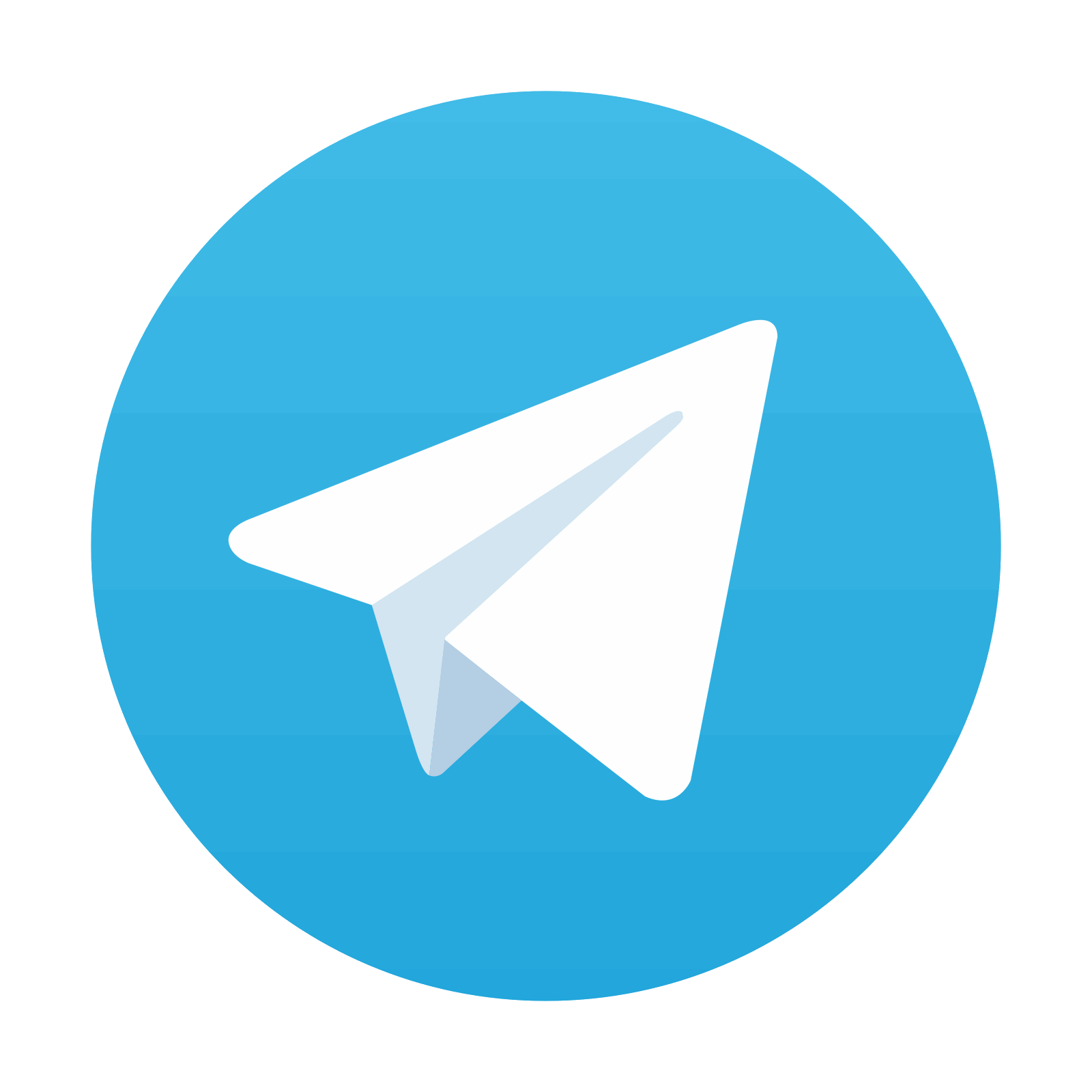
Stay updated, free articles. Join our Telegram channel

Full access? Get Clinical Tree
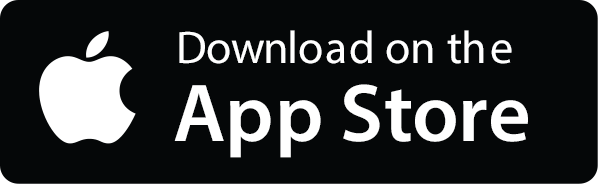
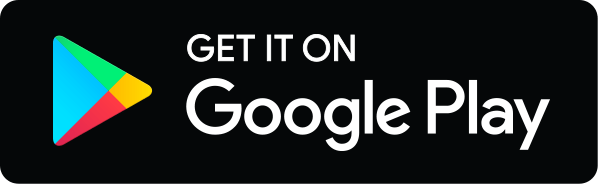
