Fig. 1
Statistical maps refer to the largest clusters (LC) at the source space indicating significant increased activity for the young adults group relative to the older adults group in the IC [(A) (B) (C) (D)], and in the DC (E). Only statistical differences are shown considering the corresponding minimum Monte Carlo p-value (in text). A time axis (in milliseconds) is included to mark the temporal dynamics of neural differences
Specifically, time-modulated activations in posterior-frontal regions were increased in young compared to older adults. Additionally, young adults exhibited greater posterior-frontal activations for the interrupting compared to the distracting condition. These results suggest that age-related deficits in inhibitory mechanisms were associated with under-recruitment in posterior-frontal regions. On the other hand, the absence of differential interference-related neural recruitments suggests that both types of interference affect the elderly equally. In a subsequent study, Solesio-Jofre et al. (2012) investigated age-related changes in brain activity during recognition and after the presentation of two types of RI, interruptions and distractions, by using the same DPA task for faces as in the previous study. These investigators, examined the temporal dynamics of brain magnetic activity during the first 1000 ms after the onset of each correct response at recognition. MEG results revealed the presence of differential age-related neural patterns. Specifically, time-modulated activations in temporo-occipital and superior parietal regions were higher in young adults compared to older adults for the interrupting condition. They suggested that age-related deficits in inhibitory mechanisms may be associated with neural under-recruitment in a highly interfering task. Finally, García-Pacios et al. (2013) reported on activity related to the encoding process of this DPA task. They found increased activity over prefrontal regions for elderly participants compared to young adults. This result indicates that elderly subjects needed higher executive control resources during encoding in order to achieve adequate performance on the task.
Altogether, this suggests that the Inhibitory deficit hypothesis provides an appropriate theoretical framework in which to understand age-related declines in memory function. Different studies show that MEG is an ideal tool to test the temporal dynamics of brain activity related to such cognitive deficits. The emerging MEG literature confirms that age-related under-recruitment, together with lower performance on memory tasks, reflect deficient inhibitory mechanisms. Specifically, deficient inhibitory mechanisms result from top-down control processing that regulates goal-directed behaviour and the suppression of irrelevant information when different responses compete for limited working memory resources.
2.2 The Neural Correlates of Age-related Inhibitory Deficits: The Frontal Deficit Hypothesis
Extensive literature suggests that cognitive decline in aging is accompanied by degeneration of tissue and functional reserves (Cabeza et al. 2002; Reuter-Lorenz and Lusting 2005). Converging evidence comes from lesion, anatomical and functional neuroimaging studies. The former show that lesions in dorsolateral prefrontal regions are associated with poorer performance on working memory, executive attention, and inhibitory control (Grasby et al. 1994; D’ Esposito et al. 1995; Muller and Knight 2006). Different anatomical approaches have reported linear declines in gray matter in prefrontal regions starting during young adulthood (Gogtay et al. 2004; Hutton et al. 2009). Declines in white matter tracts connecting prefrontal and temporal lobes present the same linear pattern starting from 45 years (Bartzokis et al. 2001; Sullivan et al. 2001). Both gray and white matter declines with advancing age are supportive of the disconnection hypothesis (Geschwind 1965; O’Sullivan et al. 2001) that proposes that functional disruptions of large-scale brain networks account for the cognitive decline across the lifespan. Finally, most functional neuroimaging studies of aging have investigated the relationships between behaviour and brain activation patterns comparing young and older adults. It has been commonly reported that during the performance of memory recognition tasks, older adults show bilateral prefrontal activity while young adults show more lateralized prefrontal activity (Cabeza et al. 2002).
Although inconclusive, these findings showing age-related over-recruitment in the frontal lobes, together with those showing under-recruitment by Solesio-Jofre et al. (2011, 2012) are consistent with the frontal deficit hypothesis. It postulates that cognitive deficits in older adults are primarily caused by the anatomical and functional deterioration of the frontal lobes (e.g., Moscovitch and Winocur 1995; West 1996). Two main mechanismsfor age-related over-recruitment of the PFC have been proposed. On one hand, the compensation model (Madden et al. 1999; Reuter-Lorenz et al. 2000; Cabeza et al. 2002; Grady et al. 2006) postulates that older adults engage some brain areas, particularly the frontal lobes, above the level seen in younger adults to compensate for reduced activity in visual processing regions (PASA, Davis et al. 2008 see above for a detailed explanation). On the other hand, the dedifferentiation model also seems to characterize the increased bilateral prefrontal activity found in older adults (Colcombe et al. 2005; Aine et al. 2010; Park et al. 2010; see introduction section). One MEG study in support of the PASA Model was conducted by Aine et al. (2006). These investigators examined spatial working memory in young and older participants with a delayed-match-to-sample (DMS) task. Correlations between MEG responses and behavioural performance suggested that two different strategies were used by the different age groups while maintaining the same overall performance levels; young adults relied on posterior brain regions while the elderly relied on inferior frontal and supramarginal regions.
To determine the aging effects of brain activity on behaviour and differentiate between compensation and dedifferentiation, Grady (2008) summarized the most relevant findings to date and described four distinctive patterns in order to select the most appropriate explanation. First, older adults show more brain activity but the same performance level as younger adults as evidence of inefficient neural processing. Second, older adults reveal equal or greater brain activity but worse performance which is evidence of inefficient neural processing. Third, older adults recruit a brain region that is not active in young adults but they show equivalent performance, as evidence of compensation or dedifferentiation. Fourth, older adults recruit a brain region that is not active in young adults and it is positively correlated with performance in older adults and not in the young as evidence of compensation.
However, in a recent review (2011), Aine et al. brilliantly suggest an alternative explanation for such under-recruitment and over-recruitment discrepancies commonly shown in brain aging research. Prefrontal differences observed between young and older adults do not necessarily refer to aging impairments but brain maturation as a dynamic process that might improve cognitive abilities through later decades in life. It emphasizes the importance of brain flexibility and development throughout the lifespan in order to differentiate between adaptive changes that occur with age in opposition to brain dysfunction. There is growing interest in the neurosciences on exploring brain development from infancy through childhood, adulthood and aging (Pascual-Leone 2011). It is important to understand the processes underlying aging and how do they shape brain structure and function until death. It is crucial to conduct longitudinal lifespan studies or to, at least, include middle-aged subjects in cross-sectional studies in order to detect the continuous changes from the first to the last stages in life. Additionally, Aine et al. (2011) also highlight the necessity to better define aging and differentiate healthy successful aging from normal and pathological aging with objective measures, instead of subjective self-reports. It will enable the identification of additional risk factors that may lead to cognitive decline.
Finally, it is important to consider that the abovementioned discrepancies across studies regarding age-related neural differences (under-recruitment or over-recruitment) and their relationship with behaviour (compensation, or dedifferentiation) may be affected by different factors (Aine et al. 2011) such as the differences in experimental designs (Daselaar et al. 2003); analysis procedures (ROIs vs. whole brain approaches); and the heterogeneous variability of different measures across lifespan (e.g., white matter tracts in DTI studies, Aine et al. 2010; neurovascular coupling in fMRI studies, Kannurpati et al. 2011). In this regard, resting state functional connectivity emerges as a powerful tool that overcomes some of the limitations derived from task-related experiments. We describe the basic notions and current developments in the use of MEG in resting state studies in the following section.
2.3 Future Directions in the Study of Normal Aging with MEG: Resting State Functional Connectivity
Resting state functional connectivity is a useful tool to investigate the large-scale functional organization of the human brain. This method is based on the discovery that functionally related brain areas have correlated signal oscillations in low frequency ranges, something that was first described (Biswal et al. 1995; Fox et al. 2005) for blood oxygen level-dependent (BOLD) fluctuations measured with fMRI. The advantages of resting state recordings include the ease in acquiring data without any complicated task design, which makes resting state recordings ideal for children, elders, and patients.
Resting state has been used to explore the default activity of different brain networks involved in motor (Biswal et al. 1995), language (Hampson et al. 2002), sensory (De Luca et al. 2005), memory (Greicius et al. 2003), attention (Fox et al. 2006) and reading (Koyama et al. 2010) systems. Most of the studies in the field have measured the integrity of the large-scale system that involves frontal and posterior brain regions (Shulman et al. 1997; Mazoyer et al. 2001; Andrews-Hanna et al. 2007). This is commonly referred to as the default network (DMN, Raichle et al. 2001) which is associated with internally directed mental states, including memory and executive functions, during rest (Greicius et al. 2003; Fransson 2005; Vincent et al. 2006) and characterized by coherent neuronal oscillations (<0.1 Hz). During high demanding cognitive tasks, the DMN is deactivated and a task-positive network (TPN) is activated. Both the DMN and TPN can be considered elements of a single default network with anti-correlated components (Fox et al. 2005). Specifically, a set of frontal and parietal cortical regions routinely exhibit task-related activity increases, whereas a different set of regions including posterior cingulate, medial and lateral parietal, and medial prefrontal cortex routinely exhibit activity decreases. Extensive literature (Park et al. 2004; Gazzaley et al. 2005; Andrews-Hanna et al. 2007) has shown that cognitive decline in normal aging arises from functional disruptions in the large-scale brain systems, especially between anterior and posterior components of the DMN.
The study of age-related changes in the DMN by means of MEG is an emerging approach with great advantages. Resting state MEG extends and complements resting state fMRI (R-fMRI) by providing high temporal resolution that covers major bands of oscillatory brain activity (Schlee et al. 2012). Additionally, MEG offers a useful way to measure connectivity between brain regions with a direct measure of brain activity, that is, the magnetic fields associated with electrophysiological brain activity (Brooks et al. 2011a). A recent study by Schlee et al. (2012), exploring aging effects within the DMN with MEG, found significant age-related alterations of functional resting-state connectivity. Specifically, they found reduced information input into the posterior cingulum/precuneus region together with enhanced information flow to the medial temporal lobe. The authors concluded that resting state functional connectivity in the elderly is driven by attention to internal processes rather than attention to external stimulation and this is associated with reduced performance in cognitive tasks. In another study, Schlee et al. (2012) focused on large-scale DMN organization across the lifespan. They found that slow frequencies were associated with larger networks compared to higher frequencies. In addition, decreases in visual memory and visuo-constructive functions were associated with an age-dependent enhancement of functional connectivity in both temporal lobes. It led them to conclude the usefulness of resting MEG recordings as a measure of the brain’s baseline activity of functional networks.
In general, the utility of MEG to investigate different resting state networks has been shown in recent papers; de Pasquale et al. (2010) showed a correlation between resting state temporal MEG signals in the DMN and the TPN or dorsal attention network (DAN). Liu et al. (2010) examined correlations between oscillatory power modulations at the sensor level showing that significant correlations could be measured across hemispheres. Brookes (2011b) used seed-based correlation in conjunction with beamformer spatial filtering methods to show interhemispheric motor cortex connectivity in source space.
Altogether, these reports provide evidence that results from resting MEG replicate those from fMRI, suggesting that MEG is a powerful tool in the study of age-related changes of resting state functional connectivity. It is also important to emphasize that a better understanding of the age-related changes in resting state networks may provide information on the neural substrates underlying the inevitable functional decline in advanced aging and help in the early diagnosis and therapy of neurodegenerative disorders (Wang et al. 2012).
3 MEG in Pathological Aging
3.1 Evoked Fields in the AD Continuum
3.1.1 Preconscious Auditory/Somatosensory Processing and Sensory Memory
In a very elegant series of studies, Pekkonen and his colleagues were the first to use MEG to show impaired profiles of magnetic brain activity at early stages of auditory processing in AD. For example, they recorded auditory evoked magnetic fields (AEFs) elicited by monaurally presented tone stimuli from ten healthy young and ten elderly participants (Pekkonen et al. 1995). These AEFs are the result of averaged brain signals and provide information aboput the polarity (“P” for positive or “N” for negative) and latency (expresed in miliseconds after the stimulus onset). As an example P50 m will express a positive component with a latency around 50 ms after the stimulus onset. The “m” is indicating their magnetic origin to differentiate it from the EEG P50. Pekkonen et al. found that the amplitude of the P50 m component in primary auditory cortex was larger in older participants. Regarding the N100 m response, interhemispheric latency difference (ipsilateral peak latency minus contralateral peak latency) increased as a function of age. The authors concluded that early auditory processing in the ipsilateral hemisphere is progressively delayed with increasing age. This study established a normative profile for subsequent studies in AD. Thus, Pekkonen et al. (1996) used similar methodology to record AEFs in AD patients and healthy elders. Peak latencies for P50m and N100m responses were significantly longer in AD patients in the ipsilateral, but not in the contralateral auditory cortex. This finding was replicated in a subsequent study with a larger sample of patients (Pekkonen et al. 1999). No inter-group differences in amplitude were found for N100m or P50m. Notably, scores on standardized language tests correlated with N100m latency recorded in the left (presumably dominant for language functions) hemisphere in the AD group.
To demonstrate that these profiles were related to a cholinergic system dysfunction, Pekkonen et al. (2001a, 2005) injected 0.3 mg of scopolamine (a cholinergic antagonist) prior to the recording session in healthy young (Pekkonen et al. 2001a) and older adults (Pekkonen et al. 2005). The scopolamine effect was associated with increased P50 amplitude in young subjects and a delayed latency of the P50m and N100m in response to tone stimuli in healthy elderly subjects. Another set of studies—using the Mismatch Negativity (MMN) paradigm—indicated that neurophysiological processes associated with sensory memory may be impaired in normal aging as well as in AD. This response takes place within the first 200 ms or so after stimulus onset and originates within the auditory cortex on the supratemporal plane. MMNm was significantly delayed in the left hemisphere ipsilaterally to the stimulated ear in AD patients (Pekkonen et al. 2001b). A subsequent report, however, indicates that MMNm was not affected by scopolamine injection in older healthy adults (Pekkonen et al. 2005). Recently, Cheng et al. (2012) assessed the M50 and MMNm responses during a passive oddball paradigm. The results showed larger cortical activation of standard-evoked M50 in AD patients compared to young and elderly controls. In contrast, the MMNm latency was longer in AD patients than in elderly controls. Thus, this report complements the scopolamine findings by Pekkonen et al., by providing support for the increased power of the signal found at early stages of processing as an indicator of disruption. Finally, Osipova et al. (2006), using a steady-state paradigm, showed that auditory stimulation at 40 Hz generates increased steady-state response in AD patients.
All these findings suggest that alterations in brain function in AD may take place at much more basic stages of stimulus processing. Together with the link established between early magnetic responses and cholinergic function, opens up the possibility of assessing the efficacy of new drugs, by evaluating their ability to modify the delay of this early response in AD patients.
Finally, Stephen et al. (2010) reported on differences in the early somatosensory magnetic response. MCI patients exhibited a larger amplitude response than healthy elders or patients with AD. These investigators also reported a relationship between neuropsychological test results and the amplitude of primary somotosensory responses. This report generalizes the idea that multiple sensory systems can be affected at early stages of the disease.
3.1.2 Memory Task
In a series of studies, comparing AD and age-matched healthy participants, using a modified version of the Sternberg paradigm (a continuous recognition memory task), Maestu et al. prospectively determined biomagnetic profiles that differentiated AD from healthy controls (Maestu et al. 2001) and elderly patients with major depression (Maestu et al. 2004). In these studies, patients with AD showed lower numbers of activity sources over the left parieto-temporal regions between 400 and 800 ms after stimulus onset. These findings correlated with performance on neuropsychological tests; the lower the number of dipoles for the posterior regions, the lower the score on the Minimental State Examination. In addition, the spatio-temporal profiles of neuromagnetic activity correlated with the degree of atrophy in MTL (Maestu et al. 2003), and with the metabolic changes detected by MR-Spectroscopy (Maestu et al. 2005). Thus, the greater the atrophy in MTL, the lower the number of activity sources over parieto-temporal regions in that particular time window. Furthermore, when an ROI for the analysis of MR-Spectroscopy was placed over these parieto-temporal regions, AD patients showed an increased ratio between myoinositol/N-acetyl-aspartate, indicating an increased glial proliferation and a loss of neurons over these regions. This biochemical finding correlates, in this sample of participants, with the loss of activity sources in these brain regions. In fact, the combination of biochemical and biomagnetic profiles was a better predictor of scores on the neuropsychological tests than either of these approaches separately. This series of studies made possible a description of particular MEG profiles that differentiated between AD and healthy elders. More importantly, the comparison between atrophy of the MTL and the biochemical profiles provided new insights about the interpretation of the MEG profiles. Thus, it seems as though the reduction of biomagnetic activity over the parieto-temporal regions could be due to a disconnection between the MTL and these neocortical regions, as well as due to a loss of neurons, as suggested by MR-spectroscopy.
Along similar lines, Walla et al. (2005) conducted a study in which participants were given an incidental verbal learning task (i.e., they were not instructed to learn the items), under two conditions (shallow and deep processing of the stimuli). After a short delay, their memory of previously presented items was tested, using a recognition memory paradigm. Recordings obtained over posterior brain regions revealed clear differences between correctly recognized repeated words (hits) and correctly rejected new words (correct rejections) in healthy elderly participants in the time window between 300 and 400 ms after stimulus onset. AD patients did not show differences between conditions for any brain region.
In two subsequent studies, Maestú et al. evaluated whether biomagnetic profiles could differentiate between MCI patients and controls. Contrary to their initial expectations, the MCI patients showed higher activity over the ventral pathway between 600 and 900 ms (Maestu et al. 2008). The ventral pathway consists of the ventral prefrontal region, the MTL, the mid-temporal gyrus and the inferior parietal lobe. This pathway is well known for its involvement in recognition memory; thus, hyperactivation of this pathway appears to be related to a compensatory mechanism in the initial stages of the disease. A similar interpretation was developed by Dickerson et al. (2005) in an fMRI study where they describe an increased hemodynamic response in the MTL of MCI patients, in comparison to a control group. In a subsequent study, five control participants that developed MCI after two years of follow-up showed lower numbers of activity sources in the medial temporal lobe (Maestu et al. 2006). These results indicate that brain activity is reduced at a very preliminary stage of the disease. They also imply that when elders progress to MCI their activity is increased as a compensatory mechanism for the progressive lack of efficiency of the memory networks. It is of great interest to note that an independent study found similar results using MEG. Püregger et al. (2003) recorded brain magnetic activity from 10 MCI patients and 10 controls during a shallow (nonsemantic) and deep (semantic) word encoding task. Between 250 and 450 ms after stimulus onset, brain magnetic activity associated with nonsemantic and semantic word encoding differed significantly mainly over left frontal and left temporal regions. MCI patients showed increased activity during shallow encoding as compared to deep encoding. Controls did not show such a profile of activation. The authors interpreted this hyperactivation as a compensatory mechanism. Thus, the increased profile of activation seems to be a general finding that could constitute a biomarker for the early stages of the disease that can be found as well in elders with subjective memory complaints (SMC) as reported by Maestú et al. (2011a). However, in this last study MCI patients and SMC did not show differences between them suggesting that similar physiological mechanisms may underlie SMC and MCI. Later we shall see how the use of functional connectivity measures allows one to detect clear differences between these two groups. Aine et al. (2010) showed that enhanced activity in a group of MCI/AD patients correlated with lower IQs and poorer performance on verbal/visual memory tests. Thus, correlations could indicate that increasing brain activity does not indicate better functioning.
Two recent studies have evaluated memory related activity in the frequency domain using a modified version of Sternberg’s paradigm in AD (Kurimoto et al. 2012) and in MCI (Aurtenetxe et al., 2013) patients. Kurimoto et al. (2012) found significant differences in the beta and gamma frequency bands. Patients with AD showed reduced beta event-related desynchronization (ERD) in the right central area compared to controls. Aurtenetxe et al. (2013) showed increased theta, lower beta reduction and decreased alpha and gamma power in frontal, temporal and parietal areas of MCI subjects, during early and late latencies. Their results point towards a dual pattern of activity (increase and decrease of frequency power) in MCI patients which is specific to certain time windows, frequency bands and brain regions. It may be that these results represent two neurophysiological sides of MCI. These two papers indicate that, along the AD process, a common neurophysiological disruption exists—the lack of beta band desynchronization (an electrophysiological phenomenon associated with successful memory performance). This neurophysiological sign is already present at the MCI stage and seems to be underlying early cognitive impairment. However, at the MCI stage, theta band increases and predicts memory test performance. This phenomenon does not seem to be present at the AD stage, indicating that compensatory mechanisms are present for a limited period of time.
3.2 Resting State Activity
Along with the approach for studying profiles of brain magnetic activity during memory tasks there is also an approach for looking at the spontaneous brain activity (resting state). The studies focusing on resting state examine brain activity mainly in the frequency domain. In a seminal study, Berendse et al. (2000) showed that the absolute low frequency magnetic power was significantly increased in fronto-central regions. Conversely, high frequency power values were decreased over the occipital and temporal areas.
In a series of studies, Fernandez et al. reported specific profiles of increased and decreased oscillatory activity in AD patients. Fernandez et al. (2002) showed an increase of delta and theta activity over the temporal and parietal lobes bilaterally. Notably, increased slow wave activity in these regions was associated with reduced performance in neuropsychological and daily living measures. In parallel with the memory studies carried out by Maestú et al. (2003, 2005), Fernandez et al. characterized the spontaneous findings in relation to MR-volumetry and MR-spectroscopy. A relationship between the presence of focal low-frequency magnetic activity and left hippocampal volume was found (Fernandez et al. 2003). When MEG and MR-volumetry were combined, left medial temporal lobe volume and the left temporal theta activity, correctly classified 87.1 % of the participants in their respective diagnostic groups. Furthermore, myoInositol/N-acetyl-aspartate rate scores in combination with MEG slow magnetic activity classified AD patients and controls with 90 % sensitivity and 100 % specificity (Fernandez et al. 2005). Osipova et al. (2005) found that the lower alpha rhythm was enhanced over the right temporal lobe in AD patients, while in age-matched controls alpha sources were found near the parieto-occipital sulcus. To evaluate whether this effect is due to a cholinergic deafferentation, it is useful to consider some classical studies (Riekkinen et al. 1991) in which a significant correlation between AChE activity of the cerebrospinal fluid (CSF) and delta power in patients with AD was detected. This correlation was further supported by EEG and MEG studies where scopolamine infusions generated changes in background activity, including reduced alpha and beta and increased delta and theta, that mirrored those found in AD patients (Osipova et al. 2003; Kikuchi et al. 2000). Thus, it might be proposed that low-frequency activity may function as an early indicator of neural dysfunction in AD and cognitive impairment. Besga et al. (2010
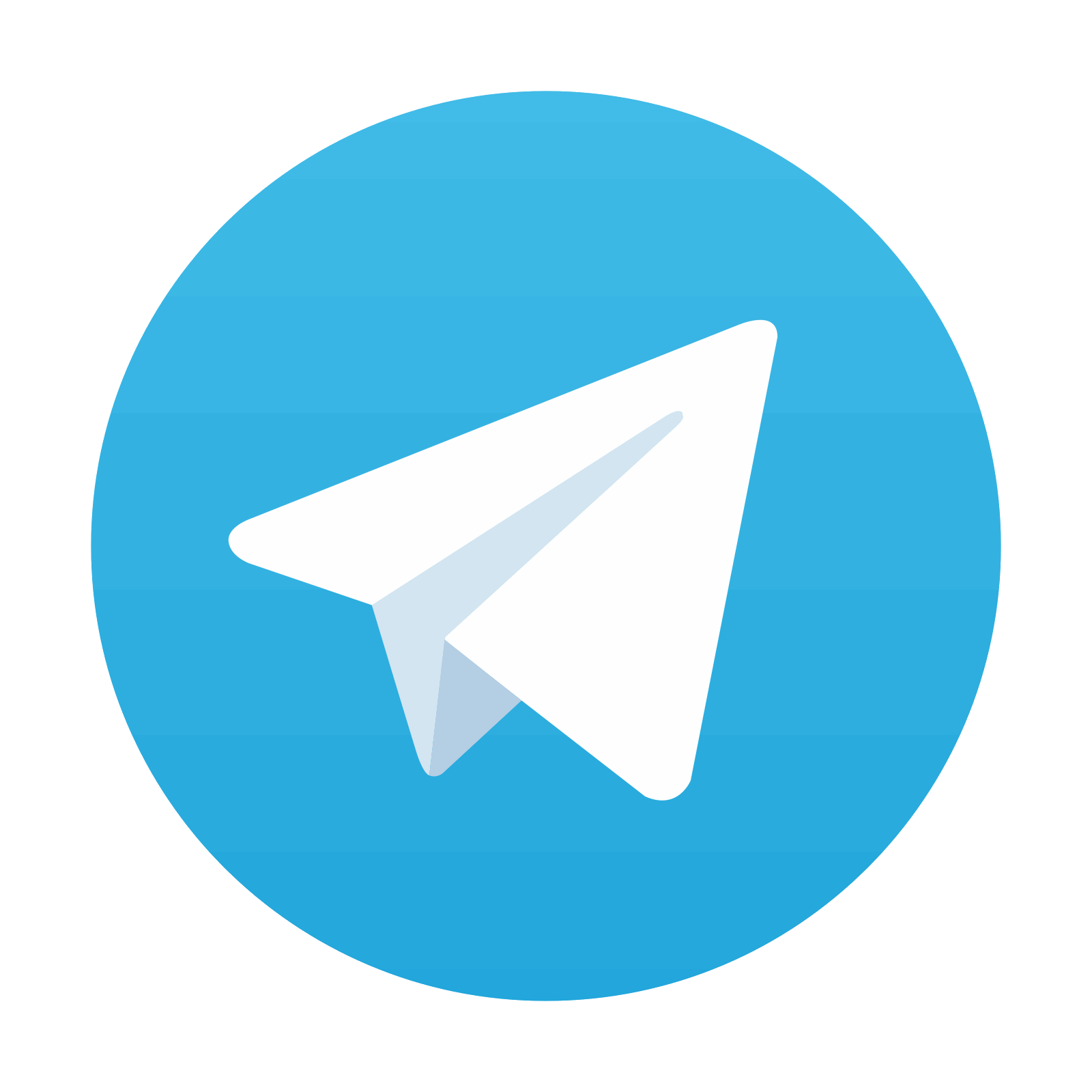
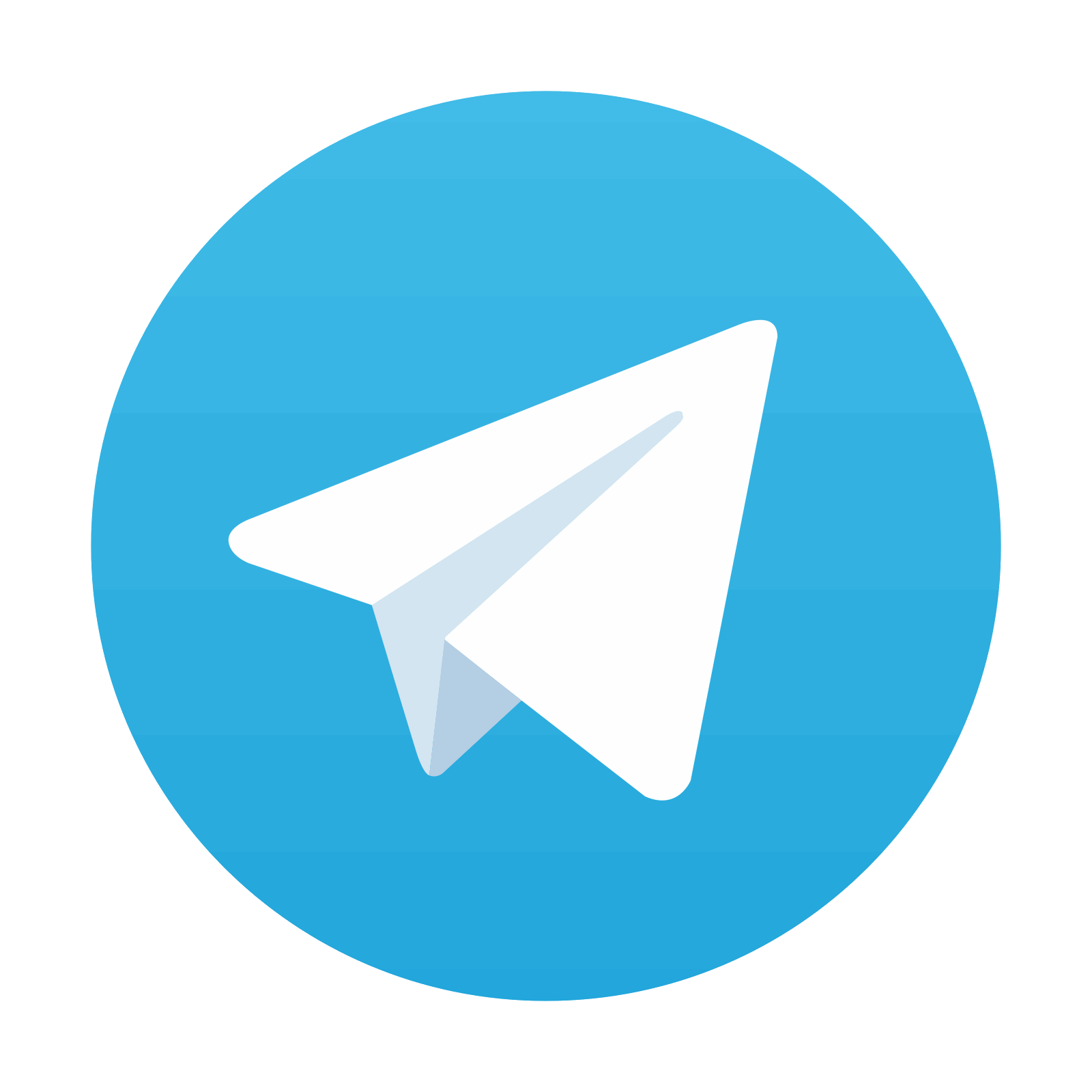
Stay updated, free articles. Join our Telegram channel

Full access? Get Clinical Tree
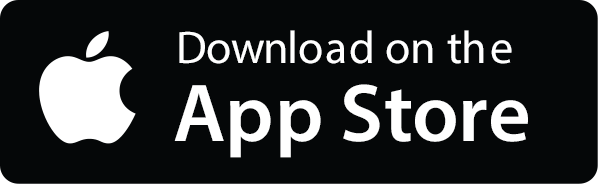
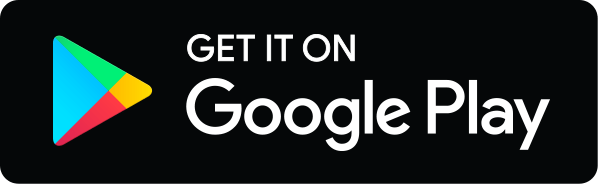