Fig. 24.1
99mTechnetium-hexamnethyl propyleneamine oxime (HMPAO) SPECT after transient ischaemic attack (Adapted from You et al. 2000. With permission). (a) Imaging 12 h after clinical event in a 63-year-old male patient presenting with right lower extremity weakness, with complete recovery 20 min after onset. Reduced perfusion was demonstrated in the territory of the left middle cerebral artery. There was also reduced perfusion in the right cerebellar hemisphere. (b) Imaging 11 days after clinical event in a 67-year-old male patient presenting with left hemiparesis and slurred speech, with complete recovery 30 min after onset. Mildly reduced perfusion was seen in the territory of the right middle cerebral artery. There was also mildly reduced perfusion in the left cerebellar hemisphere
SPECT imaging has provided insight into changes in CBF after TIA, but there are no data to suggest that this information has any additive valve to clinical assessment and risk scoring for diagnosis and prognostication. Laloux et al. demonstrated in a group of 76 patients with TIA that whilst 99mTc-HMPAO had better sensitivity than contemporary (but now superseded) CT imaging, it provided no clinically useful information about vascular risk or aetiology (Laloux et al. 1996). In clinical practice, brain imaging in TIA has been supplanted by diffusion-weighted (DWI) magnetic resonance imaging. These techniques are more available, do without radioactive isotopes and have better data for their value in prognostication. DWI MR was first used to investigate stroke in the 1990s and ischaemic damage after clinically defined TIA is now increasingly recognised as sequences that develop further. Relevant focal DWI abnormalities are frequent in TIA patients, present in up to two-thirds of cases (Kidwell et al. 1999; Coutts et al. 2005a), though there is debate about how permanent such DWI lesions are and whether they represent reversible ischaemia or irreversible parenchymal damage (Oppenheim et al. 2006). The probability of DWI positivity in TIA increases with the duration of symptoms (Awad et al. 1986; Bogousslavsky and Regli 1985). DWI positivity, present in approximately half of TIA presentations, represents a high-risk subgroup. Baseline DWI positivity following TIA confers a significantly higher risk of recurrent ischaemic events (Purroy et al. 2004; Prabhakaran et al. 2007; Coutts et al. 2005b). One study found a 90-day stroke rate of 10.8 % among DWI-positive patients and 4.3 % in those without a DWI lesion (Coutts et al. 2005b). Clinical features consistently associated with ischaemic lesions in TIA patients include longer symptom duration and motor and speech disturbance (Prabhakaran et al. 2007; Redgrave et al. 2007; Crisostomo et al. 2003) but can also be seen in patients with very brief sensory symptoms (Crisostomo et al. 2003; Lamy et al. 2006).
24.3 Vascular Imaging with PET and SPECT
Non-invasive assessment of atherosclerotic plaques after TIA is needed to better predict recurrent events, improve selection of candidates for endarterectomy, provide information regarding the underlying vascular biology and track the effect of novel interventions which will rely on interference with key identified pathways. Single-photon emission computed tomography (SPECT) and positron emission tomography (PET) are able to detect picomolar levels of radiopharmaceutical at the target site, thus making them ideal for investigating processes within atherosclerotic plaque. PET imaging has greater spatial resolution (4–5 mm versus 1–1.6 cm) than SPECT (Davies et al. 2006) though given the size of carotid atherosclerotic plaques is still subject to partial volume error (Soret et al. 2007). Co-registration with CT or MRI is needed to localise PET tracer uptake to underlying anatomy. Many of the human imaging studies have included patients with both TIA and stroke.
24.3.1 Atherosclerosis Imaging with SPECT
Numerous metabolic and signalling pathways associated with plaque vulnerability have been evaluated with SPECT in preclinical models of atherosclerosis. These include the oxidation and accumulation of LDL (low-density lipoproteins) with 125iodine-labelled oxidation-specific antibodies (Torzewski et al. 2004), matrix metalloproteinase activity with 99mtechnetium (Haider et al. 2009) and 111iridium-labelled (Razavian et al. 2011) matrix metalloproteinase inhibitors, vascular cell adhesion molecule (VCAM)-1 with 18F-4V (Nahrendorf et al. 2009) and, finally, monocyte recruitment with 99mtechnetium-labelled monocyte chemotactic protein-1 (Hartung et al. 2007).
99mTechnetium-labelled annexin A5 has been used to image macrophage apoptosis in preclinical models (Zhao et al. 2008), and in a pilot clinical study (Kietselaer et al. 2004), uptake was seen in carotid atherosclerosis after recent TIA. This study included four patients; two had been diagnosed with TIA only days previously. The authors were able to demonstrate tracer uptake in the culprit carotid lesions of both of these patients, with corresponding histology characteristic of unstable atherosclerotic plaque (Fig. 24.2). Tracer binding appeared specific to macrophage cell membranes. The two patients with more distant clinical events had negligible tracer uptake and histological features of a more stable plaque, suggesting that this technique may provide an ability to identify plaque instability and at-risk patients. Further clinical studies are awaited.
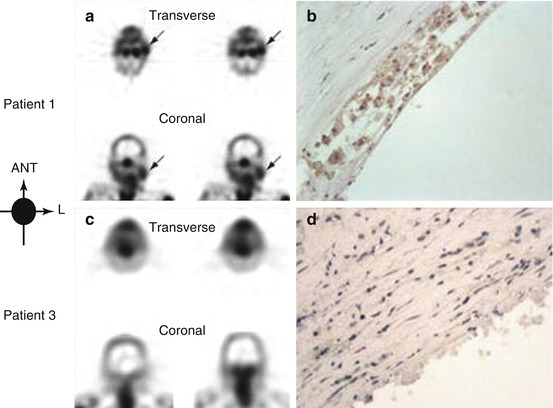
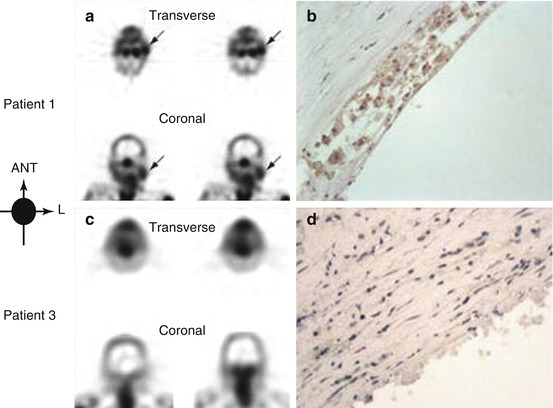
Fig. 24.2
Images of unstable atherosclerotic carotid artery lesions obtained with radiolabelled annexin A5 (Adapted from Kietselaer et al. 2004. With permission). Panel (a) shows transverse and coronal views obtained by single-photon emission computed tomography (SPECT) in patient 1, who had a left-sided transient ischaemic attack (TIA) 3 days before imaging. Although this patient had clinically significant stenosis of both carotid arteries, uptake of radiolabelled annexin A5 is evident only in the culprit lesion (arrows). Histopathological analysis of an endarterectomy specimen from patient 1 (Panel b; polyclonal rabbit anti-annexin A5 antibody, 400) shows substantial infiltration of macrophages into the neointima, with extensive binding of annexin A5 (brown). In contrast, SPECT images of patient 3 (Panel c), who had had a right-sided TIA 3 months before imaging, do not show evidence of annexin A5 uptake in the carotid artery region on either side. Doppler ultrasonography revealed a clinically significant obstructive lesion on the affected side. Histopathological analysis of an endarterectomy specimen from patient 3 (Panel d; polyclonal rabbit anti-annexin A5 antibody, 400) shows a lesion rich in smooth muscle cells, with negligible binding of annexin A5. ANT denotes anterior, and L left
In attempt to image inflammation with SPECT, Annovazzi et al. described uptake of 99mTc-labelled interleukin-2 (IL-2) in the carotid arteries of patients undergoing endarterectomy, correlating with the percentage of IL-2 receptor-positive cells at histology (Annovazzi et al. 2006). The majority of these patients were, however, asymptomatic, only four having a history of TIA.
Lees et al. described accumulation of 99mtechnetium-LDL in the carotid artery in a single patient with ‘vulnerable’ features on histology of the explanted plaque (Lees et al. 1988). No accumulation of tracer was seen in the five other patients undergoing endarterectomy (four with a history of TIA), though ex vivo, it was possible to detect some focal uptake. These plaques were all of a more stable phenotype on histology. Though promising, the authors highlighted poor target-to-background ratios and slow plasma clearance as a limitation of this technique.
Further studies in human atherosclerosis are awaited; however, greater attention has been placed on PET imaging of atherosclerosis because of its better spatial resolution and available ligands in use in the clinical arena (mostly oncology).
24.3.2 Imaging Inflammation with 18F-fluorodeoxyglucose(FDG)
FDG is the most commonly used tracer for PET imaging of atherosclerosis. It exploits the fact that macrophages, key inflammatory cells in plaque, have higher glucose metabolism than both surrounding plaque cells and healthy artery wall (Björnheden et al. 1999; Mayr et al. 2008; Leppänen et al. 2006). FDG, a glucose analogue, competes with endogenous glucose for facilitated transport sites and after phosphorylation becomes trapped within the cells it has entered. This intracellular accumulation, a reflection of glucose usage and by extension metabolic activity, can then be imaged and quantified in the PET scanner. FDG PET has become established as the gold standard imaging modality for the detection of tumour metastases in oncology, where it again exploits their higher metabolic requirements for glucose than non-malignant cells.
Arterial FDG uptake was first noted in patients undergoing PET for cancer staging (Yun et al. 2001). After balloon aortic injury in a rabbit model of atherosclerosis, FDG uptake in atherosclerotic regions correlated strongly with macrophage content (r =0.93, p <0.002) (Tawakol et al. 2005), findings confirmed by others (Vucic et al. 2011; Patel et al. 2011). In the first prospective study in man, levels of inflammation measured as accumulation rate of FDG were 27 % greater in the culprit carotid after recent stroke or transient ischaemic attack (TIA) than in the contralateral vessel (Rudd et al. 2002), though with contemporary therapy, this difference is not apparent beyond 38 days of the event (Kwee et al. 2011) (Fig. 24.3). Autoradiography of carotid plaque specimens reported in the same paper by Rudd et al. confirmed co-localisation of tracer to regions of macrophage staining (Rudd et al. 2002). Further work has confirmed FDG uptake correlates with areas of macrophage (CD68+) staining (r = 0.96, p < 0.001) and is independent of plaque thickness, area or luminal stenosis (Tawakol et al. 2006). FDG uptake within an individual carotid plaque appears to be highly variable with focal ‘hot spots’ seen on ex vivo imaging with co-registered micro-PET and MR. In a published case report, such ‘hot spots’ correspond to regions of inflammatory cell infiltration and concomitant neovasculature on histology (Kerwin et al. 2012). The region of maximal FDG uptake in this plaque co-localised with a recent intraplaque haemorrhage and significant macrophage infiltration. FDG uptake is thought to reflect activation status of macrophages (Newsholme et al. 1986; Deichen et al. 2003), though recent work by Folco et al. suggests that hypoxia, known to exist within atherosclerotic plaque (Sluimer et al. 2008), may also be an important determinant of glucose (and hence FDG) uptake into these cells (Folco et al. 2011) (Figs. 24.4 and 24.5). Nevertheless, FDG PET remains the best-validated tracer in molecular atherosclerosis imaging.
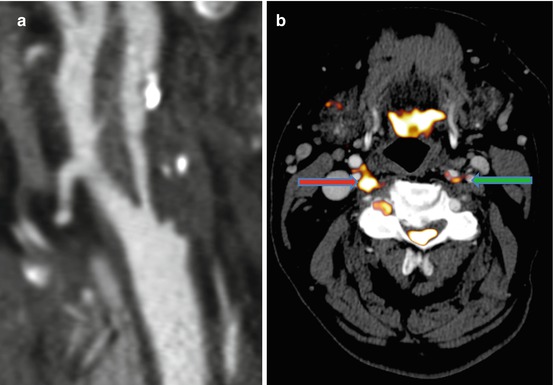
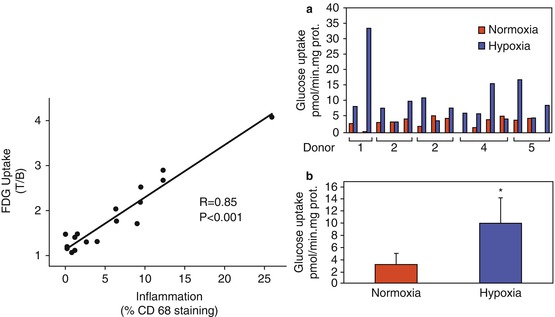
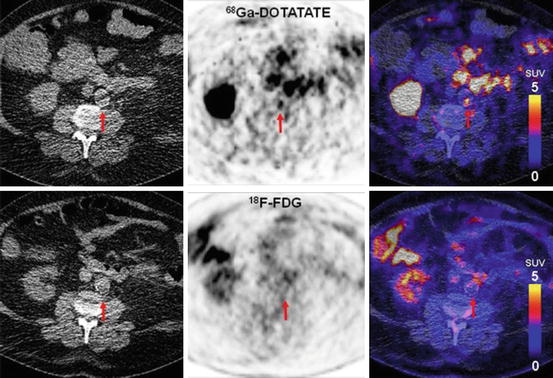
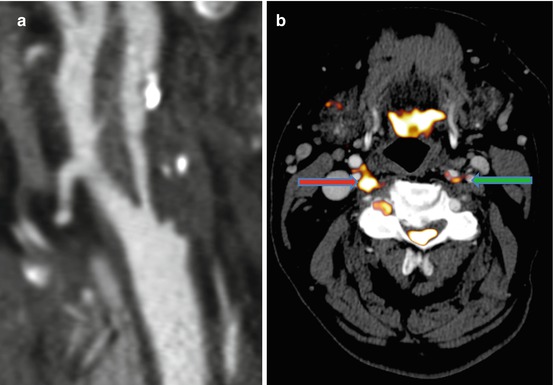
Fig. 24.3
18F-FDG PET/CT imaging in symptomatic carotid atherosclerosis. (a) CT angiography: 3D multi-planar reformatted image of a severely stenosed right internal carotid artery 1 week after an acute cerebrovascular event in a 69-year-old man. (b) 18F-FDG PET: transaxial image of PET co-registered to CT angiography in the same patient. Intense tracer uptake is noted in the culprit right internal carotid artery (red arrow). Less uptake is noted in the left internal carotid artery (green arrow)
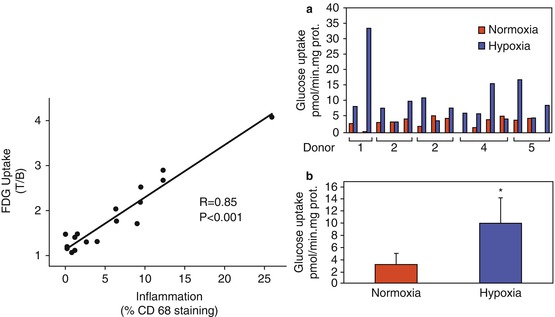
Fig. 24.4
What does 18F-fluorodeoxyglucose uptake in atherosclerosis tell us about plaque biology? Left hand panel: FDG uptake (expressed as target-to-background ratio) strongly correlates with macrophage infiltration (expressed as percentage area staining with CD68 immunohistochemistry) on histological analysis of carotid plaque removed at endarterectomy (From Tawakol et al. 2006. With permission). Right hand panel: glucose uptake in specimens from human carotid atherosclerotic lesions. (a) Absolute rates of glucose uptake in carotid endarterectomy specimens from five donors, alternately exposed to hypoxia (blue bars) or kept in normoxia (red bars) for 24 h. (b) Average of the glucose uptake determinations in (a). *p < 0.005. Prot. protein (From Folco et al. 2011. With permission)
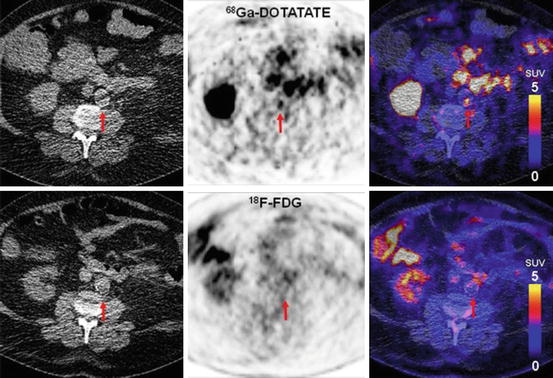
Fig. 24.5
Focal vascular uptake of 68Ga-DOTATATE without corresponding focal 18F-FDG uptake (From Li et al. 2012. With permission). Transverse PET/CT images of a 73-year-old male patient with hypertension and hypercholesterolaemia and history of smoking. Intense focal uptake of 68Ga-DOTATATE can be observed in the aortic arch (upper row), whereas no focally increased 18F-FDG uptake was seen (lower row). TBRDOTATATE was 7.60, whilst TBRFDG was 1.74
Arterial FDG signal is linked to male sex, age, cardiovascular risk factors (Yun et al. 2002; Tatsumi et al. 2003; Tahara et al. 2007a, b), levels of inflammatory biomarkers matrix metalloproteinases 1, 3 and 9 (Wu et al. 2007; Rudd et al. 2009) and genetic markers of plaque instability (Pedersen et al. 2010). Results of associations with serum C-reactive protein (CRP) have been mixed, but healthy individuals with the highest quartile of CRP had the greatest degree of carotid FDG uptake in one recent study (Yoo et al. 2011). Plaques with high-risk features on MRI (large lipid core (Silvera et al. 2009) or intraplaque haemorrhage (Kwee et al. 2009)) or echolucency on ultrasound (Choi et al. 2011) have higher FDG uptake than more stable phenotypes. Davies et al. recruited 12 patients after TIA with severe carotid stenosis, all of whom underwent endarterectomy (Davies et al. 2005a). In seven of the patients, FDG accumulated in the carotid plaque removed at surgery. However, in the remainder, there was no significant FDG uptake. By demonstrating FDG uptake in non-stenotic lesions within the relevant arterial territory, and in one case, the posterior circulation, this work suggested that in some patients, the obvious stenotic carotid plaque might not be the culprit lesion. Endarterectomy in these circumstances may therefore not be the most appropriate management. Further work by the same group has shown that carotid FDG uptake in the carotid artery correlates with microembolic signals on transcranial Doppler ultrasound after TIA (Moustafa et al. 2010).
FDG PET is a reproducible measure, valid across several vascular beds with excellent short-term and interobserver reproducibility (Silvera et al. 2009; Rudd et al. 2007; Davies et al. 2005b). Rarely co-localising with vascular calcification (Dunphy et al. 2005; Rudd et al. 2009), uptake may be widespread (Tahara et al. 2007b; Rudd et al. 2009), though especially affecting the carotid arteries and proximal aorta. This is consistent with the hypothesis that atherosclerosis is a generalised (rather than focal) inflammatory condition.
24.3.3 Prognostic Implications of Vascular FDG Uptake
In retrospective analyses of patients undergoing PET for oncology staging, high levels of baseline vascular FDG uptake were associated with subsequent cardiovascular events (Rominger et al. 2009), including ischaemic stroke (Grandpierre et al. 2011). In the investigation of symptomatic carotid atherosclerosis, emerging evidence suggests that FDG PET imaging can have prognostic value. In a pilot study (Arauz et al. 2007), patients with recent symptomatic (TIA or stroke) carotid lesions underwent FDG PET imaging after angiography. They found high levels of uptake in 11 out of 13 symptomatic patients. Despite treatment, six patients suffered from an ischaemic stroke, death or restenosis after stenting, during follow-up. All of these six patients had highly elevated FDG uptake. The results of prospective event-driven studies are awaited, including the BioImage Study that aims to identify imaging markers (CT, MRI and FDG PET/CT) of future cardiovascular risk in asymptomatic patients (Muntendam et al. 2010).
More recently, with a larger series of 60 patients after TIA and stroke, Marnane et al. have shown that high uptake of FDG predicts recurrent stroke independently of the degree of carotid stenosis (Marnane et al. 2012). As others have shown, there was no relationship between stenosis and carotid FDG uptake (Tawakol et al. 2006). The authors were able to define thresholds of FDG uptake to accurately identify the risk of recurrent stroke; levels above this conferred a 14-fold increase in the accuracy of the clinical estimate of the likelihood of stroke recurrence in a recently symptomatic patient with carotid stenosis. Additionally, because some of these patients with FDG uptake above the threshold will have undergone endarterectomy, it is likely that this figure represents an underestimate of the predictive power of FDG PET. These data need further validation but suggests FDG PET may have a role in the selection of candidates for endarterectomy.
24.3.4 Emerging Indications for FDG PET
Increasingly, arterial FDG PET/CT imaging is being applied for the early assessment of anti-atherosclerotic therapy. Published studies have used statin drugs, with positive signal changes after only 3 months’ therapy (Tahara et al. 2006). In addition, ezetimibe (Patel et al. 2011) and dietary modification (Lee et al. 2008) seem to have beneficial effects. Consistent with clinical trial data (Ebbing et al. 2008), B vitamins did not reduce vascular inflammation as assessed by FDG PET (Potter et al. 2009). Investigating the method by which pioglitazone reduces cardiovascular events, Mizoguchi et al. used FDG PET/CT to show that the drug reduced vascular inflammation as compared to glimepiride in patients with diabetes or impaired glucose tolerance (Mizoguchi et al. 2011). The recent dal-PLAQUE trial with dalcetrapib (Fayad et al. 2011) has further demonstrated the value of FDG PET for this purpose, providing evidence of vascular safety (i.e. no increase in inflammation) versus placebo at 6 months.
24.3.5 Limitations of FDG PET
A limitation of PET imaging with FDG is its lack of cellular specificity (FDG is accumulated by all cells that metabolise glucose). This has prompted the search for alternative tracers more specific for macrophages. Many already have applications in oncology. Those showing promise include radiolabelled choline (Matter et al. 2006), taken up and integrated into cell membranes in tumour cells and macrophages, and crucially for coronary imaging not accumulated by myocardial cells (Bucerius et al. 2008). 11C-choline was not taken up into normal vascular wall, or purely calcified lesions, in a retrospective analysis of 93 male patients undergoing cancer imaging (Kato et al. 2009). Ninety-five percent of patients in this study had evidence of tracer uptake in the left and right common carotid arteries, though no correlation was made with either cardiovascular risk factors or prior cerebrovascular events.
The somatostatin receptor SSTR-2 is specifically expressed and upregulated in activated human macrophages (Armani et al. 2007) and provides another potential target for imaging macrophages in atherosclerosis. Coronary artery uptake has been described for the somatostatin receptor analogue 68Ga-DOTATATE in the left anterior descending coronary artery, correlating with prior cardiovascular events (Rominger et al. 2010). More recently, a retrospective study of cancer imaging has demonstrated 68Ga-DOTATATE uptake in large arteries, including the carotids, correlating with the presence of calcified plaques, age, hypertension and vascular FDG uptake (Li et al. 2012, Fig. 24.5). Concordant vascular uptake of both tracers was only seen in a minority of the cases reviewed.
In a small retrospective series such as this, the reasons for these differences are not clear. They may reflect greater specificity of 68Ga-DOTATATE for activated macrophages, suggested by the fact that very few 68Ga-DOTATATE-positive foci could be found in low-risk individuals. However, the different kinetics of the two tracers (68Ga-DOTATATE uptake into macrophages being ultimately limited by saturation of receptor sites) may also have influenced the results of this retrospective analysis. Additionally, expression of SSTR-2 is upregulated in proliferating human umbilical vein endothelial cells (Adams et al. 2005). Neovascularisation (derived from the vasa vasorum) is a feature of mature atherosclerotic plaques (Moreno et al. 2006), and it is possible that 68Ga-DOTATATE uptake is also reporting on this process. Histological validation and prospective clinical studies are required to evaluate this promising approach to imaging vascular inflammation.
Yet another potential target is the macrophage translocator protein (TSPO), highly expressed in the activated state. In vitro, the TSPO ligands PK11195 and DAA1106 will bind to macrophage-rich regions in human carotid plaque (Bird et al. 2010). In a recent prospective clinical study in carotid atherosclerosis, imaging with the PET ligand 11C-PK11195 was found to correlate with the extent of immunohistochemical macrophage infiltration (Gaemperli et al. 2012). Additionally, significant differences in uptake were noted between recently symptomatic lesions and those remaining asymptomatic. However, mixed binding affinity in humans (Owen et al. 2011), poor target-to-background ratios and the short half-life of 11C (requiring on-site synthesis) may limit the practical application of this tracer.
24.3.6 Imaging Vascular Calcification
18F-sodium fluoride (18F-NaF) is a positron-emitting bone-seeking agent used clinically to identify primary osteoblastic tumours and bone metastases (Grant et al. 2008). After intravenous injection, it diffuses from the bloodstream into the extracellular fluid (ECF). Fluoride ions then exchange with hydroxyl groups at the surface of bone crystals to form fluoroapatite. Uptake of NaF within calcified structures such as bone reflects both blood flow and osteoblastic activity (Even-Sapir et al. 2007). It therefore detects areas of new calcification. Retrospective studies, all performed in oncology patients, have shown that 18F-NaF can provide insight into the process of vascular calcification (Derlin et al. 2010, 2011a, b). There are positive correlations between the extent of 18F-NaF uptake and the presence of cardiovascular risk factors. Subjects previously imaged with both FDG and 18F-NaF demonstrated that vascular lesions seldom have coincident uptake of both tracers, implying each reflects distinct pathophysiologic processes within atherosclerosis (Derlin et al. 2011a). Eighty percent of calcified arterial sites appear ‘inert’, but uptake in the remainder suggests the tracer may have a role in imaging dynamic micro-calcification in advance of current imaging techniques.
In a recent study in coronary atherosclerosis (a sub-study of an investigation into aortic stenosis), discrete regions of 18F-NaF activity were commonly observed in the coronary arteries that could be localised to individual plaque (Dweck et al. 2012a). 18F-NaF activity was higher in patients with atherosclerosis compared to those without and rose steadily with progressive disease burden. Patients with atherosclerosis and increased tracer uptake were more likely to have anginal symptoms, a clinical diagnosis of coronary artery disease, previous major adverse cardiovascular events and prior coronary revascularisation. Furthermore, Framingham risk scores were higher in this group. Increased coronary 18F-NaF activity therefore appears to occur in patients with a high-risk profile. 18F-NaF uptake correlated with coronary artery calcium scores, but interestingly, 41 % of patients with very high scores (>1,000) had normal uptake.
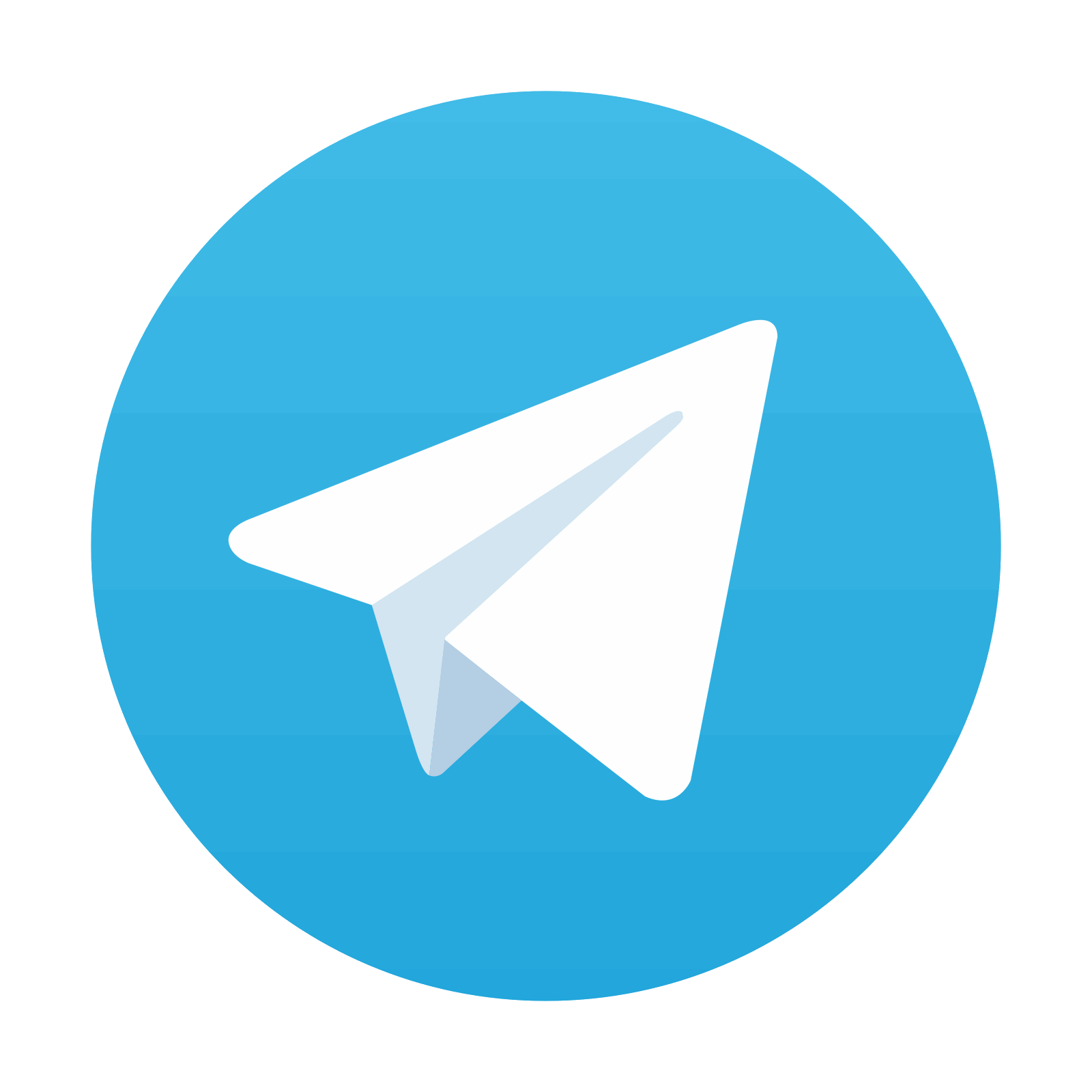
Stay updated, free articles. Join our Telegram channel

Full access? Get Clinical Tree
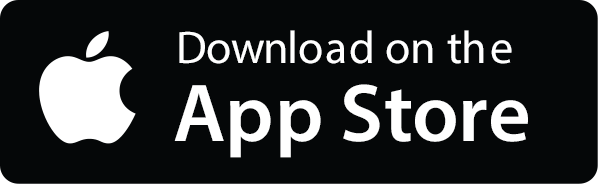
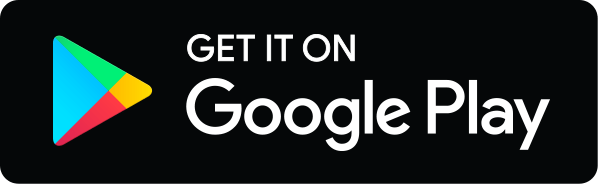