Physical Therapy
Physical therapists utilize several energy-based therapies in their arsenal to treat specific injuries or illnesses, including ultrasound, laser, electrical nerve stimulation, or even hydrotherapy. These therapies including ultrasound are used to accelerate and enhance tissue repair and regeneration. As indicated above, there are two mechanisms of action of therapeutic ultrasound: thermal and nonthermal. Heat generated by the absorption of ultrasound energy in tissue dilates blood vessels, increasing the volume of a multitude of circulating factors for tissue repair. In addition, heat has an analgesic effect on nerves. Tissue composition also determines the magnitude of thermal effect; protein has a higher absorption coefficient than fat and water. Thus, fatty tissue and blood (high water and/or fat content) do not experience as much of a thermal effect as ligament and tendon (higher protein, lower water content). Although cartilage and bone have the highest protein content, acoustic impedance mismatching results in a higher proportion of ultrasound energy being reflected than absorbed. The best absorbing tissues in terms of clinical practice are those with high collagen content: ligament, tendon, fascia, joint capsule, and scar tissue. Nonthermal mechanisms, such as acoustic streaming and cavitation, effect changes in membrane permeability and hence the movement of essential ions and nutrients into and out of the cell. Thus, it can be said to achieve an upregulation of cellular activity triggered by ultrasound energy.
Although there is conflicting clinical evidence to support the use of ultrasound to facilitate the treatment of injured ligaments, tendons, and joints, and to alleviate symptoms associated with excessive scar tissue, it is widely used today for such purposes. Indeed, much of the conviction surrounding the efficacy of ultrasound in treating soft tissue injury and pain originates from in vitro studies, which have not been duplicated in vivo (Baker et al, 2001). Within the most recent decade more clinically oriented papers have attested to the utility of therapeutic ultrasound in conditions such as osteoarthritis, carpal tunnel syndrome, myofascial pain syndrome, among others. Despite its widespread application in clinical use, there remains little standardization in terms of duration of therapy, frequency and intensity range, and number of treatments. The most common frequency range found on physical therapy ultrasound units is 0.7 to 3.3 MHz.
Bone Healing
There have been many published reports of the benefit of ultrasound on bone regeneration in all types of bone injury including fresh fractures, nonunion and delayed union fractures, and for enhanced ossification in distraction osteo-genesis. Fracture healing is a complex process that can be divided into three phases: reactive, reparative, and remodeling. The reactive phase is characterized by hemostasis and inflammation; the reparative phase heralds the formation of a fracture callus, where primitive bone and collagen cells begin the process of rebuilding across the fracture, and in the remodeling phase, the final constitution of mature compact bone is gradually replaced along the fracture. The first phase is relatively brief, and the cells of the reparative phase are seen within days of bone injury; complete healing is only seen 3 to 5 years after injury. It is the hyperactive first two phases that are the most sensitive to ultrasound therapies. Low-intensity pulsed ultrasound or LIPUS was approved by the FDA in 1994 for stimulating bone growth in a number of applications including fracture healing and dental applications. It generally utilizes 1.5-MHz frequency pulses, with a pulse width of 200 μs, duty cycle of 1 kHz, at an intensity of 30 mW/cm2, 20 min/day.
Observations of ultrasound stimulation of bone healing are based on in vitro and animal models, and include increased secretion of cytokines (IL-1β) and angiogenesis-inducing factors (IL-8, b-FGF, VEGF), leading to cell proliferation, collagen production, bone formation, and angiogenesis. The biophysical principle of this cellular recruitment by ultrasound, however, remains unproven. Hypotheses include: a thermal effect from the absorption of energy in tissues, which may increase collagenase activity; the strain effect from the acoustic energy that may stimulate periosteal bone formation; and cavitation that increases protein and collagen synthesis, but the exact mechanism is yet unknown.
LIPUS has the advantage of being a safe, low-energy, low-cost and efficacious method for encouraging more rapid healing of bony fractures. Although there is a lack of rigorous randomized controlled studies, this shortcoming is shared with all other treatments of fractures. Some may hail the necessary duration of treatment as lengthy, in the range of 3 to 6 months, although this likely represents an acceleration of the natural healing process.
Sonophoresis
Transdermal drug delivery offers the advantage of improved patient comfort and thus compliance over needle injection, and avoids the first-pass metabolism observed with many oral drugs. The composition of human skin, however, limits the usefulness of this technique to a few low-molecular weight (<500 Da) lipophilic drugs, and in small doses. In particular, the stratum corneum, with its matrix of corneo-cytes embedded in lipid bilayers, is a highly impermeable membrane. Insonifying skin with acoustic waves in the frequency range 20 to 100 kHz has been shown to increase its permeability to macromolecules, a process known as sonophoresis. Initially devised for local delivery of drugs, it is now being investigated for systemic drug delivery.
Low-frequency sonophoresis can be divided into two types: simultaneous sonophoresis and pretreatment. As is implied, simultaneous sonophoresis involves administering the drug in synchrony with the application of ultrasound. This technique increases skin’s permeability to the molecule by enhancing diffusion via structural alterations of the skin (defects in the stratum corneum), and by inducing convective solvent flow by cavitation. This effect can be quantified by measuring the skin’s electrical resistivity or impedance, which decreases in the presence of ultrasound.
Clinically sonophoresis has been used to enhance the local delivery of topical local anesthestic, by decreasing dramatically the application time needed for effect. Animal studies are ongoing with respect to systemic delivery of macromolecules, including proteins such as insulin and heparin, or immunogens such as tetanus toxoid. Sonophoresis is generally a safe process, if maintained within certain parameters of frequency, intensity, duty cycle, and application time. Specifically, intensities greater than 4 W/cm2 have been shown to result in epidermal detachment and dermal necrosis. Continuous monitoring of skin’s impedance can be employed to ensure safety during use.
Sonoporation and Gene Therapy
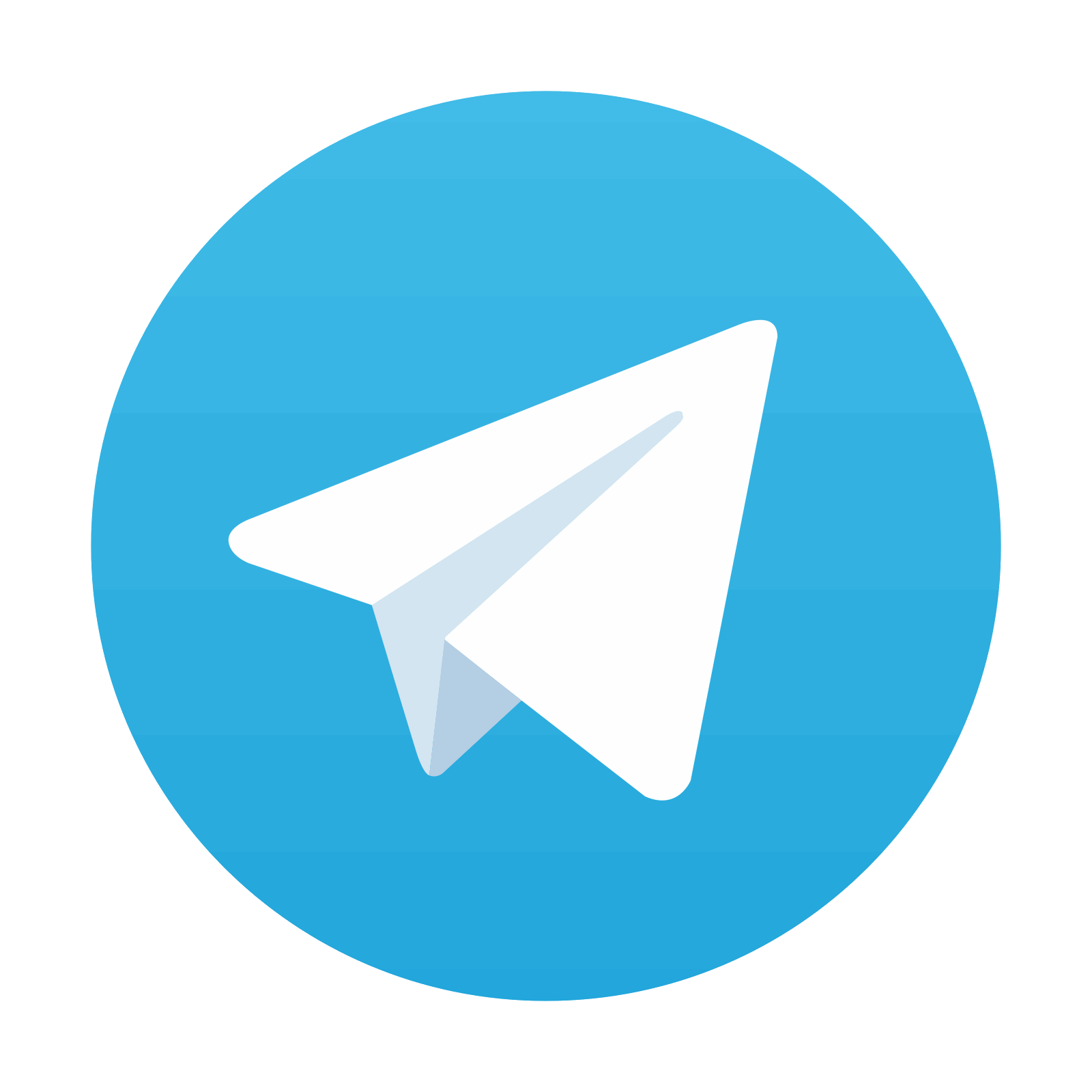
Stay updated, free articles. Join our Telegram channel

Full access? Get Clinical Tree
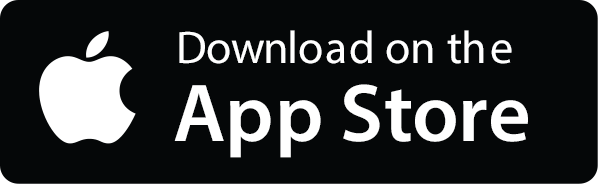
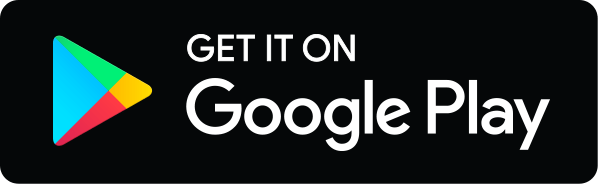