Computed tomography angiography (CTA) has become a mainstay for the imaging of vascular diseases, because of high accuracy, availability, and rapid turnaround time. High-quality CTA images can now be routinely obtained with high isotropic spatial resolution and temporal resolution. Advances in CTA have focused on improving the image quality, increasing the acquisition speed, eliminating artifacts, and reducing the doses of radiation and iodinated contrast media. Dual-energy computed tomography provides material composition capabilities that can be used for characterizing lesions, optimizing contrast, decreasing artifact, and reducing radiation dose. Deep learning techniques can be used for classification, segmentation, quantification, and image enhancement.
Key points
- •
Computed tomography (CT) is a highly accurate technique in the evaluation of vascular disease.
- •
Recent advances in technology enable acquisition of images at high spatial resolution with low artifacts, radiation dose, and contrast.
- •
Using dual energy CT and photon counting CT, material composition can be characterized.
Introduction
Computed tomography angiography (CTA) has evolved into a vital noninvasive imaging technique in the evaluation of vascular diseases. CTA relies on optimal contrast opacification of the vasculature of interest following the injection of an appropriate volume of iodinated contrast medium at high flow rates. The advantages of computed tomography (CT) include its near-universal availability, rapid turnaround time, and high accuracy in the evaluation of vascular disease because of its isotropic submillimeter spatial resolution, multiplanar reconstruction capabilities, wide field of view, and excellent temporal resolution. The use of ionizing radiation and iodinated contrast media are the concerns associated with the use of CT. There is no conclusive evidence to link the low-dose radiation of CT with carcinogenesis; however, the linear no-threshold model, which implies that no radiation dose is safe and the risk progressively increases with the radiation dose, has gained credibility. Hence, it is imperative use the lowest possible radiation dose without compromising on image quality. Iodinated contrast material was thought to be associated with the development of contrast-induced nephropathy in patients with risk factors and amplification of the DNA radiation damage by CT. , Recent evidence has shown the absence of any association between the intravenous CT contrast material and acute kidney injury, even in the presence of predisposing comorbidities, questioning the need for prophylactic measures such as volume expansion and decreasing dose of contrast.
Recent advances in CTA have focused on improving the image quality, increasing the acquisition speed, eliminating artifacts, providing tissue/material characterization, and reducing the dose of radiation and iodinated contrast. This article reviews the current state of the art in vascular CT.
Scan technology
Multidetector CT scanners are the now the norm in vascular imaging, with current scanners ranging from 4 to 320 detector rows. The wide-array or volume CT scanners have a large z-coverage of up to 16 cm. The entire heart can be imaged in 1 R-R interval, which minimizes motion artifacts from cardiac arrhythmias. The entire body can be scanned in a few seconds, as a result of which motion artifacts can be minimized. Because contrast is required for only a short period of time, the total contrast volume injection is also reduced. Radiation doses are also lower with these scanners. With the use of electrocardiogram (ECG) gating, motion-free images of the coronary arteries can also be obtained in the same study. One study on a 320-volume CT scanner used prospective ECG triggering without medications (acquisition at 40%–50% R-R interval for HR ≥70 beats/min [bpm] and at 70%–80% R-R interval for HR <70 bpm) to obtain motion-free images of the aortic root, aortic valve, and the coronary arteries, with lower radiation doses.
Ultrafast high-pitch helical mode (flash mode) is one of the several acquisition modes available with the latest generation of dual-source scanners. All these modes can be used either with or without ECG gating. Both the x-ray tubes can be operated at the same energy, which can achieve temporal resolution of up to 66 milliseconds with ECG gating, allowing generation of motion-free images of the aortic root and coronary arteries. Alternatively, the x-ray tubes can be operated at different energy levels (discussed later), providing dual-energy information. With the high-pitch helical mode, patients can be scanned at pitches of up to 3.4 without artifacts because of filling of data gaps by the data from the other x-ray tube. Because of the ultrafast acquisition, the entire aorta can be scanned in just 2 seconds , ( Fig. 1 ). The absence of motion artifacts caused by such rapid scanning is beneficial in patients who cannot comply with breath-hold instructions, particularly in the emergency room and inpatient settings. The rapid scanning obviates sedation/anesthesia in the pediatric population. Multiple vascular beds, such as the aorta and coronary arteries or heart, head, and neck, can be scanned rapidly in 1 acquisition, saving radiation and contrast doses. ,
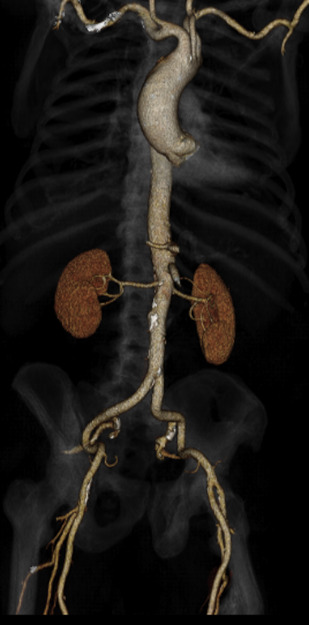
The image quality of these scans is higher than that of conventional CTA with less motion artifact and similar vascular attenuation. Flash mode can be performed either without ECG gating or with prospective ECG triggering. The quality of both these modes has been shown to be comparable. , , Motion-free images of the aortic root can be obtained even without ECG gating. , The addition of ECG gating did not substantially reduce motion artifacts in children. Motion-free images of adjacent structures such as heart and pulmonary arteries can also be obtained using the flash mode, although the contrast bolus timing may be an issue with such a rapid acquisition. Motion-free images of the coronary arteries can also be obtained in the same study if the heart rate and variability are low (HR<63 bpm; variability<1.2 bpm) (12) ( Fig. 2 ).
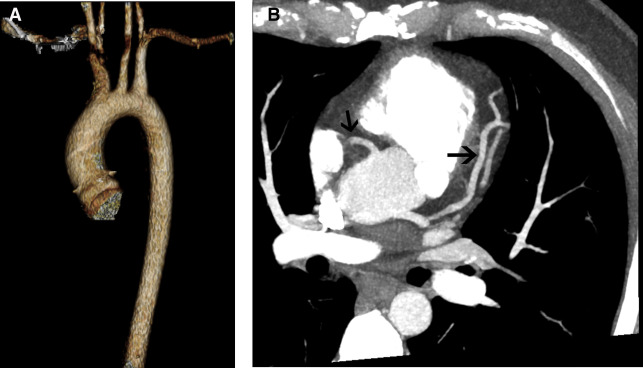
Radiation doses are lower with the flash mode because of decreased overlapping projection data. , Radiation doses are lower than conventional CTA by 50% to 72% for imaging the entire aorta. Contrast dose is also lower with this mode, because arterial contrast is required only for the small duration of scanning. High-pitch helical mode along with 70-kVp tube voltage and iterative reconstruction can achieve radiation dose savings of 81% and contrast-dose savings of 33% with the same image quality of a 120-kVp scan. , Good-quality images of the renal arteries have been acquired using high-pitch helical mode with 51% contrast dose and 38% radiation dose reduction. Transcatheter aortic valve replacement (TAVR) CTA of the chest, abdomen, and pelvis has been performed by using only 20 mL of contrast. ,
Higher image noise is a challenge of high-pitch helical mode because of the presence of fewer redundant projection values to average. Even with higher noise, the subjective image quality is comparable with a standard-pitch mode if good contrast opacification is achieved. One study showed poorer image quality for abdominal than thoracic aorta, whereas other studies have shown good quality even for abdominal aorta. , Noise is a significant challenge in morbidly obese individuals and with low-voltage techniques because of tube saturation. Noise can be reduced by using iterative reconstruction techniques. ECG-gated flash acquisition requires a slow (<70 bpm) and steady heart rate and does not provide functional information because data are gathered from a single cardiac phase with the prospective ECG technique. In some instances, the contrast bolus may be out-run because of the rapid acquisition. Interpolation artifacts may be seen in the earlier generations of dual-source scanners with smaller x-ray detectors. Lower spatial resolution, helical artifacts, and degraded section sensitivity profiles were also challenges associated with the earlier-generation scanners.
Low-tube-voltage ct
Vascular CT is typically performed at tube voltage of 120 to 140 kVp. Scanning at lower tube voltages (<120 kVp) has the advantage of lower radiation dose because of lower photon flux. However, the lower photon flux increases the image noise, necessitating compensatory increase in tube currents up to 1500 mAs. Hence, this technique is generally limited to children and those with lean body habitus, and is typically avoided in obese individuals. Iterative reconstruction algorithms can also be used to maintain the image quality by decreasing the noise. Another advantage of low-kVp scanning is the higher signal of contrast caused by higher photoelectric attenuation of iodine as the x-ray energy approximates the k-edge of iodine, which is 33.2 keV. The mean energy of x-ray photons with peak voltage of 80 kVp is 43.7 keV and with peak voltage of 73 kVp is 33.2 keV.
Radiation and contrast-dose savings with maintained image quality have been shown in several CTA studies using tube voltage as low as 70 kVp ( Fig. 3 ). A study on 256-slice CT with 100 kVp and 30 mL of iodinated contrast achieved 70% reduction in radiation dose with diagnostic performance comparable with a standard CT. Aortoiliac CTA can be performed at 90 kVp with 60% lower contrast and similar vascular enhancement as a 120-kVp study. At 80 kVp, radiation dose savings of 65% and contrast-dose savings of 30% can be obtained. The 80-kVp scans of dual-energy CT also have improved vascular attenuation, diagnostic performance, and salvage of suboptimal studies compared with the mixed images, albeit at a higher noise. , Lower-extremity CTA at 70 kVp at comparable diagnostic performance can be obtained with less than half the radiation dose and lower contrast volume of 1.2 mL/kg. Lower-extremity CTA using 70-kVp, high-pitch acquisition and iterative reconstruction can achieve 81% radiation dose reduction and 33% contrast volume reduction. Another dual-source CTA study also used 70 kVp and 80 mAs with 30 mL of contrast following timing bolus to generate good-quality images in peripheral arterial disease. Automatic selection of tube potential and contrast volume based on weight can achieve contrast-dose savings of 20% without compromising image quality. Another similar study that used automated attenuation-based tube voltage selection (70–150 kVp) and individualized low-volume contrast media protocols showed good quality for CTA of the aorta irrespective of the tube voltage.
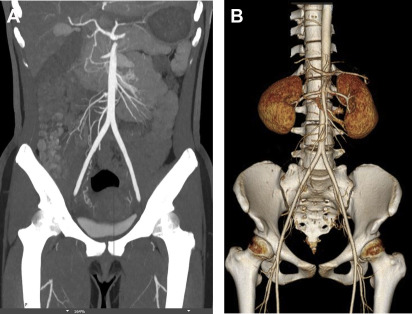
Dual-energy ct
Dual-energy CT (DECT) uses the differential attenuation properties of tissues/materials at different energy levels to separate them beyond that possible with a conventional CT. By calculating the attenuation of a voxel at 2 different energies and the prior knowledge of the attenuation coefficients of 2 or 3 dominant materials, the contribution of different tissues to each voxel can be estimated (2-material or 3-material decomposition). Dual-source and rapid kilovoltage-peak switching are the commonly used x-ray source-based technologies, whereas dual layer is the commercially available detector-based DECT technology. In addition to conventional images, DECT generates images such as virtual monoenergetic imaging (VMI), iodine maps, virtual noncontrast (VNC), and effective atomic number–based images.
VMI mimics CT images obtained with a monoenergetic x-ray beam. In dual-energy CT, VMI can be generated from 40 to 200 keV (kiloelectron volts) by a process of linear combination of basis pair images at different ratios. VMI at 70 keV has the same attenuation as a conventional 120-kVp image, but with lower noise and artifacts. VMI at low energy levels (<70 keV) shows higher signal of iodinated contrast because of higher photoelectric effect closer to the k-edge of iodine. This property is advantageous in reducing the load of iodinated contrast ( Fig. 4 ), salvaging suboptimal enhanced studies, generating CTA-quality images from a routine CT scan ( Fig. 5 ), improving visualization of side branches, and improving lesion conspicuity. The optimal VMI energy for boosting contrast can range from 40 to 60 keV, depending on the vessel and vendor, with some scanners having higher noise at low energy levels, whereas others have consistently lower noise. For example, 1 study with dual-layer CT found 50 keV to be the optimal energy level for aortic CTA with higher attenuation (by 91%), contrast/noise ratio (CNR) (by 85%), and signal/noise ratio (108%) than conventional 120-kVp level, salvaging all suboptimal enhanced studies. Low-energy VMI has been used in several low-contrast-dose studies in different vascular beds, with contrast material doses as low as 25 mL , iodine concentration as low as 15 g and contrast material reductions of up to 70% with maintained image quality and CNR. Improved visualization of small branch vessels such as bronchial, intercostal, and vertebral arteries has been shown with low-energy VMI. The detection of leaks in perigraft space of inclusion grafts and endoleaks has been shown to be improved with the use of low-energy VMI, , even in routine non-CTA scans.
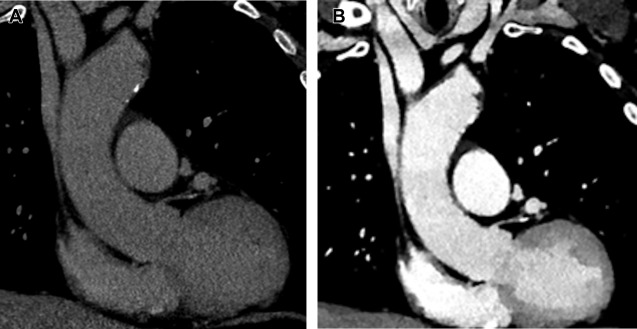
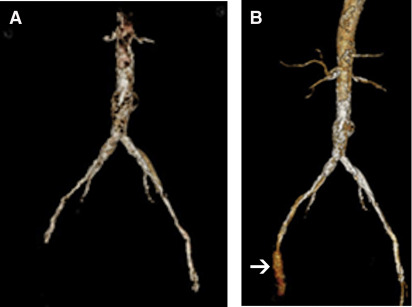
Higher-energy VMI (>70 keV) can be used to decrease several artifacts, such as beam hardening, metallic artifact, and calcium blooming ( Fig. 6 ). VMI ≥ 90 keV has significantly lower hypoattenuating and hyperattenuating beam hardening artifacts from high-density contrast material in axillary and subclavian veins. The best results for diagnostic assessment were provided at 130 keV with overcorrection of artifacts observed at higher keV values.
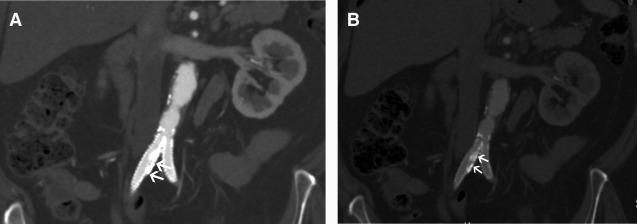
Iodine maps are obtained by a 3-material decomposition of iodine, soft tissue, and Iodine maps can be displayed either in gray scale or color overlay over VNC images. It provides an objective measurement of contrast enhancement (in milligrams per milliliter), unrelated to Hounsfield units (HU). Iodine maps can be used to distinguish high-attenuation lesions in contrast CT as contrast, hemorrhage, or calcification ( Fig. 7 ). Only contrast enhancement is associated with higher iodine uptake. Iodine maps can also help in characterizing masses, particularly thrombus, which does not show significant iodine uptake. One study on a rapid kilovoltage-peak switching scanner showed 1.74 mg/mL as the cutoff for distinguishing thrombus from a contrast admixture artifact in left atrial appendage, but this number varies with the scanner and organ. Iodine maps are used for evaluating parenchymal perfusion, particularly in the heart and lungs. In the heart, iodine maps can be used for detecting myocardial ischemia with higher sensitivity and specificity than conventional CTA. Iodine maps or derivatives, such as pulmonary blood volume maps, can be used to improve the diagnosis and provide prognostic information in acute pulmonary embolism, chronic pulmonary embolism, and chronic thromboembolic pulmonary hypertension. Color iodine map overlays from venous-phase imaging increase the detection of subtle endoleaks with 100% accuracy, while saving 28% radiation dose compared with single-energy biphasic studies ( Fig. 8 ). Iodine maps also have lower artifacts from high-atomic-number metals, such as platinum embolization coils.
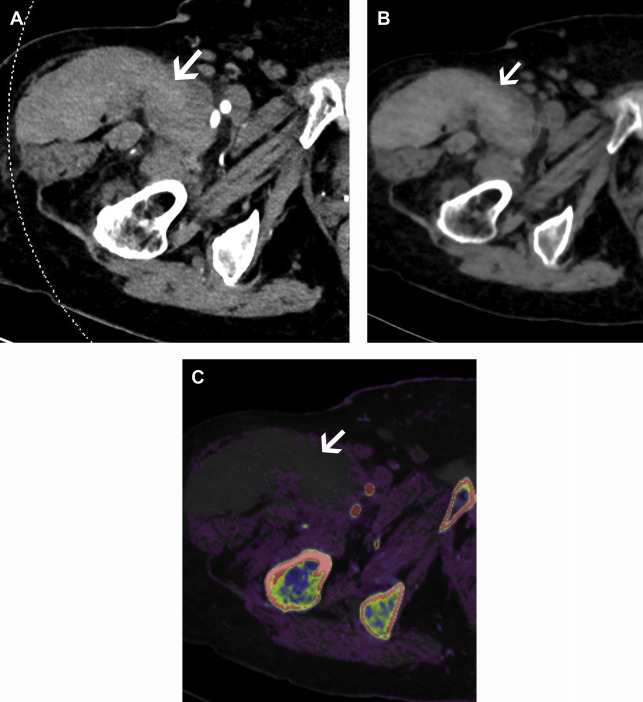
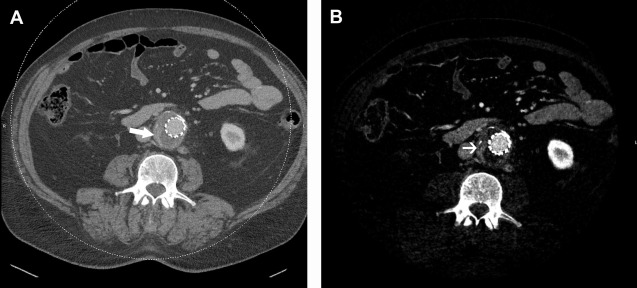
Virtual noncontrast (VNC) images are derived from the same 3-material decomposition process as the iodine map, but with subtraction of pixels containing iodine. Because the VNC images have similar attenuation and noise , they can replace the true non contrast (TNC) images of a multiphasic vascular CT protocol, achieving 50% radiation-dose savings. , , Image quality is not compromised, although some of the calcium can also be removed because it is not one of the 3 basis materials. , Further radiation dose reduction of up to 68% can be accomplished by acquiring only the delayed venous-phase images and generating virtual arterial-phase reconstructions, either from 40-keV low-energy VMI or from the low energy acquisition (80 kVp) in a dual-source scanner ( Fig. 9 ).This technique has noninferior image quality and high diagnostic performance in the detection of endoleak (96%–100% sensitivity, specificity, accuracy) compared with true arterial-phase images. , , VNC either on its own or along with iodine map can be used to characterize high-attenuation lesions encountered in contrast CT. Hemorrhage is seen in VNC but not in the iodine map, whereas extravasated contrast is seen in the iodine map but not in VNC (see Fig. 7 ). Similarly, VNC and iodine map can be used to detect and localize gastrointestinal bleeding with high diagnostic performance (area under the curve, 0.94) and 30% radiation dose savings compared with a triple-phase protocol.
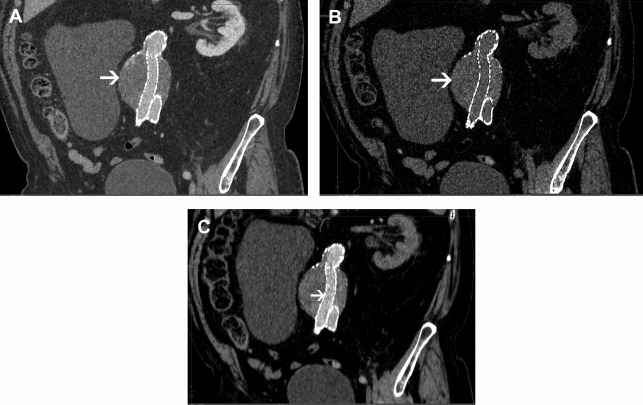
CTA has high accuracy in the evaluation of peripheral arterial disease. , This evaluation requires good-quality maximum intensity projections (MIPs) and three-dimensional (3D) reconstructions without bones. The conventional threshold-based bone subtraction techniques are time consuming and may erode vessels adjacent to bones, resulting in overestimation of luminal stenosis or mimicking of an occlusion. DECT can generate bone-subtracted images using 3-material decomposition of calcium, iodine, and blood or 2-material decomposition of calcium and iodine. The voxels with bone are assigned a low attenuation value (ie, −1024 HU) as a result of which bone-free MIP and 3D volume rendering (VR) images can be easily generated ( Fig. 10 ). DECT bone subtraction takes substantially less time and is more robust than conventional techniques. , DECT MIPs are superior to single-energy bone-subtracted MIPs (sensitivity, 97.2% vs 77.1%; specificity, 94.1% vs 70.7%; accuracy, 94.7% vs 72.0%), with less frequent erosion of vessels adjacent to the bone. The performance is better in the aortoiliac and femoropopliteal regions as well as bypass grafts, but moderate at the knee and poor in the calf and pedal arteries, especially in critical limb ischemia. , With severely calcified vessels, DECT MIPs performed better than single-energy MIPs (91% to 96% vs 57% to 74%). Incomplete bone subtraction may be seen in areas outside the central field of view (FOV) of the scanner, which can be manually subtracted. Some calcified plaques can also be removed as bone. , The resolution can be improved by isocenter positioning and regional batches.
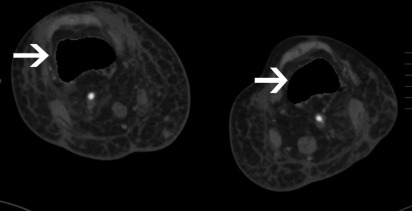
In conventional CTA, calcified plaque cannot be removed and hence the MIPs are not accurate in grading the stenosis. Often an additional curved multiplanar reformation image has to be generated to accurately quantify luminal stenosis. With DECT, calcified plaques can be removed using morphologic criteria to separate plaque and bone ( Fig. 11 ), from which a CTA luminogram MIP can be generated, which saves additional postprocessing ( Fig. 12 ). Similar to bone-subtracted DECT images, calcified plaque subtraction is better for the pelvic and thigh arteries, especially for heavily calcified plaques in the larger vessels (>5 mm). Over-removal of plaque may result in overestimation of luminal stenosis. The diagnostic performance of bone subtraction plus plaque removal was shown to be slightly lower than DECT bone subtraction alone (sensitivity, 84%; specificity, 56%; accuracy, 75%), , with better results at the aortoiliac level. Hence the findings should always be correlated with axial source images. Recently a modified 3-material decomposition algorithm with calcium as the primary material has been developed with complete and selective calcium subtraction. These datasets have higher CNR and higher accuracy (96.5% vs 93.1%) with comparable image quality. This technique can potentially decrease inconclusive and false-positive CTA results in heavily calcified vessels.
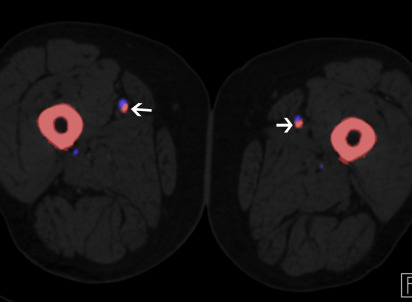
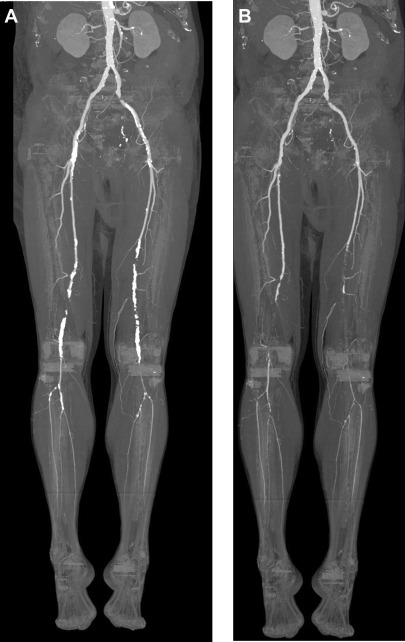
DECT has been shown to be faster and superior to conventional bone subtraction in thorax, which often requires multiple clip planes in addition to threshold or region growing techniques. Small branch vessels, such as intercostal arteries, are not eroded with DECT bone subtraction. The entire thorax may not fit into the smaller x-ray tube FOV, but, with the latest generation of scanners, this is not an issue anymore. Advanced algorithms can be used to separate intravascular iodinated material from simultaneously administered high-atomic-number contrast media such as oral tantalum, tungsten, or rhenium contrast, with the tantalum appearing in iodine-only images and the rhenium in water-equivalent images. DECT is still not widely available, involves additional cost, and may need new protocols for most of the scanners. Postprocessing may take additional time but can be automated and performed at the scanner. With the dual-source scanners, there is a size limitation, because one of the x-ray detectors is smaller. There are higher beam hardening/streak artifacts on material-specific images in rapid kilovoltage-peak switching.
Photon-counting computed tomography
Photon-counting CT is an emerging technology with a semiconductor detector that converts the incident x-ray photons directly into positive and negative charge clouds. These clouds are pulled in opposite directions to generate an electrical pulse, whose height is proportional to the energy of the x-ray photon. The x-ray spectrum can be divided into several specific energy bins, albeit with some overlap. Energy weighting is a technique in which more weight is assigned to 1 specific energy bin to highlight a specific tissue. Material decomposition can be achieved from energy-selective images and generation of basis image maps from 2 or 3 energy bins. Because of energy weighting, the accuracy of detection of contrast agents can be increased. K-edge imaging allows the measurement of the concentration of specific elements. Simultaneous multicontrast agent imaging is possible because of different k-edges allowing differentiation of multiple contrast agents, such as iodine or gadolinium with nanoparticles There is also potential for novel contrast agents with high atomic numbers such as gold, platinum, xenon, bismuth, lutetium, tungsten, silver, and ytterbium because of higher spectral separation. Molecular CT imaging with targeted contrast agents is also possible.
In addition, the CNR is higher in photon detector CT because of lower electronic noise and higher weighting of low-energy bins. This technique has the potential to reduce the radiation and contrast doses. , Photon detector CT scans also have high spatial resolution up to 0.07 × 0.07 mm 3 because of smaller detector elements and magnification factor. The high spatial resolution is useful in the evaluation of small vascular structures and their disorders, such as vasculitis. The high spatial resolution along with material decomposition also has the potential to reduce calcium blooming and provide an accurate estimation of the severity of luminal stenosis, thus increasing the specificity of CT. This potential is particularly relevant in patients with critical limb ischemia for evaluation of calcified arteries below the level of knee. Other artifacts, such as beam hardening and metal, can also be reduced by using high-energy bins. Photon-counting CT is still an experimental technology and is not commercially available, with only a few prototype scanners installed at select institutions.
Ultrahigh spatial resolution
High spatial resolution is beneficial in evaluating small vessels and elucidating fine details. High spatial resolution can also minimize or eliminate artifacts such as blooming from high-density structures such as calcium and metallic stents. The smallest clinically available detector element size, and hence the spatial resolution, is 0.5 mm, which is limited in evaluating small vessels (<3 mm) and vessels with calcium/metallic stents. Prototype ultrahigh-resolution (UHR) scanners are now available with up to 0.25 × 0.25-mm detector element size, 1792 detector channels, and small x-ray focus size of 0.4 × 0.5 mm, which allows a reconstruction matrix of 2048 × 2048 or 1024 × 1024 and slice thickness of 0.25 mm.
UHR CT will be useful in critical limb ischemia for the visualization of infrapopliteal and pedal arteries. UHR CT provides more detailed visualization of the distal vasculature, including digital arteries, pedal arch, and collateral vessels, than conventional CT. This information is important for interventional procedures below the knee, such as transmetatarsal retrograde puncture, which may be difficult to achieve even with invasive angiography. In a phantom study, UHR CT achieved twice the spatial resolution of conventional CT (29 line pairs per centimeter at 2% modulation transfer function vs 14 line pairs per centimeter for conventional CT), and yielded improved accuracy for evaluation of in-stent restenosis in a phantom study of renal artery stents. UHR CT also decreases blooming artifacts from calcium and stent, which improves the diagnostic accuracy of CTA compared with digital subtraction angiography (DSA). With subtraction imaging of noncontrast from contrast images following orbital helical scanning, volume position matching, and zero clipping, calcium and stent can be removed. UHR CT has also shown improved visualization of the entire course of the artery of Adamkiewicz from the aorta to the anterior spinal artery, which typically measures only 1 mm ( Fig. 13 ). Future developments may include evaluation of tissue perfusion. The use of thinner sections may lead to higher radiation dose without the use of other techniques such as iterative reconstruction. Higher processing power, larger storage, and faster network traffic are required to manage the additional images generated because of thin slices.
