An increase of the magnetic field strength to ultrahigh-field yields advantageous as well as disadvantageous changes in physical effects. The beneficial increase in signal/noise ratio can be leveraged into higher spatiotemporal resolution, and an exacerbation of artifacts can impede ultrahigh-field imaging. With the successful introduction of intracranial and musculoskeletal imaging at 7 T, recent advances in coil design have created opportunities for further applications of ultrahigh-field magnetic resonance (MR) imaging in other parts of the body. Initial studies in 7 T neck and spine MR imaging have revealed promising insights and new challenges, demanding further research and methodological optimization.
- •
Ultrahigh-field magnetic resonance (MR) imaging of the neck and spine is an emerging promising imaging technique; the increased signal/noise ratio (SNR) at 7 T can be used to achieve higher spatiotemporal resolution imaging than at lower field strengths
- •
Initial ultrahigh-field radiofrequency (RF) coil concepts have enabled the successful demonstration of high-resolution nonenhanced MR angiography of the carotid arteries and structural imaging of the spine and parotid gland
- •
Further developments in RF coil design, pulse sequences, and shimming strategies are needed before the full diagnostic potential of ultrahigh-field MR imaging of the neck and spine can be assessed
- •
Owing to recent advancements in RF coil design and construction, 7 T MR imaging has established its initial feasibility for neck and spine applications
- •
The increase in the magnetic field strength to ultrahigh-field yields potentially advantageous as well as disadvantageous changes in physical effects
- •
The increase in SNR associated with 7 T can be transitioned into imaging at high spatial resolution, allowing for detailed anatomic depictions
- •
7 T MR imaging allows nonenhanced, high-resolution MR angiography of the carotid arteries and high-quality cross-sectional plaque assessment
- •
The exacerbation of artifacts affiliated with the increase in the magnetic field strength may impede the diagnostic quality of 7 T MR imaging and necessitates imaging sequence and parameter modification
- •
Imaging of large fields of view (FOVs) at 7 T, as is often required in spine imaging, remains challenging because of RF field inhomogeneities
- •
Specific absorption rate (SAR) limitations, especially with respect to local SAR, need particular consideration
- •
Medical implants, metallic devices in general (eg, piercings), and tattoos have to be handled with caution when scanning at ultrahigh-field strength to prevent potential harm
- •
Further optimization of imaging sequences, shim techniques, and RF coil concepts is needed to make imaging at 7 T competitive with the diagnostic ability of neck and spine imaging at low to high-field strength
Ultrahigh-field MR imaging of the neck and spine
MR imaging has evolved to become the diagnostic modality of choice for spine imaging and, because of its excellent soft tissue contrast, also the preferred diagnostic modality for neck imaging. Indications to perform MR imaging of the neck and spine range from suspected vertebral disk herniation to spinal cord tumors, as well as assessment of possible neoplastic or inflammatory disease of the cervical soft tissue.
Although 1.5 T MR systems are still considered the clinical standard, 3 T imaging has established its place in clinical diagnostics within the last few years. A further increase in field strength to 7 T has shown initial benefits for clinically oriented neuroimaging ; however, potential diagnostic benefits for extracranial imaging are yet to be shown.
This article reviews the current status of ultrahigh-field MR imaging in the neck and spine. Ultrahigh-field is defined here as imaging at more than 3 T but, because 7 T is emerging as the next standard field strength beyond 3 T, the article focuses on work at 7 T.
Increase of the magnetic field strength: benefits
The urge to increase the magnetic field strength is based on potentially beneficial physical properties. The increase of the SNR is considered one of the most desirable physical effects associated with the increase of the field strength, because the higher SNR can be transformed into imaging at higher spatial or temporal resolution, or both. Various ultrahigh-field studies, mainly neuroskeletal and musculoskeletal MR imaging, have shown the successful transformation of the associated higher SNR into a higher spatiotemporal resolution, enabling an improvement in the assessment of anatomic details as well as increased accuracy for the depiction of pathologic findings.
Increase of the magnetic field strength: benefits
The urge to increase the magnetic field strength is based on potentially beneficial physical properties. The increase of the SNR is considered one of the most desirable physical effects associated with the increase of the field strength, because the higher SNR can be transformed into imaging at higher spatial or temporal resolution, or both. Various ultrahigh-field studies, mainly neuroskeletal and musculoskeletal MR imaging, have shown the successful transformation of the associated higher SNR into a higher spatiotemporal resolution, enabling an improvement in the assessment of anatomic details as well as increased accuracy for the depiction of pathologic findings.
Increase of the magnetic field strength: challenges
Despite the anticipated gain in SNR, an increase in the magnetic field strength is also accompanied by various physical effects that can lead to an impairment of image quality and confound the expected advantages. Apart from an increase of magnetic susceptibility and chemical shift artifacts, RF field alterations and increased deposition of RF energy are considered major disadvantages of high-field MR imaging.
The energy deposition in tissue, measured by the SAR, scales with the square of the magnetic field, with the square of the flip angle, and is proportional to the duty cycle of the RF pulse, which is particularly problematic for the adequate acquisition of RF-intense turbo-spin-echo (TSE) and fat-saturated sequences. To mitigate the associated SAR increase and preclude potentially harmful heating of the human body, parallel imaging can be applied to reduce the number of RF excitations, or various flip angle modulation techniques can be used. However, the application of parallel imaging as well as the reduction of flip angles may result in an SNR penalty.
With increasing field strength, maintenance of the homogeneity of the RF magnetic field also becomes more challenging. The wave frequency scales linearly with increasing magnetic strength, leading to a shortening of the RF wavelengths applied for tissue excitation (53 cm at 1.5 T, 27 cm at 3 T, 14–15 cm at 7 T), which may result in alterations of the RF energy distribution in tissue and so-called B 1 inhomogeneity, which is particularly pronounced in high-field body applications when a large FOV (eg, abdomen) is imaged. Hence, ultrahigh-field body imaging requires multichannel RF transmit systems that enable RF shimming. One example of an add-on system for RF shimming consists of an RF amplifier with 8 individual modules, modified to split the excitation signal of the conventional single-channel system into 8 independent channels. Optimized sets of amplitudes and phase shifts thus enable more uniform excitation of specific body regions, as shown in recent ultrahigh-field abdominal studies.
Notwithstanding these challenges, the exacerbation of certain artifacts caused by an increase in field strength may also be useful for diagnostics. Magnetic susceptibility effects are known to increase with increasing field strength, resulting in image distortion and signal loss at interfaces of soft tissue and bowel gas in abdominal MR imaging. However, increased sensitivity to susceptibility differences may also improve the conspicuity of possible microbleeds in the brain, because paramagnetic substances such as hemosiderin may be superiorly detected at higher magnetic field strength.
Ultrahigh-field spine imaging
MR imaging of the spine benefits from an increase of the magnetic field strength from 1.5 T to 3 T, resulting in an improved delineation of soft tissue, cerebrospinal fluid, vertebral discs, and bone interfaces. In a comparison study in the spine in patients with isolated syndrome suggesting multiple sclerosis, the imaging results revealed an improved diagnostic accuracy for 3 T MR imaging. With the benefits of increased field strength being shown for 3 T spinal cord imaging, even higher field strengths such as 7 T and greater may be able to improve the diagnostic accuracy, or may even may be able to provide earlier diagnosis of spinal disease.
Initially hampered by the lack of dedicated RF coils, recent advancements in RF coil design have created opportunities for scientific research in 7 T spine imaging. In an initial technical approach Wu and colleagues designed and built transceiver arrays with coil geometries of different coil size and number. Their preliminary results showed the feasibility of in vivo human spine imaging using a microstrip loop design with adjustable inductive decoupling, offering large image coverage and satisfactory B 1 penetration and parallel imaging performance.
Using an alternative design approach, Kraff and colleagues recently introduced a prototype of a custom-built 8-channel transmit coil for spine imaging at 7 T ( Fig. 1 ). The combined transmit/receive RF array is constructed of 8 square surface loop coils, with neighboring elements being overlapped to decrease the mutual inductance. The assembled spine array is connected to a set of 8 preamplifiers and T/R switches to provide controlled independent tissue excitation for maximum excitation uniformity and coverage. Imaging results revealed a reasonably homogeneous excitation along the spine for a coverage of a 40-cm FOV, providing good delineation of anatomic details including the vertebral bodies, longitudinal ligaments, and the venous drainage through the vertebrae ( Fig. 2 ). Residual signal alterations caused by B 1 inhomogeneity and inconsistent contrast between cerebrospinal fluid and myelin (caused by flip angle variations) were noted as impairing limitations.
This combined transmit/receive RF spine array was used by Grams and colleagues to perform a clinical study to evaluate the feasibility of common clinical sequences for imaging of the lumbar spine at ultrahigh-field strength. After sequence adjustments and modifications for 7 T, T2-weighted (T2w) TSE, three-dimensional (3D) double-echo steady-state (DESS), 3D constructive interference in steady-state (CISS), and 3D volumetric interpolated breath hold examination (VIBE) sequences were acquired. T2w TSE imaging in 5 healthy volunteers was strongly limited in its penetration depth, yielding sufficient signal homogeneity in only 2 slim volunteers. Hence, qualitative image analysis of T2w TSE imaging was rated as poor. In contrast, 3D VIBE MR imaging provided the best imaging results, enabling the highest spatial resolution with 0.57 isotropic imaging. Gradient-echo sequences are generally less susceptible to flip angle variations than spin-echo sequences. Nevertheless, VIBE imaging also showed limitations in terms of signal loss in portions of the spine more distant from the coil, as well as an impaired delineation of the caudal fibers and the intraforaminal structures. 3D CISS and 3D DESS MR imaging yielded comparable imaging results in terms of anatomic display, with slightly better conspicuity of intraforaminal structures in CISS imaging and slightly better conspicuity of the facet joints in DESS MR imaging. In terms of quantitative image analysis, T2w TSE imaging achieved the highest mean values of all the assessed sequences for the contrast ratio between the cerebrospinal fluid and the vertebral body, as well as between the cerebrospinal fluid and the spinal cord. The best contrast ratio between vertebral disc and body was rated for 3D CISS MR imaging. Despite these promising initial imaging results of ultrahigh-field spine imaging, none of the assessed sequences, all of which are established in 1.5 T and 3 T clinical imaging, could provide sufficient conspicuity of all assessed anatomic structures or compete with the diagnostic ability of clinical spine imaging at low to high-field strength. Hence, the routine clinical application of ultrahigh-field spine MR imaging is not yet possible.
Vossen and colleagues examined ultrahigh-field spine imaging for assessing the complete human spinal column within an acceptable scanning time. For image acquisition, they used a dedicated quadrature transmit, 8-channel receive array RF coil. Large FOV scanning allowed sagittal spine imaging in 2 or 3 stations within a total scan time of 10 to 15 minutes. In addition to initial clinical findings such as signs of osteochondrosis as well as small dislocations in the disks, their study results also revealed the efficiency of using an anterior coil for transmission of RF energy combined with a posterior receive array.
In conclusion, these first approaches toward dedicated 7 T spine MR imaging reveal the potential benefits as well as challenges of ultrahigh-field spine imaging. Further optimization of RF sequences, shim techniques, and dedicated RF coil concepts are expected to better cope with the physical effects affiliated with high magnetic field strength, enabling clinically oriented studies at 7 T. The first commercial RF coils for the spine are becoming available and include a dedicated cervical spine coil that is constructed in a 2 × 2 channel arrangement and is compatible with MR systems with both a single transmitter or 4 individual transmitters for parallel excitation applications such as Transmit SENSE.
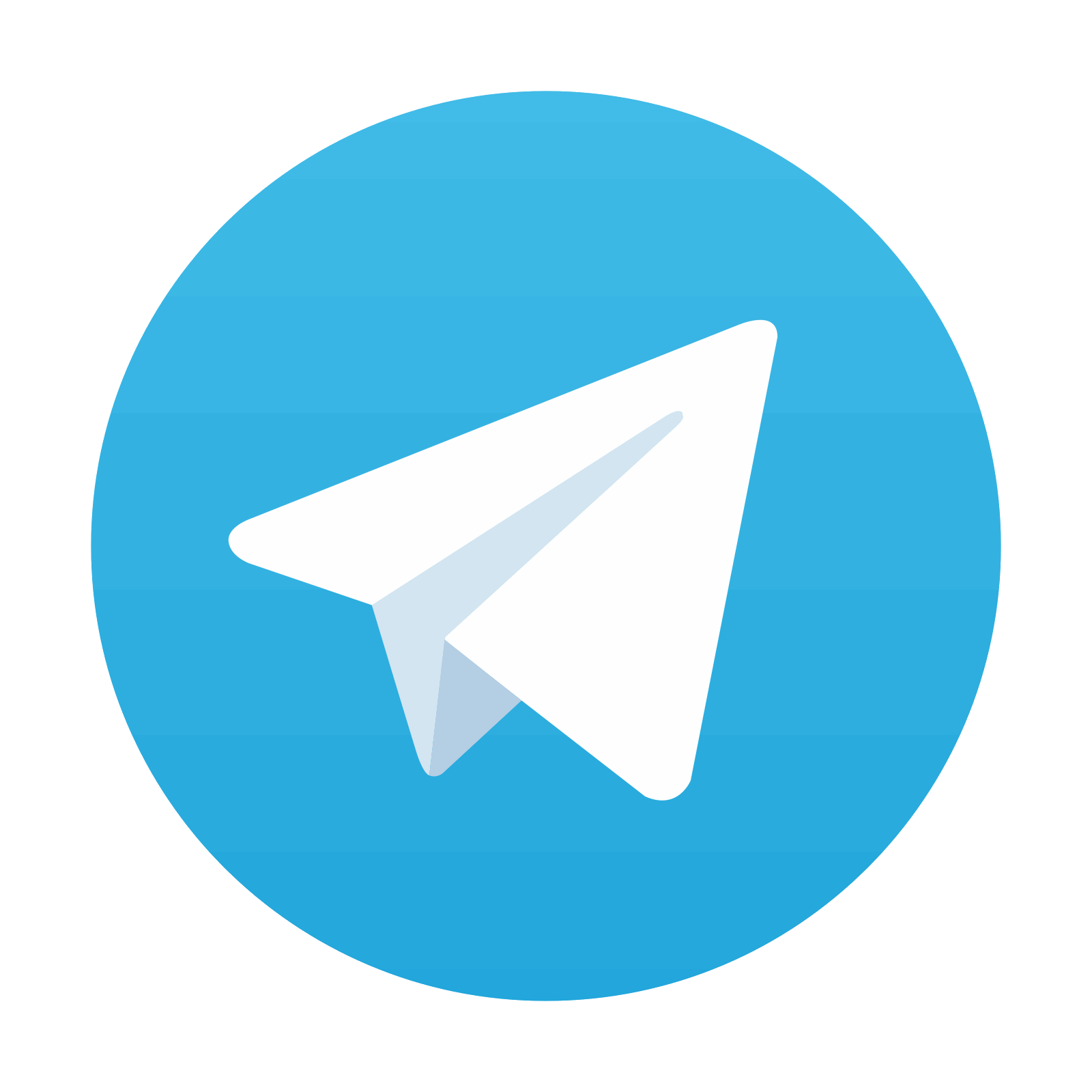
Stay updated, free articles. Join our Telegram channel

Full access? Get Clinical Tree
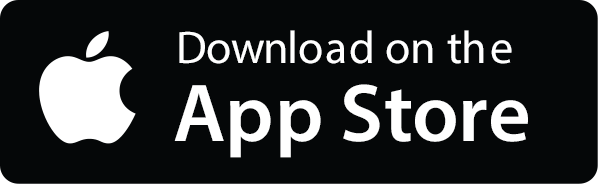
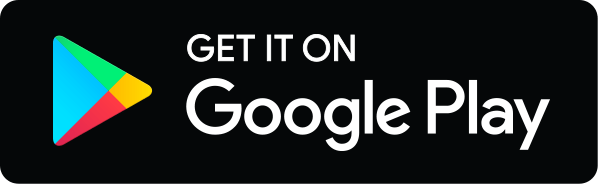