Fig. 7.1
Effect of transcatheter aortic valve replacement (TAVR) on survival in patients with severe symptomatic aortic stenosis in the randomized controlled PARTNER trial. (a) In inoperable patients (PARTNER trial cohort B), TAVR results in significantly improved 2-year survival, compared to standard therapy, including balloon valvuloplasty (Reprinted from Makkar et al. [3], with permission from the Massachusetts Medical Society). (b) In operable patients (PARTNER trial cohort A), 2-year survival is similar between TAVR and surgical aortic valve replacement (Reprinted from Kodali et al. [16], with permission from the Massachusetts Medical Society)
Risk has been arbitrarily defined according to the Society of Thoracic Surgeons (STS) database scoring system, with low risk as STS <4, intermediate-risk STS 4–8, and high-risk STS >8 [6]. If TAVR offers favorable data compared to SAVR in the intermediate-risk group, the referral base for this technology will increase significantly, and its true potential will be realized. There remain important caveats to the expansion of TAVR beyond such cohorts. Low surgical risk patients are still best treated by SAVR until evidence accumulates for intermediate-risk patients. This is for several reasons. Importantly, the durability of TAVR has only been demonstrated to 3 years [3, 7] (Fig. 7.2), with the longest surviving patients with TAVR only followed to around 6 years, whereas several studies have demonstrated long-term durability of surgical bioprostheses as long as 15 years and beyond [8]. Moreover, the safety of the procedure and ongoing refinements in the technology have a growing evidence base in this regard, but it will take time to address all the shortcomings of the early generation devices and procedures; these will be discussed in the next section of this chapter.
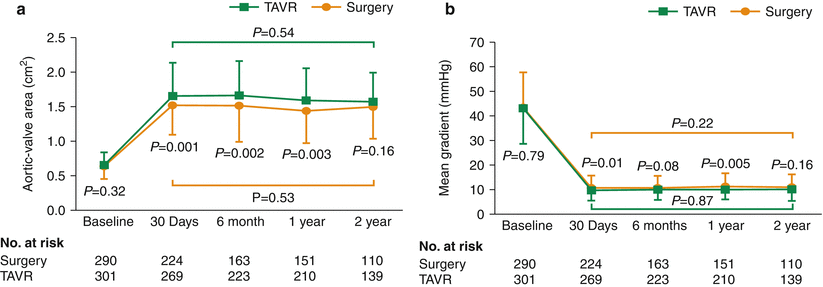
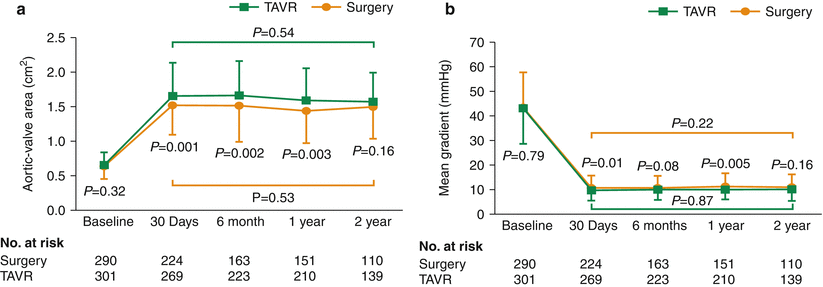
Fig. 7.2
In the randomized controlled PARTNER trial, transcatheter aortic valve replacement resulted in a sustained improvement in aortic valve area (a) and mean gradient (b), up to 2 years [2]
Conversely, although procedures are often technically feasible in the patients with prohibitive surgical risk, they may be clinically futile in some patients, in whom TAVR should not be performed. There will be an important focus in identifying such patients, so that an unnecessary and costly procedure may be avoided. There already exists some data in this regard. In a subset analysis cohort B of the PARTNER trial, patients at the highest surgical risk by STS score (STS ≥15) had no survival benefit with TAVR in comparison to medical therapy [3] (Fig. 7.3). Imaging may offer some insight into this group of patients; for instance, novel MRI techniques can quantify cardiac fibrosis reliably [9] and could identify patients with AS and advanced fibrosis that may not benefit from TAVR. At the other end of the spectrum, imaging may help stratify risk of cardiac structural deterioration in asymptomatic patients with severe AS, whose treatment remains controversial [10]. TAVR, with its less invasive nature than open surgery, may one day offer a viable treatment option in such patients.
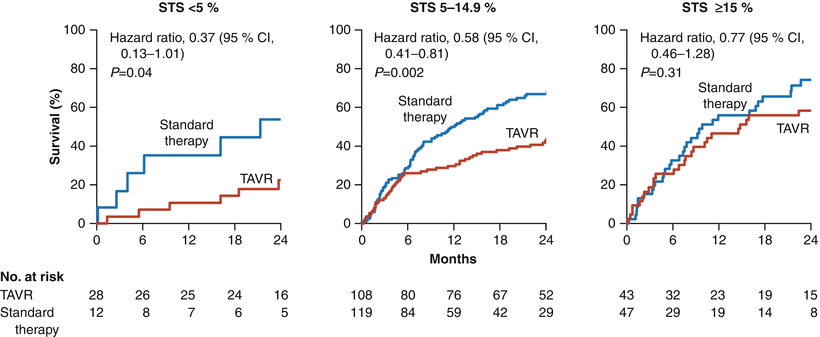
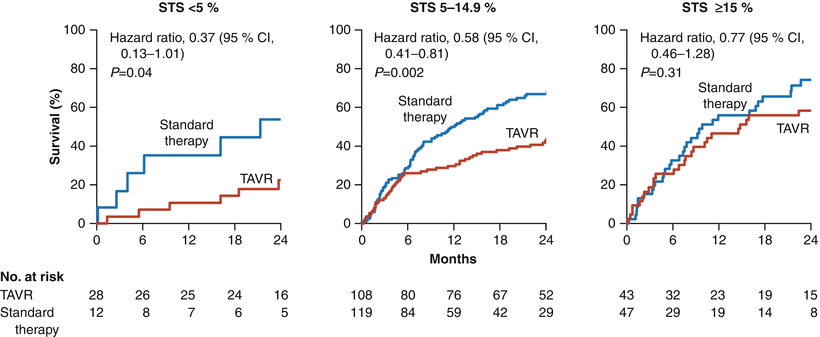
Fig. 7.3
Stratification of 2-year mortality according to STS score (<5 %, 5–14.9 %, or ≥15 %) in inoperable patients with severe symptomatic aortic stenosis in the randomized, controlled PARTNER trial. The survival benefit of transcatheter aortic valve replacement, in comparison to standard therapy, diminishes with a higher STS score, with no survival benefit from TAVR in patients with STS score ≥15 % [1]
Novel Indications for TAVR
TAVR also offers promise in the treatment of patients for conditions other than native aortic valve stenosis. The failing surgical bioprosthesis is an important and exciting new indication for TAVR [11, 12] (Fig. 7.4), potentially limiting the risk of redo operation. Data is currently accumulating in the form of single-center and multicentric registries, but a randomized trial is merited to provide definitive evidence supporting this strategy. There is also increasing technical data regarding how best to select a particular TAVR for a specific failing bioprosthesis, which patients and bioprostheses can be treated in a straightforward manner and which may pose increased risks, such as coronary occlusion [13].
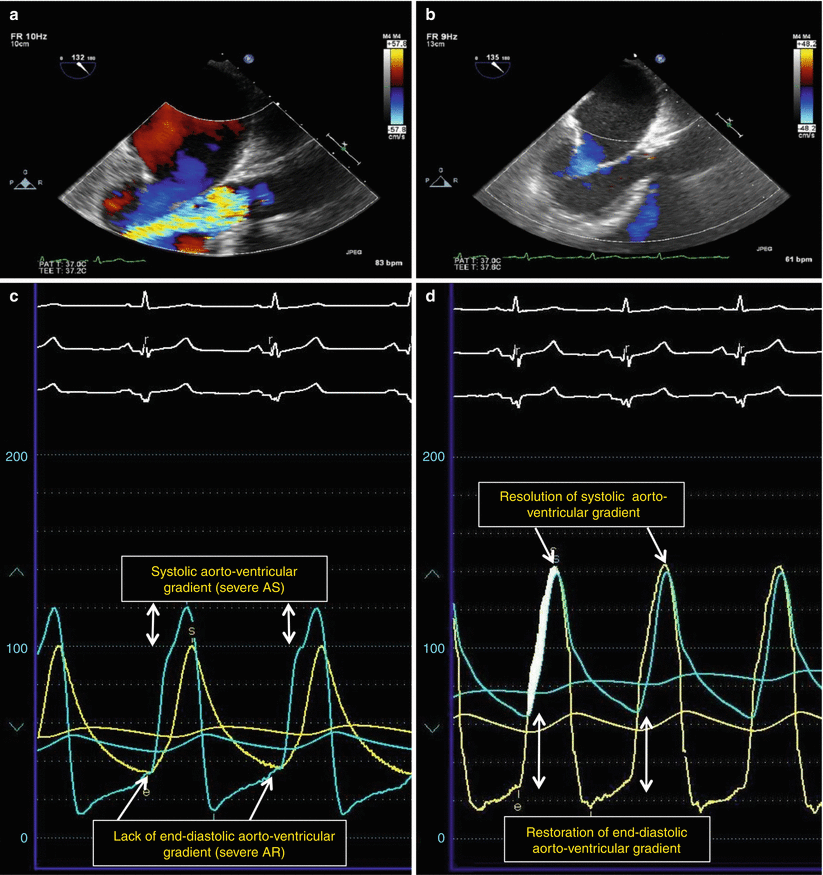
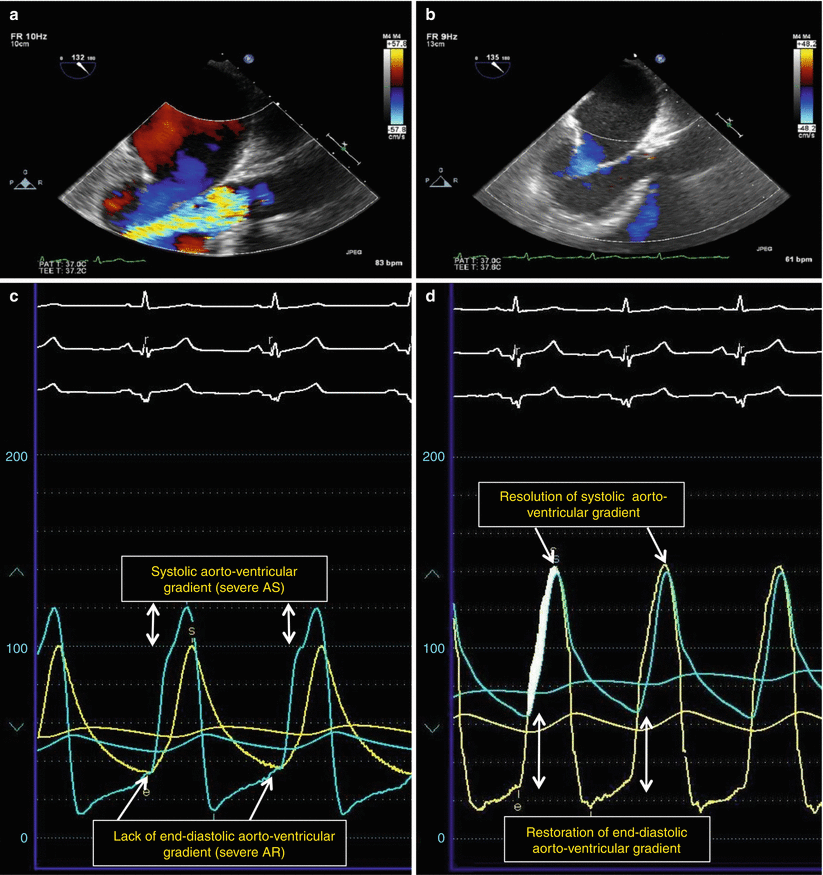
Fig. 7.4
Valve-in-valve implantation of Edwards-SAPIEN valve by transcatheter aortic valve replacement (TAVR) for degenerative bioprosthetic aortic valve stenosis (AS) with regurgitation (AR). (a, b) Echocardiographic findings: Severe bioprosthetic valve degenerative aortic stenosis and aortic regurgitation (a) resolved after valve-in-valve implantation (b). (c, d) Hemodynamic findings: resolution of systolic aorto-ventricular gradient and restoration of end-diastolic aorto-ventricular gradient after valve-in-valve implantation
Native aortic regurgitation is also an exciting new indication for TAVR and presents new challenges [14]. For instance, the use of a balloon expandable device is not feasible as the presence of leaflets without substantial calcification offers much less of an anchor. The vast majority of next-generation TAVR devices comprise self-expanding designs that will be more likely to address this clinical problem. Anchoring a transcatheter bioprosthesis to a native aortic valve in the absence of stenosis will also demand a more rigorous attention to multimodality imaging, ascertaining the qualitative and quantitative aspects of the aortic valvar complex. New challenges may arise; for instance, in stenotic valves leaflet mobility is restricted, with a low risk of coronary occlusion presented only by the most bulky leaflets or in cases of great proximity of the coronary ostia to the aortic annulus. In contrast, in predominant native aortic valve regurgitation, the leaflets are hypermobile and may come close to or even reach the level of the coronary ostia in systole. Indeed, advanced multimodality imaging may draw on lessons for new TAVR indications from cases already performed and provides ongoing data for the safety and efficacy of TAVR for all potential indications.
Limiting the Complications and Improving the Safety and Efficacy of TAVR
Although a lot has been learnt from the early experience of TAVR, several complications that limit the safety and efficacy of this technology are an important focus for future study. Many of these will be addressed in later chapters of this book but the near-term future evidence required for the principal issues of concern will be discussed here.
Paravalvular Regurgitation
Paravalvular AR is an important complication post-TAVR. It is more than moderate in 10 % of cases and has been shown consistently to be associated with increased mortality [15–17]. Two-year follow-up of patients in cohort A of the PARTNER study seemed to suggest that lower degrees of PV AR, even mild PV AR, are also associated with increased mortality [16]. This may be attributable to difficulties in PV AR assessment and underestimation of severity by TEE. An important study recently demonstrated that a transcatheter hemodynamic assessment of PV AR severity (Fig. 7.5) offered mortality prediction of incremental prognostic value to echocardiographic assessment [18]. Further studies are merited as to whether this should be the gold standard for the quantification of this complication. There is a fundamental need for more evidence for optimal imaging for the prevention and assessment of this complication. Recently, contrast cross-sectional CT has been shown to predict paravalvular AR much more reliably than 2D TEE [19, 20] (Fig. 7.6). However, due to renal impairment, many patients undergoing TAVR cannot receive the contrast required for accurate cross-sectional CT assessment of the aortic annulus. Although MRI and 3D TEE offer considerable potential in this regard, evidence of their reliability to the standard of contrast CT is lacking at this time.
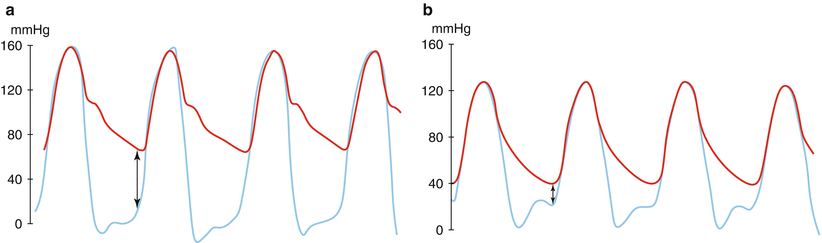
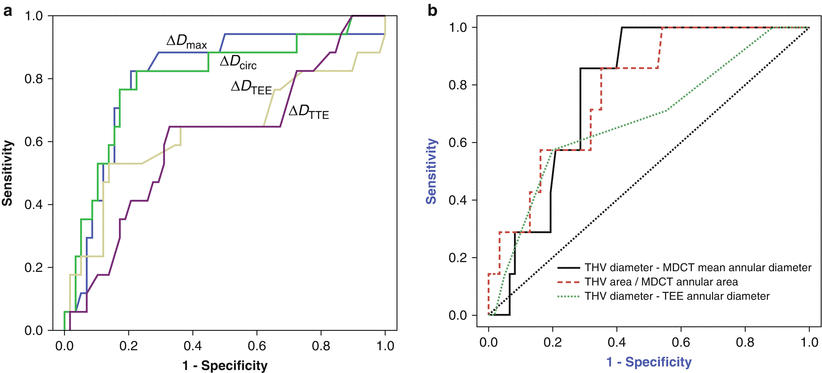
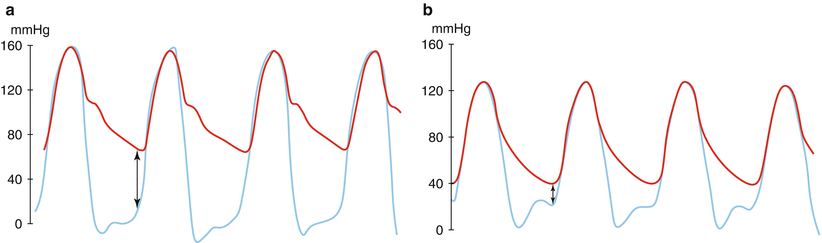
Fig. 7.5
Calculation of aortic regurgitation (AR) index. AR Index is calculated by measuring left ventricular end-diastolic pressure (LVEDP) (blue line) and diastolic blood pressure (DBP) in the aorta (red line) simultaneously. (a) Patient without periprosthetic AR. AR index = ([65 − 10]/160) × 100 = 34.4. (b) Patient with moderate periprosthetic AR. AR index = ([40 − 20]/130) × 100 = 15.4 (Reprinted from Sinning et al. [18], with permission from Elsevier Inc)
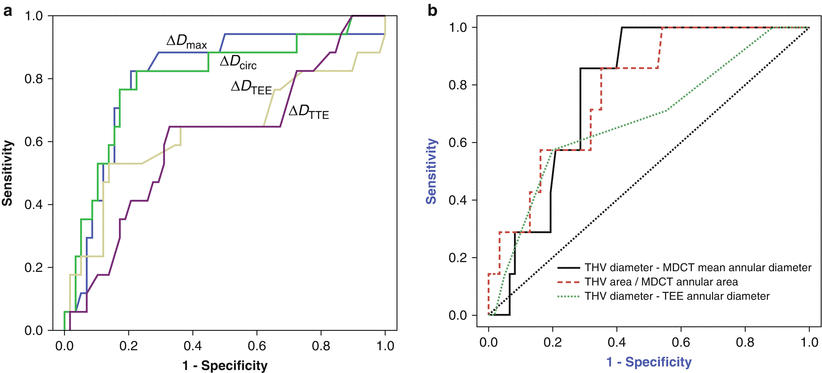
Fig. 7.6
Comparison of cross-sectional CT and transesophageal echocardiography (TEE) for predicting paravalvular aortic regurgitation (AR) after transcatheter aortic valve replacement (TAVR) using area under the receiver-operating characteristic curves method. (a) The cross-sectional CT-derived parameters (ΔD max and ΔD circ) have greater discriminatory value for predicting paravalvular AR after TAVR than TEE-derived parameters (ΔD TEE(max) and ΔTEE(circ)) (Reprinted from Jilaihawi et al. [19], with permission from Elsevier Inc). (b) The MDCT-derived parameters (MDCT mean annular diameter and MDCT annular area) have greater discriminatory value for predicting paravalvular AR after TAVR than TEE-derived parameters (TEE annular diameter) (Reprinted from Willson et al. [20], with permission from Elsevier Inc.). Abbreviations: THV transcatheter heart valve. ΔD max, (CT-derived non-orthogonal true maximum annular diameter) − (THV size); ΔD circ, [(CT-derived perimeter of the traced polygon)/π] − (THV size); ΔD TEE(max), (TEE-derived non-orthogonal true maximum annular diameter) − (THV size); ΔTEE(circ), [(TEE-derived perimeter of the traced polygon)/π] − (THV size)
Stroke
Stroke is an important and much feared complication of both transcatheter and surgical aortic valve replacements. The PARTNER IA study demonstrated a higher incidence of stroke after TAVR than surgery; differences were small but significant early post-procedure but not significant at 1- and 2-year follow-up [4]. Detailed and objective-specialized neurological assessment is thus an integral part of the PARTNER IIA study, mandatory before and after every TAVR and SAVR procedure. Proponents of the transapical approach initially attributed many neuroembolic events with the transfemoral approach to the requirement to negotiate an often atheromatous aortic arch [21]. Subsequent data has shown no difference in either clinical stroke [22] or imaging evidence of neuroembolic events by diffusion-weighted MRI [23]. A detailed analysis of strokes in the PARTNER IIB study showed the majority of post-TAVR embolic stroke events to be periprocedural with late stroke often attributable to hemorrhage (Fig. 7.7) and falls [3]. Important studies investigating the timing of these periprocedural events have shown them to be most marked at the time of valve deployment [24], which points to the main source being the valve leaflets. This supports the need for embolic protection devices.
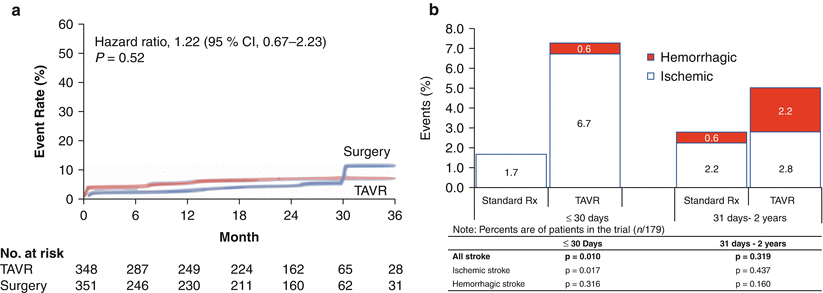
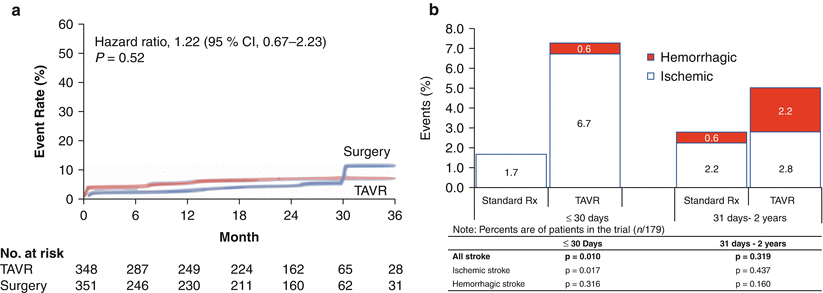
Fig. 7.7
Comparison of transcatheter aortic valve replacement (TAVR) with surgical aortic valve replacement (SAVR) and standard therapy. (a) The stroke rates at 2 years were not statistically different between TAVR and SAVR in the operable cohort in the PARTNER trial [2]. (b) The stroke rates in the inoperable cohort in the PARTNER trial were higher with TAVR in comparison with standard therapy, both at 30 days and 2 years. The excess strokes in the TAVR group in the first 30 days were attributable to a greater number of ischemic strokes in that group. Beyond 30 days and up to 2 years, the excess of strokes was attributable to hemorrhagic events [1]
There are at least three embolic protection devices customized to TAVR (Fig. 7.8). Two are CE marked and under ongoing evaluation in Europe and Canada. The first to be approved in Europe, in May 2010, was the Embrella Embolic Deflector [25], which is inserted by right radial approach, consists of two panels that open in a clam-like configuration, and acts as a deflective shield covering the right innominate and left carotid ostia. Importantly, it does not collect debris but prevents cerebral embolization by covering the ostia of the great arch vessels. Gerckens et al. used the device in 20 TAVR patients [26]—with 18 available for final analysis—and achieved coverage of the innominate artery and the left common carotid artery in all patients; 60 % of the patients also received additional coverage of the left subclavian artery. Post-procedure MRI in 15 of the patients found new cerebral lesions in 11/15 subjects, with an average of 4.36 lesions per patient, comparing favorably with studies of patients undergoing TAVR without embolic protection. Like Embrella, SMT is also an embolic deflector device. It has been evaluated in a small feasibility study, reported by Stella et al. [26]: 15 patients received the SMT device during TAVR. One patient suffered a transient ischemic attack (TIA) at 4 days following the procedure, and one suffered a stroke after 5 days. MRI showed a 50 % reduction in new cerebral lesions compared to 25 % in control patients. The CE mark study started in January 2012.
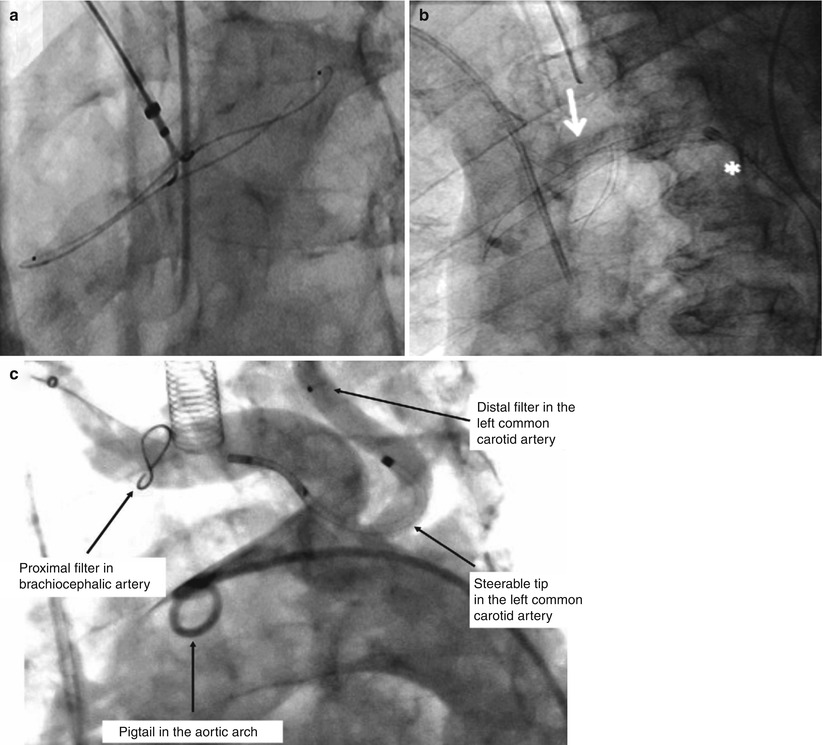
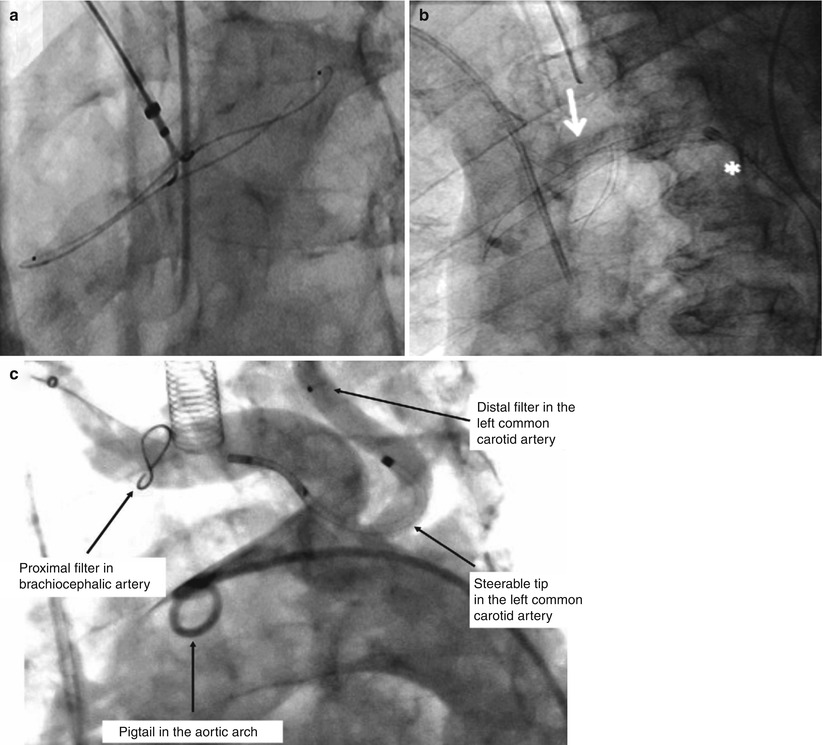
Fig. 7.8
Embolic protection devices for transcatheter aortic valve replacement (TAVR). (a) Embrella Embolic Deflector System (Embrella Cardiovascular, Inc., Wayne, Pennsylvania) (Reprinted from Nietlispach et al. [25], with permission from Elsevier Inc.). (b) SMT Embolic Deflection Device (SMT Medical Technologies, Herzliya, Israel) (Reprinted from Onsea et al. [40], with permission from Europa Digital & Publishing). (c) Claret Dual Filter System (Claret Medical, Santa Rosa, CA) (Reprinted from Naber et al. [41], with permission from Europa Digital & Publishing)
The Montage System is manufactured by Claret Medical and achieved CE mark approval in October 2011. It is inserted by unilateral 6 Fr radial or brachial access and facilitates insertion of a filter in both right innominate and left carotid arteries. Naber et al. reported its use in 40 TAVR patients [26]: although there were no procedural TIAs or minor or major strokes, within 30 days of the procedure, one patient suffered a minor stroke, two suffered major strokes, and six patients died (all non-device related). Although the latter device has the largest clinical experience to date, further studies are required to establish the efficacy of all of these devices, with a fundamental need for randomized studies. Given the relatively low incidence of clinical stroke, DW-MRI will be an important surrogate outcome, although it appears to detect many clinically silent events that remain of unclear significance.
Access-Related Complications
The transfemoral approach, when feasible, is the preferred access approach for TAVR. Both improved imaging of the peripheral vasculature and careful case selection for transfemoral cases have been associated with a marked reduction in peripheral vascular complications over time [27] and will be discussed in later chapters. Technological advances have resulted in further reductions in the profile of TAVR devices, which have also been associated with a reduction in vascular complications [28]. Improved peripheral vascular techniques have facilitated a truly percutaneous approach with pre-closure of the femoral access site and the avoidance of the need for cutdown in the majority of cases [29]. The contingency planning required for bail-out maneuvers in the event of vascular complication have also, most likely, improved outcomes.
However, there remain a significant proportion of patients who, due to peripheral vascular disease or small vasculature, cannot safely undergo the transfemoral approach at this time. It is in these patients that the optimal access approach is yet to be determined. Although the transapical approach was the first alternative approach [30], transaortic [31] and transaxillary [32] are now promising alternatives. Each of the 3 has relative merits. The latter two approaches demand retrograde crossing of the aortic valve but avoid disruption of the left ventricular apex. The transaortic approach cannot be performed in a true porcelain aorta. The transaxillary approach requires axillary vasculature of adequate dimension, which is often observed in larger patients with peripheral vascular disease but not smaller patients. Recently, a propensity-matched study demonstrated similar outcomes of transaxillary and transfemoral TAVR. A head-to-head randomized study of the transaortic and transapical approaches is also merited to provide further evidence required for decision making for the optimal approach in a particular patient.
Evidence for Novel TAVR Devices
The landscape of available devices for TAVR is dramatically changing. From only 2 first-generation devices, several new prostheses are emerging, some from the original companies (Edwards Lifesciences and Medtronic CoreValve) but many more from start-up companies (Fig. 7.9). Those that have entered the clinical arena, and the evidence supporting them, as well as the future evidence required will be discussed here.
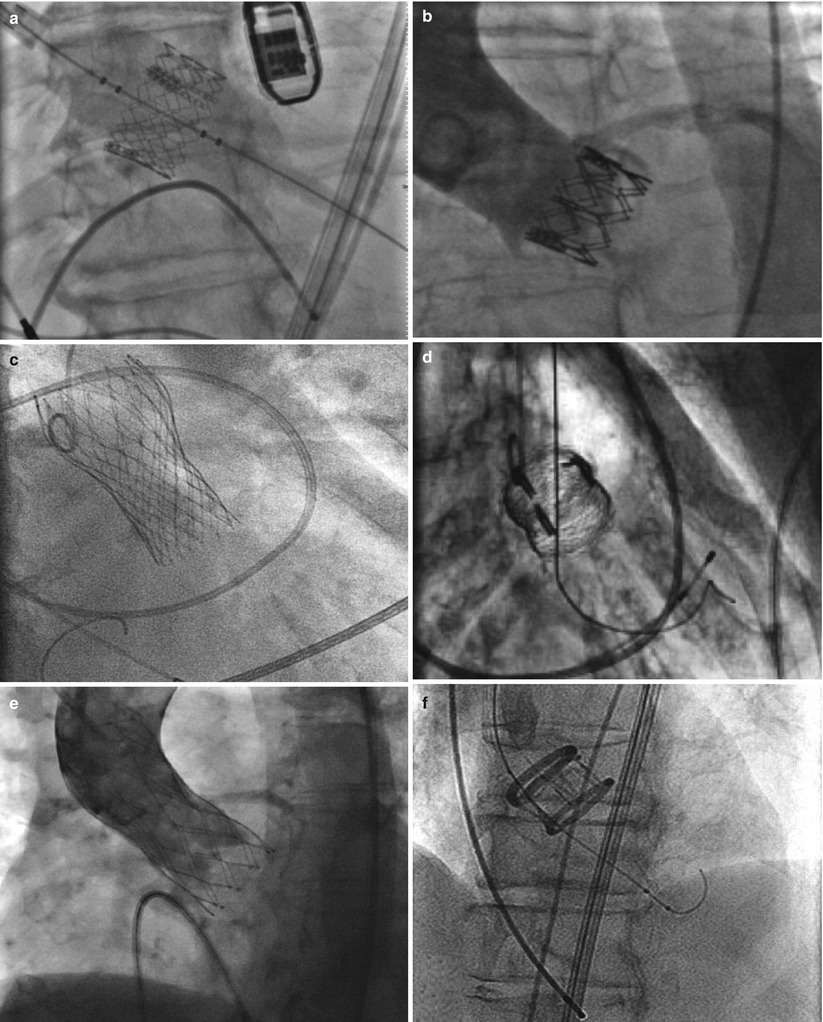
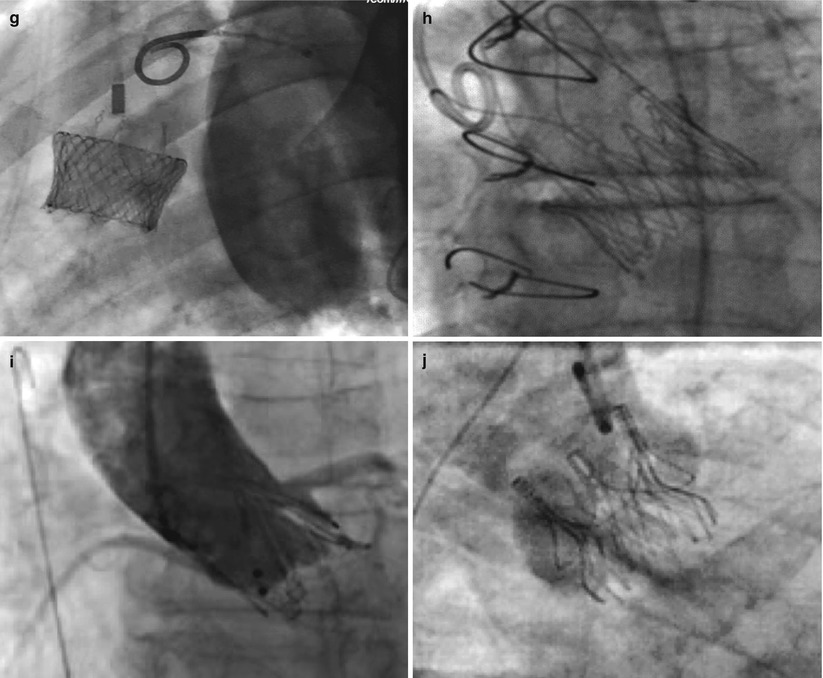
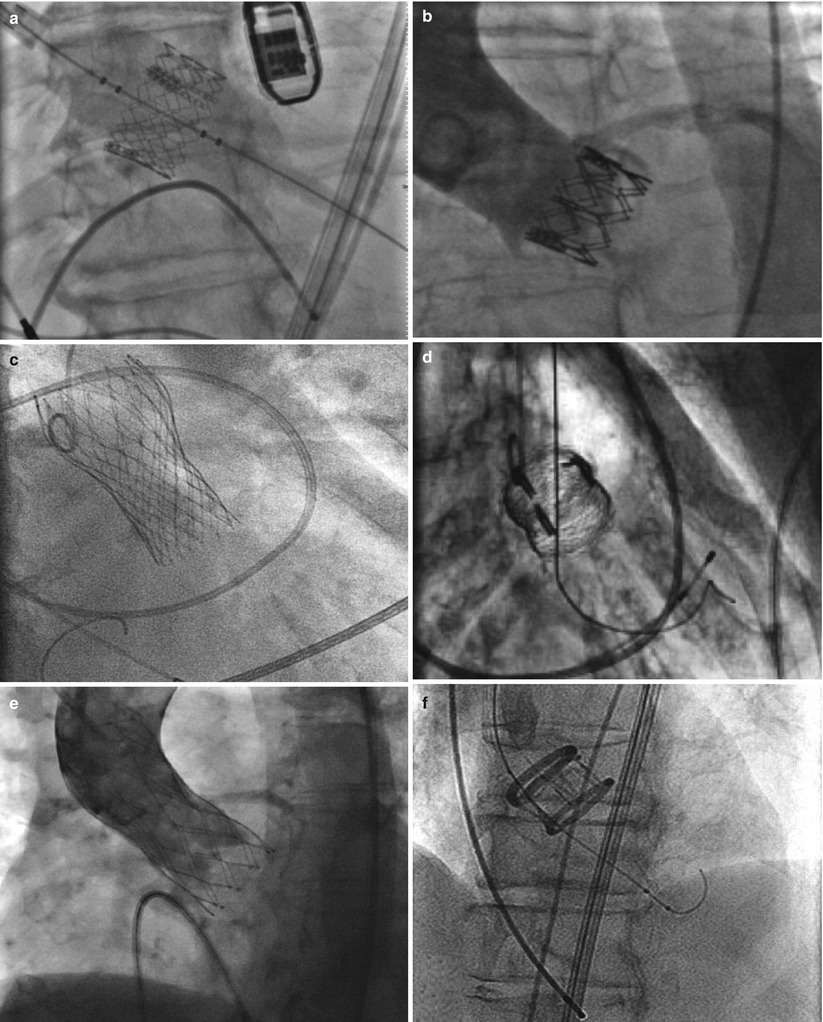
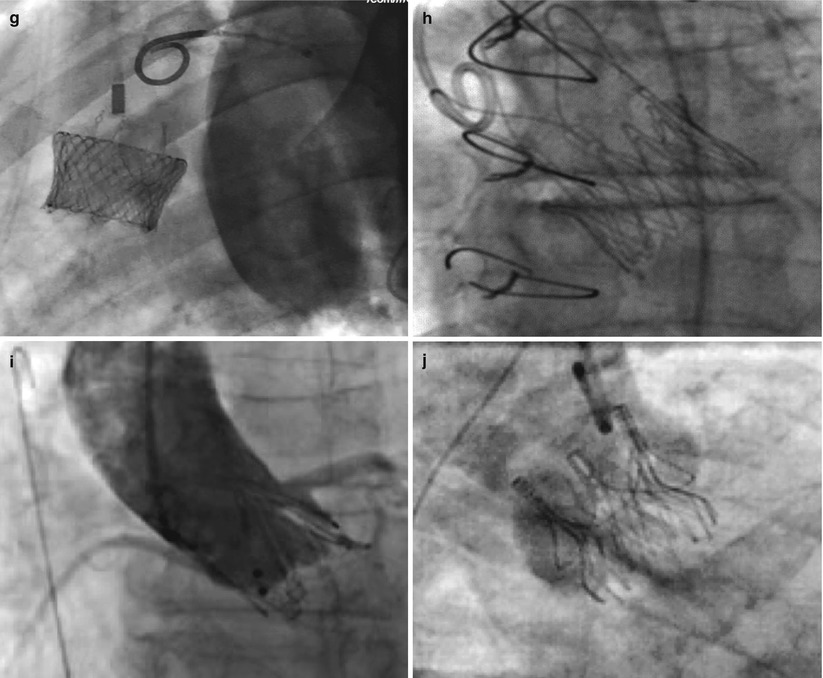
Fig. 7.9
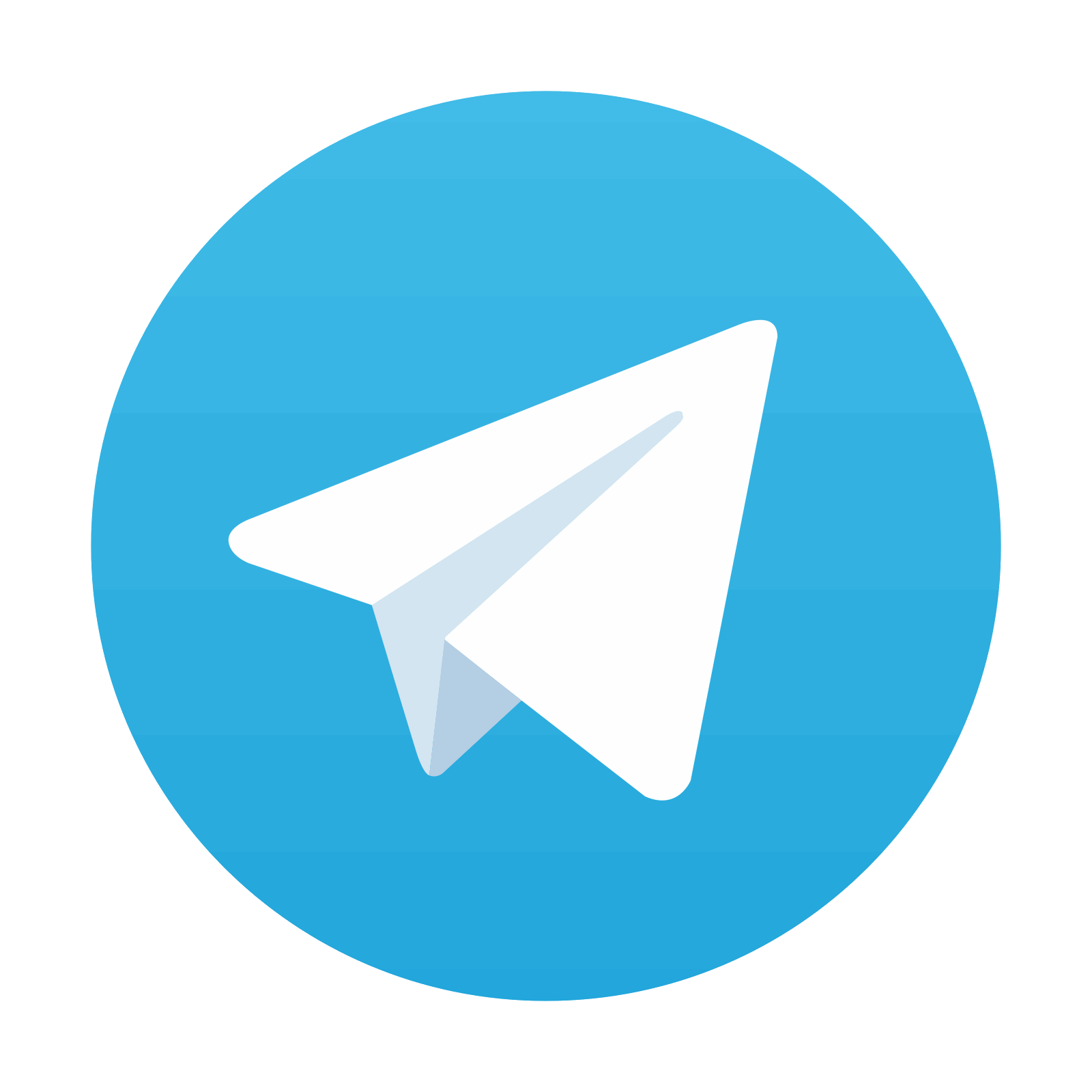
Fluoroscopic appearance of deployed transcatheter aortic valves. (a) Edwards-SAPIEN trascatheter heart valve (Edwards Lifesciences Corporation, Irvine, CA). (b) Sapien XT transcatheter heart valve (Edwards Lifesciences Corporation, Irvine, CA). (c) CoreValve (Medtronic Inc., Minneapolis, MN) (Reprinted from Grube et al. [42], with permission from Elsevier Inc.). (d) Sadra Lotus (Boston Scientific Corporation, Natick, MA) (Reprinted from Buellesfeld et al. [36], with permission from Wiley Periodicals Inc.). (e) Portico valve (St. Jude Medical, Inc., St. Paul, MN) (Reprinted from Willson et al. [43], with permission from Elsevier Inc.). (f) Direct Flow Medical (Direct Flow Medical, Inc., Santa Rosa, CA) (Reprinted from Schofer et al. [44], with permission from Lippincott Williams & Wilkins). (g) Heart Leaflet Technologies (Bracco Diagnostics, Inc., Princeton, New Jersey) (Image courtesy of HLT, Inc., © 2012). (h) JenaValve (JenaValve Technology, Inc., Wilmington, DE) (Reprinted from Treede et al. [45], with permission from Oxford University Press). (i) Acurate valve (Symetis Inc., Ecublens, Switzerland) (Reprinted from Kempfert et al. [46], with permission from Oxford University Press). (j) Engager valve (Medtronic Inc., Minneapolis, MN) (Reprinted from Falk et al. [47] with permission from Oxford University Press)
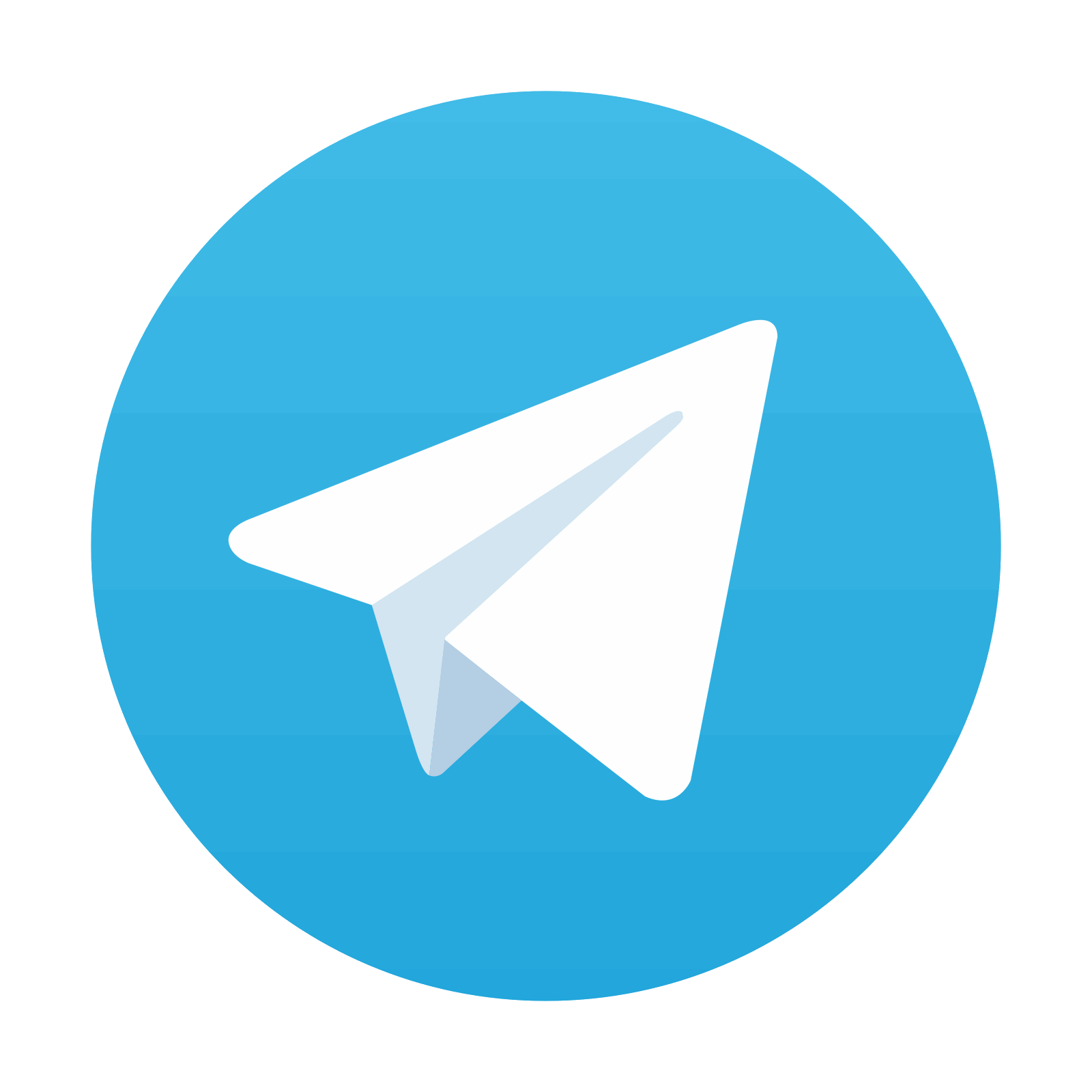
Stay updated, free articles. Join our Telegram channel

Full access? Get Clinical Tree
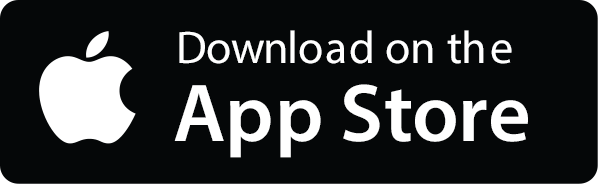
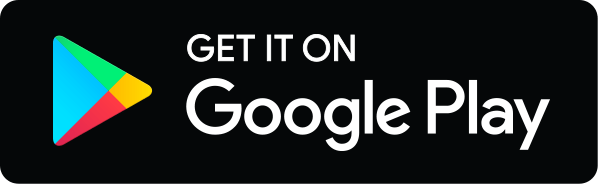
