Abstract
The introduction of magnetic resonance imaging (MRI) to the field of spinal cord injury (SCI) has vastly improved the diagnosis of injury level and severity as well as the prediction of long-term functional outcomes (see previous chapter on acute SCI and prognosis). Additionally, MRI represents a noninvasive clinical instrument to assess anatomical changes in the spinal cord after injury in longitudinal investigation. With the advent of more advanced techniques, it is also possible to differentiate macroscopic changes ongoing in anatomically distinct regions of the spinal cord (i.e., gray and white matter). Importantly, these changes may be subthreshold to detection according to other clinical measurement instruments. The primary aims of this chapter will be to describe: (1) the current state of MRI as a complementary tool to the neurophysiological examination of SCI for the purpose of monitoring changes during the natural course of spontaneous recovery, and (2) future MRI applications to disclose anatomical regeneration/repair due to rehabilitation interventions.
Keywords
Clinical outcomes, Electrophysiology, Motor evoked potentials, MRI paradox, Neurophysiology, Regeneration, Rehabilitation, Sensory evoked potentials, Spontaneous recovery
1.3B.1
Introduction
The introduction of magnetic resonance imaging (MRI) to the field of spine trauma has vastly improved the clinical diagnosis of spinal cord injury (SCI). MRI has advantages over conventional X-ray and computer tomography (CT) as it more precisely details posttraumatic compression of the spinal cord, either due to soft tissue (i.e., traumatic disc herniation or bleeding within the spinal canal) or spinal canal encroachment (e.g., following a burst fracture or vertebral misalignment). Also in cases where an SCI is clinically suspected, but without obvious vertebral column fracture or disco-ligamentary injury (SCIWORA = spinal cord injury without obvious radiological abnormality), MRI is essential for the diagnosis of injury and planning of appropriate surgical interventions. In addition, MRI studies in acute SCI are applied in order to estimate long-term outcomes (i.e., prognosis) and as a postsurgical outcome measure in selected cases to confirm that the spinal cord is indeed sufficiently decompressed.
With the advent of novel treatment options aiming at the repair of the injured spinal cord, insights into disease mechanisms paralleling functional recovery are essential in order to distinguish treatment-induced changes from spontaneous recovery (i.e., pattern and extent of repair/regeneration beyond spontaneous recovery). Regardless if applied during spontaneous or therapeutically derived recovery, changes in anatomical substrates in the spinal cord may be subthreshold to detection compared to other clinical measurement instruments. However, compared to the brain, MRI of the spinal cord faces a number of technical (i.e., artifacts) and anatomical (i.e., small size) challenges that limit conventional approaches for cross-sectional and longitudinal studies. Consequently, fewer advanced MRI techniques have reached the level of clinical (i.e., routine and robust) applicability to evaluate damage/integrity at the level of the spinal cord lesion. The primary aims of this chapter will be to describe: (1) the current state of MRI as a complementary tool to the neurophysiological examination of SCI for the purpose of monitoring changes during the course of recovery during standard rehabilitation, and (2) future MRI applications to disclose anatomical regeneration/repair due to novel treatment (medical or surgical) and/or rehabilitation interventions.
1.3B.2
Neurophysiology and MRI: Complementary Approaches
At present, most clinical applications of MRI to assess the injured spinal cord are rather qualitative (i.e., defining the localization, size, and type of damage, such as bleeding and compression). Therefore, MRI findings of the injured spinal cord need to be complemented by clinically meaningful readouts as well as quantitative neurophysiological measures that provide additional insights into spinal cord function. Neurophysiological approaches commonly adopted after SCI include those that objectively examine spinal conduction in specific ascending and descending pathways. Ascending fibers in the dorsal columns, which convey light touch sensation and proprioception, are most frequently studied by measuring somatosensory evoked potentials (SSEPs) using electroencephalography (EEG) techniques following surface electrical stimulation of mixed nerves in the periphery (e.g., tibial nerves). The other major ascending sensory pathway, conveying pain and temperature sensation (i.e., spinothalamic tract), can likewise be investigated monitoring evoked EEG responses to contact or radiant heat stimulation (contact heat evoked potentials, CHEPs, or laser evoked potentials, LEPs, respectively). Descending pathways (e.g., corticospinal tract) are routinely examined by measuring motor evoked potentials (MEPs) following noninvasive stimulation of the motor cortex (i.e., transcranial magnetic stimulation or TMS) and recording electromyography (EMG) in the periphery. Whether ascending or descending pathways are examined, neurophysiological outcomes provide an objective readout of spinal conduction based on latency and amplitude of the measured cortical or muscle responses. Therefore, sensory (SSEP) and motor (MEPs) evoked potentials are principally important for detecting lesions in affecting spinal white matter.
The primary objective of implementing neurophysiology after SCI is to assess the severity of sensory and motor deficits related to the damaged area of spinal cord (i.e., localization and density of damage), independent of complex sensorimotor function (i.e., motor task or rating of sensory perception). The goal of coupling neurophysiological outcomes with MRI is not to provide redundant information regarding the severity of damage of the spinal cord (as revealed by SSEPs, CHEPs/LEPs, or MEPs) but rather as a complementary assessment relating morphological and functional changes. Although there is a relationship between functional and anatomical findings (i.e., in complete cord damage and absence of SSEPs/LEPs/MEPs), the functional impact of morphological changes (e.g., swelling and edema of the cord or hemorrhage) on the performance of complex sensorimotor tasks may vary considerably between subjects. This could be related to a number of factors, including the heterogeneity of the lesion area in the white and gray matter.
- •
International Standards for the Neurological Classification of SCI light touch and pinprick, and motor scores
- •
Electrophysiology (somatosensory and motor evoked potentials, contact heat evoked potentials, nerve conduction)
- •
T1- and T2-weighted MRI
1.3B.3
Morphological Changes in the Spinal Cord after SCI
The primary strength of MRI in the acute stages of SCI is to objectively assess the localization and extent of morphological damage in the spinal cord, from which surgical interventions can be planned. During the transition from the acute to chronic stage of SCI, the role of MRI changes, with the emphasis to disclose secondary morphological changes ongoing in the spinal cord. Therefore, it is important to understand the typical evolution of spinal cord damage according to clinical MRI findings and the extent to which observed changes can be related to clinical outcome. From the perspective of a clinical trial, an understanding of the dynamic temporal and spatial pattern of the changes in the gross damage and eventual formation of the posttraumatic (i.e., consolidated) lesion area is required to distinguish potential effects of interventions on the lesion area.
While the acquisition of MRI after SCI is routinely performed in the acute stages of injury, there are no studies that have systematically tracked (prospective longitudinal follow-up) changes in the spinal cord during the course of recovery. This is likely due, in part, to some of the challenges of spinal cord MRI. However, based on clinical observations of T1- and T2-weighted anatomical images collected serially in the first year after SCI in patients demonstrating representative patterns of spontaneous recovery, and where surgical instrumentation was either not implanted or not resulting in significant MRI artifacts, SCI are often characterized by three prominent morphological stages ( Figure 1.3B.1 ).
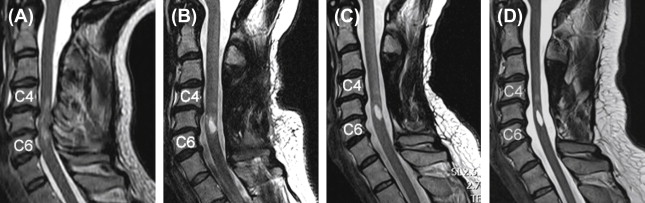
The first stage corresponds to the acute onset of edema and hemorrhage spreading rostrally and caudally from the injury epicenter. T1-weighted images are generally more sensitive to detecting hemorrhage, whereas T2-weighted images are sensitive to edema. An intramedullary high intensity signal change can be observed on sagittal images in the early hours after SCI and may extend across a number of segments ( Figure 1.3B.1 (A)). The signal change at this point is generally diffuse, and it may be difficult to define the most rostral and caudal boundaries.
The second stage is marked by ongoing resolution of posttraumatic edema and hemorrhage, evident by an apparent shrinking of the rostral-caudal extent of the high intensity intramedullary signal change ( Figure 1.3B.1 (B–C)). Lastly, the formation of a posttraumatic cyst, the rostral-caudal and ventral-dorsal boarders of which are generally well defined, represents the final morphological stage of SCI. Atrophic shrinking of the affected area of spinal cord in the sagittal plane (i.e., rostral-caudal) becomes obvious at this stage. At this point, the area of the posttraumatic cyst is generally stable, and further changes in size are not expected. Although the specific timing stages 1 and 2 are likely to vary considerably between subjects, the formation of the cyst should be complete within the first year after injury ( Figure 1.3B.1 (C and D)).
- •
Stage 1: Rostral and caudal spread of edema and hemorrhage
- •
Stage 2: Resolution of edema and hemorrhage
- •
Stage 3: Formation of posttraumatic cyst and spinal cord atrophy rostral and caudal to injury epicenter
1.3B.4
Relationship between Neurological Recovery and Morphological Changes
Some changes in clinical MRI findings may correspond with obvious features of spontaneous neurological recovery. For example, the greatest period of spontaneous neurological recovery according to clinical sensory and motor testing outcomes is expected in the initial months after injury, begins to plateau at approximately 4–6 months, and remains relatively stable thereafter. Therefore, spontaneous recovery roughly occurs in parallel with some of the morphological changes described before. However, the relationship between morphological changes and spontaneous recovery is poorly understood. Even in patients where gross morphological changes are observed (e.g., enlargement of the posttraumatic cyst), the severity of injury may not change and the patient remains clinically stable. While this represents an apparent discrepancy between MRI and functional outcomes in longitudinal pathways traversing the lesion epicenter, this underscores the importance of coupling MRI with neurophysiological outcomes to assess function. Indeed, neurophysiological outcomes assessing conduction in ascending and descending pathways traversing through the lesion level generally remain unchanged during recovery.
Although the majority of individuals with SCI are expected to moderately improve from their initial sensory and motor deficits, the trajectory of spontaneous recovery can take a deleterious turn of events in the months and years following SCI. In fact, the role of clinical MRI techniques in disclosing neurological complications (e.g., posttraumatic tethering and/or progressive syrinx formation) associated with SCI is much better defined than the role of monitoring spontaneous neurological recovery. The incidence of the development of posttraumatic syringomyelia after SCI has been reported between 5% and 8%. Particularly when coupled with obvious signs of descending neurological deficits (e.g., loss of muscle strength in myotomes rostral to the initial level of injury), the appearance of posttraumatic tethering and/or progressive syrinx formation according to MRI often warrants surgical intervention to prevent further deterioration. In some cases where a posttraumatic cyst develops, surgical intervention is potentially warranted based solely on MRI findings if further increases in rostral-caudal boundary threaten vital brainstem functions (i.e., breathing). The onset of symptoms related to syrinx formation can be rapid, thus imaging the spinal cord for morphological changes to confirm the diagnosis is paramount. In the majority of cases, following surgical intervention collapsing of the syrinx can be imaged by T2-weighted MRI. Neurophysiology coupled with MRI has an important role in confirming that the developing cyst is altering conduction in the spinal cord. Owing to the fact that small diameter afferent fibers conveying temperature sensation decussate across spinal segments before ascending in the spinothalamic tract, CHEPs/LEPs are particularly useful to examine the functional significance of lesions localized or developing in the central gray matter. This can be achieved by stimulating dermatomes corresponding to segments adjacent to the imaged level of pathology.
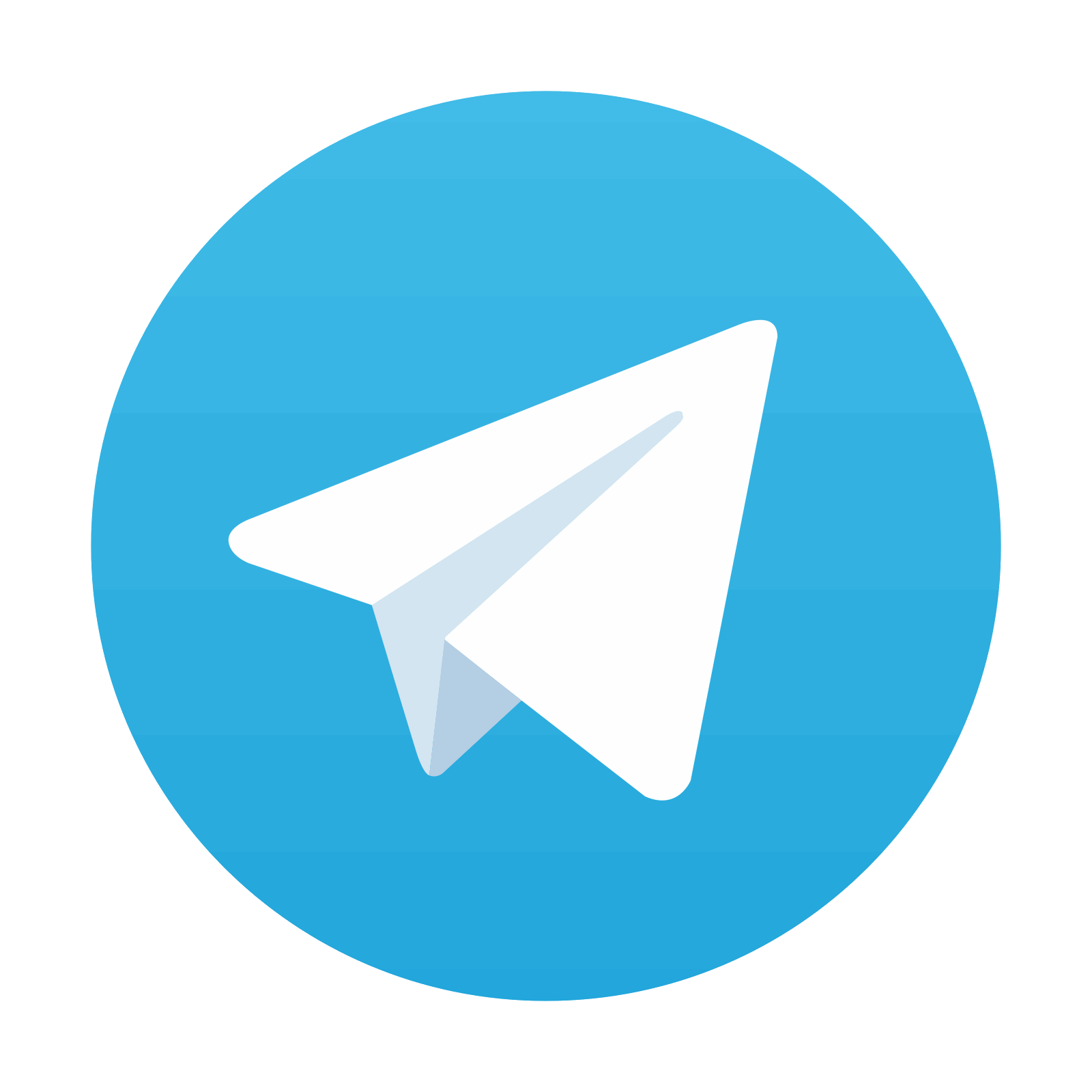
Stay updated, free articles. Join our Telegram channel

Full access? Get Clinical Tree
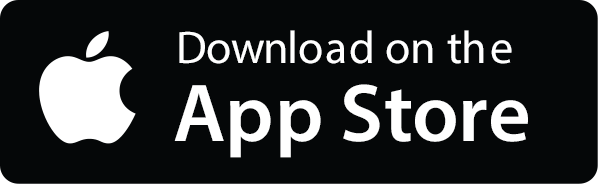
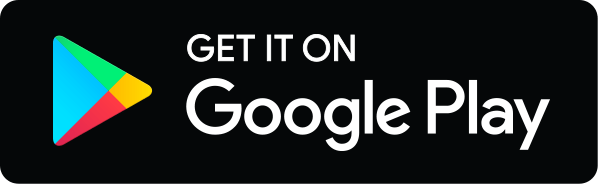