Vascular Anatomy and Microanatomy
Farhood Saremi, MD
Cameron Hassani, MD
Ameera Ismail, MD
▪ Introduction
Normal function of every organ in the body depends on the integrity of macro- and microcirculation. The blood flow regulation is based on the specific needs of an organ as long as the arterial pressure is sufficient to maintain adequate tissue perfusion. In general, each artery entering an organ branches six to eight times before becoming arterioles (10 to 15 µm). To reach to the capillary level, with diameters of approximately 9 µm, two to five more branchings occur.1 The term “microcirculation” is applied to the vessels less than 200 µm in diameter, including arterioles, capillaries, and venules, that are responsible for the distribution of blood, transportation of oxygen and nutrients, and removal of toxins within tissues. The abnormalities in the microcirculation play an important role in the start and progression of several diseases. Depending on the severity and duration of the tissue blood flow disturbances, alterations in the microcirculation structure may take place causing permanent modifications in the vessel morphology, a process known as “vascular remodeling.”1 It is shown that sustained vasoconstriction may lead to a decrease in the number and density of working small vessels. This phenomenon is called “functional rarefaction,” which may promote permanent vascular regression and loss also termed “structural rarefaction.” In turn, microvessel regression may further trigger the vicious circle of arterial remodeling, constriction, and rarefaction leading to further tissue injury.
Both CT and MR angiographies are great techniques to evaluate the macrovasculature of different organs. Given the limited spatial resolution of diagnostic imaging techniques, direct visualization of the microvasculature is not feasible in clinical practice, and most clinical imaging studies of the microvasculature revolve around indirect analysis of tissue perfusion. Microangiography using monochromatic synchrotron radiation has recently been used in animal studies for the assessment of the microvasculature in different organs such as the heart, brain, liver, kidneys, and lungs.2,3,4 and 5 Although fluorescence microscopy has also been used to image small arteries for a long time, it does not show microvessels after they enter the parenchyma of an organ. High-resolution monochromatic synchrotron microimaging system allows simultaneous observations of up to four levels of branching of arteries/arterioles from approximately 30 to 400 µm in diameter and provides measurements of vascular diameters that are minimally affected by the relative distance or orientation of individual vessels to the camera or x-ray source. The vessel detection limit of the system is 10 µm.4 However, synchrotron radiation technique is invasive and requires insertion of a catheter and injection of contrast media into the arterial system to achieve sufficient contrast of the blood vessels. New generation of micro-CT systems use a cone-beam x-ray source equipped with a multifocus x-ray tube allowing ultrafast in vivo CT angiography of small vessels at resolutions down to 16 µm.6
▪ Brain Circulation
Brain perfusion imaging provides physiologic information, which goes beyond the anatomic imaging. It has an established role in acute stroke imaging, tumor staging, and its response to treatment, and developing role in demyelinating disorders and seizures. It is important to be familiar with the normal gross vascular and microvascular anatomy of the brain in order to master interpreting brain perfusion imaging. In this section, brain vascular anatomy, anastomotic network, and normal variations are briefly discussed.
▪ Brain Arteries
The arterial supply of the brain is divided into anterior and posterior circulations, which have rich anastomosis together7,8,9 and 10 (Fig. 1.1). The anterior circulation is derived from the internal carotid arteries and their branches. The posterior circulation is derived from the vertebrobasilar arteries and their branches. The arterial supply to the brain also anastomoses with external carotid, which supplies the scalp and calvarium. Anatomic variations are common and usually asymptomatic. Arterial fenestration is more common in the vertebrobasilar arteries. An association has been observed between fenestration and aneurysm formation.10
Anterior Circulation
Internal Carotid Artery
The internal carotid artery has six intracranial segments: the petrous segment (C2), lacerum segment (C3), cavernous segment (C4), clinoid segment (C5), supraclinoid segment “ophthalmic” (C6), and terminus segment (C7).
The internal carotid artery gives rise to the ophthalmic artery, the anterior choroidal artery, and the posterior communicating
artery before it terminates into its two terminal branches: the anterior cerebral artery (ACA) and the middle cerebral artery (MCA).
artery before it terminates into its two terminal branches: the anterior cerebral artery (ACA) and the middle cerebral artery (MCA).
Anterior Cerebral Artery
The ACA has three segments: horizontal (A1), vertical (A2), and distal (A3). The anterior communicating artery connects the bilateral A1 segments. The ACA gives rise to perforating and cortical branches. The perforating arteries include the medial lenticulostriate arteries arising from the A1 segment and the anterior communicating artery, and the recurrent artery of Heubner arising from either the distal A1 or the proximal A2 segment.
The cortical branches include the orbitofrontal (proximal A2), frontopolar (mid A2), pericallosal, and callosomarginal (distal branches of A2).
Normal variants are seen in the ACA including (a) hypoplastic (10%) or aplastic (1% to 2%) A1 segment; (b) bihemispheric ACA, when the distal branches of an ACA supply the contralateral hemisphere; and (c) absent, fenestrated, or duplicated anterior communicating artery. Duplication of the anterior communicating artery is seen in 18%, whereas fenestration is present in 12% to 21% of the population.11
True vascular anomalies include (a) azygos ACA, a single ACA with absent anterior communicating artery, which is seen in 0.5% to 4%, and (b) infraoptic ACA, which is the A1 segment that passes under the optic chiasm instead of over it and is associated with aneurysms. Occlusion of an azygos ACA due to thromboembolic disease may result in ischemia/infarction of both hemispheres.
Middle Cerebral Artery
The MCA has four segments: horizontal (M1), insular (M2), opercular (M3), and cortical (M4).
The MCA gives rise to perforating branches and cortical branches. The perforating branches include the lateral lenticulostriate arteries and the anterior temporal artery, all arising from the M1.
The cortical branches arise from M4, including orbitofrontal artery, prefrontal artery, precentral (prerolandic) artery, central sulcus (rolandic) artery, postcentral (anterior parietal) artery, posterior parietal artery, angular artery, temporo-occipital artery, posterior temporal artery, and medial temporal artery.
There is high variability in the branching pattern of the MCA resulting in early bifurcation or early trifurcation of the artery. True vascular anomalies are rare and mainly include (a) hypoplastic or aplastic MCA; (b) duplicated MCA, arising from the distal ICA before its termination; (c) accessory MCA; and (d) fenestrated MCA. An accessory MCA arises from the ACA and runs parallel to M1. It is seen in less than 3% of cases and supplies the anteroinferior of the frontal lobe.10
Posterior Circulation
Posterior Cerebral Arteries
The two posterior cerebral arteries (PCAs) are the terminal branches of the basilar artery.
The PCA has four segments: precommunicating (P1), ambient (P2), quadrigeminal (P3), and calcarine (P4).
The PCA gives rise to perforating, choroidal, and cortical branches. The perforating branches include the posterior thalamoperforating arteries from P1, the thalamogeniculate arteries from P2, and the peduncular perforating branches from P2 as well.
The choroidal branches include the medial posterior choroidal and the lateral posterior choroidal arteries.
The cortical branches include the anterior and posterior temporal arteries arising from P2. Then, the distal PCA terminates into two trunks: (a) a medial trunk giving rise to the medial occipital artery, parietoocipital artery, calcarine artery, and posterior splenial arteries and (b) a lateral trunk giving rise to the lateral occipital artery and temporal arteries.
A normal variant of the PCA is a fetal origin of the PCA, where the PCA arises directly from the internal carotid artery as a large posterior communicating artery with hypoplastic or aplastic P1 segment. This variant is common and present in 10% of the population.12
A true vascular anomaly is persistent carotid-basilar anastomoses, where the PCA originates from persistent trigeminal artery or a proatlantal intersegmental artery.
Vertebrobasilar Arteries
The V4 segment is the intracranial segment of the vertebral artery starting after it enters the cranium through the foramen magnum, and both V4 segments unite to form the basilar artery. The V4 segment gives rise to the anterior and posterior spinal arteries, and the perforating branches give rise to the medulla and the posterior inferior cerebellar artery.
The basilar artery gives rise to the perforating pontine and midbrain branches, the anterior inferior cerebellar artery, and the superior cerebellar artery (SCA), and terminates in the PCA.
There are many variations in the dominance of the vertebral arteries and the branching pattern of the basilar artery. True vascular anomalies include fenestrated or duplicated basilar artery and persistent carotid-basilar anastomoses.
Arterial Vascular Territories
There is overlap between the vascular territories of the anterior, middle, and posterior cerebral arteries. Typically, vascular territories are as follows (Fig. 1.2):
Anterior Cerebral Artery
The perforating branches supply the caudate head, the anteromedial lenticular nucleus, the genu of the corpus callosum, and the anterior limb of the internal capsule.
The cortical branches supply the anterior two-thirds of the medial hemispheres, the inferomedial frontal lobes, and the medial 1 to 2 cm of the cerebral convexity.
Middle Cerebral Artery
The perforating branches supply most of the lenticular nucleus, most of the caudate nucleus, the superior internal capsule, and deep white matter.
The cortical branches supply the temporal poles and most of the lateral surface of the cerebral hemispheres.
Posterior Cerebral Artery
The perforating branches supply the thalamus, hypothalamus, midbrain, and choroid plexus.
The cortical branches supply most of the inferior temporal lobes, the occipital pole, and the posterolateral surface of the cerebral hemispheres.
Basilar Artery
It supplies the pons, superior vermis, and superior cerebellum and all the vascular territory of the PCA.
Vertebral Arteries
Supply the cerebellar tonsils, most of the medulla, and the inferior cerebellum and vermis.
Anterior Choroidal Artery
Supplies the posterior limb of the internal capsule and part of the hippocampus.
Arterial Watershed Areas
These are the border zones between arterial territories that become vulnerable to ischemia with hypoperfusion. There are two watershed zones:
Cortical watershed zone, between the major cerebral arteries ACA, MCA, and PCA
Deep white matter watershed zone, between the perforating branches and the cortical branches of the circle of Willis
Arterial Anastomosis
The circle of Willis forms the main anastomosis of the anterior and posterior circulation and is formed by 10 components: Two ICAs, two A1 segments, an anterior communicating artery, two posterior communicating arteries, two P1 segments, and a basilar artery.
The circle of Willis is more often found to be incomplete rather than complete. Absence of the anterior communicating artery is found in 5% of surgeries.13
Anastomosis also exists between the branches of the internal carotid and external carotid arteries.
In patients with intracranial arterial occlusion, sufficient blood supply may still be available via anastomotic collateral pathways. Demonstration of the extent of leptomeningeal collateral channels beyond the occlusion has important prognostic significance for patient outcome.14 Collateral blood supply can be shown by digital subtraction angiography, MR angiography, or CTA. The effectiveness of collateral blood supply can be assessed by MR or CT perfusion techniques.15,16 Overall poor collateral status is described as less than 50% collaterals and good collateral status as =50%.16
Brain Vein
The detailed anatomy of the brain venous system is beyond the scope of this chapter. It is grossly comprised of two groups that are interconnected:
Dural venous sinuses
Cerebral veins: superficial, deep, and brainstem and posterior fossa veins
The Dural Venous Sinuses
Dural venous sinuses are divided into the following:
Anteroinferior group including the cavernous sinus, the superior and inferior petrosal sinuses, the clival sinus, and the sphenoparietal sinuses.
Posterosuperior group including the superior sagittal sinus, the inferior sagittal sinus, the straight sinus, the transverse sinuses, the sigmoid sinuses, and the occipital sinus (Fig. 1.3).
Another way of classifying the dural venous sinuses is as paired and unpaired sinuses. Confluence of the sinuses or torcular herophili is usually defined as the confluence of the superior sagittal sinus, transverse sinus, and straight sinus (Fig. 1.3B). Confluence of all three sinuses is seen in less than 30% of patients. Common variants include branching of the straight sinus into the bilateral transverse sinuses and preferential drainage of the superior sagittal sinus draining into the right transverse sinus17 (Fig. 1.3B).
Cerebral Veins
Superficial Veins
Superficial veins are located in the leptomeninges and drain the cerebral cortex and subcortical white matter. They are formed by three groups of veins: (a) superior group that includes the small cortical veins and vein of Trolard, (b) middle group that includes the superficial middle cerebral vein and the vein of Labbé, and (c) inferior group that includes the deep middle cerebral vein and the basal vein of Rosenthal.
Deep Veins
These include the subependymal veins, the internal cerebral veins, and the vein of Galen and drain the brain tissue around the ventricles or the basal cistern and connect with the cortical veins.
Brainstem and Posterior Fossa Veins
These are classified into superior, anterior, and posterior groups.
Venous Vascular Territories
The venous vascular territories are highly variable and not well known as the arterial vascular territories (Fig. 1.4).
In general, the basal ganglia and the deep white matter are drained by the internal cerebral veins.
While most of the superior and middle brain surfaces are drained by superficial cortical veins into the superior sagittal sinus, the temporoparietal lobes are drained by the vein of Labbé and transverse sinuses, and the insular cortex and the parenchyma around the sylvian fissure are drained by the sphenoparietal and cavernous sinuses.
▪ Brain Microcirculation
The brain is a highly perfused organ, and in order to function normally, it consumes 20% of available oxygen delivered by carotid and vertebral arteries. The cerebral microcirculation comprises a network of terminal arterioles, capillaries, and postcapillary venules.19 Its arterial supply is derived from recurrent branches of several cortical arteries penetrating into the Virchow-Robin spaces. Capillary
density is about threefold higher in the cerebral gray matter than in the white matter. In contrast to other organs, both large arteries and small arterioles (<100 µm diameter) contribute significantly to vascular resistance. This is a protective mechanism that prevents vasogenic edema if the blood pressure is suddenly raised.
density is about threefold higher in the cerebral gray matter than in the white matter. In contrast to other organs, both large arteries and small arterioles (<100 µm diameter) contribute significantly to vascular resistance. This is a protective mechanism that prevents vasogenic edema if the blood pressure is suddenly raised.
Distinguishing characteristics of the brain capillary circulation in the cortex compared to other organs are the existence of tortuous course of capillaries and a remarkably high velocity of red blood cells.19,20,21 and 22 Other characteristics of the brain perfusion are continuous blood flow in the capillaries and that at rest greater than 90% of cortical capillaries are functional.19 In spite of continuity of flow, transient regional variation in mean transit time (MTT) within the capillary network exists.21 This variation in MTT is mainly due to differences in capillary path length and flow velocity and may change in response to various physiologic stimuli such as changes in arterial PCO2 and perfusion pressure. For example, functional neuronal activation increases cerebral blood flow (CBF) and decreases the heterogeneity of capillary perfusion. The path length and velocity for red blood cells are important determinants of capillary gas and nutrient exchange.19 In the brain, the tortuous course of capillaries tends to increase MTT, whereas the high velocity of flow has the opposite effect. The average capillary velocity is about 0.5 to 1.8 mm/s. At rest, red cells travel through the capillary network in 100 to 300 milliseconds along 150- to 500-µm-long paths.21 MTT values of normal gray and white matter are not significantly different (5 to 6 seconds), but CBF of gray matter is higher than that of white matter (70 mL/100 g/min vs. 20 mL/100 g/min).23
Cerebral circulation time is an important parameter that provides information on cerebrovascular physiology and helps to define the perfusion status of the brain. It can be measured using CT perfusion techniques by measuring the times of contrast bolus arrival in the internal carotid artery and jugular bulb. The average value is 5.8 ± 1.4 seconds. The timing will be increased in arterial occlusion and is faster in arteriovenous malformations.24
The response of capillary flow to decreased perfusion pressure reflects autoregulation of CBF (Fig. 1.5). Autoregulation is well developed in the brain to maintain a constant CBF despite changes in cerebral perfusion pressure (CPP).22 The CPP is the difference between arterial and venous pressure. The brain venous pressure is proportional to intracranial pressure. According to Ohm law, CBF equals CPP divided by the vascular resistance, and according to Poiseuielle law, CBF is inversely related to vessel radius to the fourth power. Therefore, adjustment of vessels’ diameter by autoregulatory mechanisms is the primary function to maintain vascular resistance hence CBF. In normotensive adults, CBF is maintained at approximately 50 mL/100 g of brain tissue per minute, provided CPP is in the range of approximately 60 to 160 mm Hg.25 Above and below this limit, autoregulation is lost, and CBF becomes dependent on mean arterial pressure in a linear fashion26 (Fig. 1.5). Cerebrovascular reserve (CVR) provides important information on hemodynamic status in patients with chronic cerebrovascular occlusive diseases.27 CVR can be measured by measurement of CBF before and after acetazolamide administration. Acetazolamide is a carbonic anhydrase inhibitor that dilates vessels by increasing blood CO2 levels. In patients with stenotic vessels and decreased CPP, the vessels are already maximally vasodilated by autoregulatory mechanisms and cannot respond further to acetazolamide. These patients are considered to be at increased risk of stroke and can be diagnosed with acetazolamidechallenged test.
Acute hypoxia causes vasodilation and increased CBF by direct effect on the arterial wall muscles, and increased local production of the nitric oxide and adenosine.19 CBF does not increase until parenchymal PO2 falls below 50 mm Hg.21 Vasodilatory response of cerebral microcirculation to hypercapnia is also responsible for increase in capillary flow velocity and CBF, but the mechanism appears to be mainly related to a direct effect of extracellular H+ on vascular smooth muscle.28 It is seen that with hypercapnia, CBF increases by 54% and CBV increases by 41%.29 CBV is the sum of arterial, capillary, and venous blood volumes with an arterial fraction of 30% in humans.19 Changes in CBV during changes in PCO2 are caused by changes in arterial blood volume without changes in venous blood volume.30 Autoregulatory impairment in acute hypertension (e.g., eclampsia) occurs. Increased intravascular pressure in acute hypertension that is above the autoregulatory limit causes forced dilatation of cerebral vessels, decreased vascular resistance, increased CBF, and increased hydrostatic pressure resulting in brain edema.31 Sustained hypertension above CBF autoregulation as seen in acute hypertensive encephalopathy causes cerebral vasospasm, ischemia, and edema.32
In acute tissue hypoxia such as within infarction core, cerebral autoregulation is severely disturbed in the early stages. Severe hypoxia leads to tissue lactate acidosis and local vascular paralysis, compromising the autoregulatory mechanism in both the ischemic core and periinfarct region.33 Autoregulatory impairment tends to increase mainly in large infarction and involves the contralateral side during the first days after ischemic stroke.34 A vicious cycle of reperfusion, production of inflammatory vasotoxic substances, impaired autoregulation, edema, and progression of ischemia has been described.34 In acute brain infarction, both of the infarcted and ischemic (penumbra) zones show decreased CBF and increased MTT (Fig. 1.6). However, in the infarcted tissue, the cerebral blood volume (CBV) is markedly
decreased, whereas in the penumbra, due to preserved autoregulatory mechanisms (vasodilatation and recruitment of collaterals), the CBV remains normal to slightly increased.23 In ischemic gray matter, CBF values are close to normal white matter CBF values. Wintermark et al.35 reported an MTT of greater than 145% as the threshold for the ischemic tissue at risk of infarction and absolute CBV of 2.0 mL/100 g as the most accurate parameter of the acute infarct core delineation and corresponds the restricted diffusion area in diffusion-weighted MR, indicating irreversible damage.35
decreased, whereas in the penumbra, due to preserved autoregulatory mechanisms (vasodilatation and recruitment of collaterals), the CBV remains normal to slightly increased.23 In ischemic gray matter, CBF values are close to normal white matter CBF values. Wintermark et al.35 reported an MTT of greater than 145% as the threshold for the ischemic tissue at risk of infarction and absolute CBV of 2.0 mL/100 g as the most accurate parameter of the acute infarct core delineation and corresponds the restricted diffusion area in diffusion-weighted MR, indicating irreversible damage.35
▪ Pulmonary Circulation
The flow of blood from the heart into the lungs and back to the heart is accomplished by two separate pathways, the pulmonary circulation and bronchial circulation. Pulmonary circulation represents the bulk of blood flow into the lungs for the primary goal of blood oxygenation, whereas the bronchial circulation nourishes the airways and lungs.
Pulmonary circulation arises directly from the right ventricle in a distinct, isolated circuit that runs in parallel to the systemic arterial circuit, from which bronchial blood supply originates. While the pulmonary vascular circuit runs in parallel to the systemic arterial circuit, there is an important difference, much lower pressure within the pulmonary circuit. Despite this low pressure, it is a highflow circuit, receiving the entire volume of cardiac output with each stroke (5 to 6 L/min). The pulmonary circuit exists for the primary purpose of gas exchange and blood oxygenation.
The low overall pressure of the pulmonary circuit is the effect of low overall resistance from the pulmonary microcirculation. Pulmonary microcirculation is a smaller and shorter vascular network that is simultaneously more dense, offering a much larger surface area.
Pulmonary macrocirculation and microcirculatory arteries, arterioles, and capillaries are connected in series with one another, and their pathologic classification depends on their wall composition such as the degree of muscularity or presence of elastic lamina.36
▪ Embryology
Pulmonary circulation arises in tandem to the development of the human cardiovascular system. By day 34, in embryogenesis, circulation exists between the primitive heart and the lung buds.37
There is a secondary system, which is present early in life, that exists to offer collateral circulation between the pulmonary and systemic circuits. This system seemingly recedes but remains a potential system for collateral flow between the higher pressure bronchial/systemic circuit and the much lower pressure pulmonary circuit if there is breakdown or disruption in either parent system.37,38 and 39
▪ Pulmonary Artery Macrocirculation
Pulmonary arterial circulation passes deoxygenated blood from the right heart into the pulmonary capillary network throughout both lungs by way of a series of branching pulmonary arteries, which progressively decrease in caliber.
Normal Anatomy
From the right ventricular outflow tract of the heart arises the main pulmonary artery (MPA), a short conduit that arches from the anteriorly situated right ventricle to divide into the right pulmonary artery (RPA) and the left pulmonary artery (LPA).
The RPA has a longer course, having to cross the midline, coursing posterior to both the ascending aorta and the superior vena cava. The RPA simultaneously passes cranial to both the right atrium and the right superior pulmonary vein. At the level of the right hilum, the anterior-to-posterior relationship of vessels is vein, artery, and bronchus. RPA divides into three major trunks, the right upper, middle, and lower lobe arteries. The right upper artery subdivides into an apical, posterior, and anterior segmental artery. The right middle artery subdivides into medial and lateral segmental branches. Finally, the right lower artery subdivides into apical, anterior, posterior, medial, and lateral branches.37
The LPA is shorter and is a continuation of the curvature of the MPA. The LPA arches cranially over the left mainstem bronchus to ultimately hook over the left superior bronchus at the level of the pulmonary hilum. At the level of the left hilum, the anterior-toposterior relationship of vessels is vein, bronchus, and artery. LPA divides into two major trunks, the upper and lower lobe pulmonary arteries. The upper lobe pulmonary artery subdivides into the apicoposterior, anterior, and lingular arteries, with superior and inferior branches of the lingular artery. The left lower lobe pulmonary artery subdivides into the superior as well as anteromedial, lateral, and posterior basal segmental arteries.39,40
Variant Anatomy
While the aforementioned pulmonary arterial relationships are the most commonly encountered, there is variability to this branching pattern encountered in 16.4% of patients.41 The most common variants involve right middle lobe and lingular arteries, anomalously arising from the basal lower lobe arteries.
▪ Pulmonary Artery Microcirculation
Pulmonary microcirculation consists of small arterioles and capillaries (Fig. 1.7). This microcirculation network comprises approximately 25% of lung volume42 and is designed to handle the entirety of cardiac stroke volume, permitting proper gas exchange.
Small pulmonary arterioles contain smooth muscle fibers and thus have a level of control over blood distribution, acting as resistance vessels and allow for the shunting of blood within the microcirculation. Even smaller capillaries arise from the branching arterioles, which then form a network of vessels surrounding the alveoli. These capillaries have very thin walls, less than 0.1 µm thick, permitting gas exchange and oxygenation of blood. In addition to gas exchange, the capillaries play a vital role in chemical substance exchange, such as water and electrolytes, as well as biochemical destruction and synthesis. For example, the vasoconstrictive hormone angiotensin I is converted to the active angiotensin II form at the pulmonary capillary level. After gas exchange occurs, blood then continues within the pulmonary venous system for ultimate return to the heart.
▪ Bronchial Artery Circulation
Bronchial circulation differs from pulmonary circulation in that it carries oxygenated blood on the arterial side, arising from the aorta. Bronchial circulation supplies the trachea and bronchia, bronchovascular bundles, pulmonary interstitium, lymph nodes, visceral pleura and the vasa vasorum of the aorta, pulmonary artery, and pulmonary vein.43
Normal Anatomy
Bronchial arteries arise directly from the aorta to supply both the right and left lung. There are four typical variations of bronchial artery anatomy, the most common variation (41% of people)43,44 has one bronchial artery on the right and two on the left. In this variation, the bronchial arteries arise from the T5-6 level. The right bronchial artery arises from a crossing right intercostal artery. The left bronchial supply is, however, the result of two bronchial arteries (superior and inferior bronchial artery), arising directly from the aorta. The left superior bronchial artery directly branches from the anteromedial aorta, lateral to the carina and posterior to the left main bronchus. The left inferior bronchial artery has similar origin but inferior to the left main bronchus.
Variant Anatomy
There is a wide ranging distribution of variant bronchial anatomy; however, all variants ultimately arise from the aorta carrying oxygenated blood. Reported variants include right bronchial artery
arising from the left superior bronchial artery, bronchial arteries arising from internal mammary arteries, and the thyrocervical trunk among some examples.
arising from the left superior bronchial artery, bronchial arteries arising from internal mammary arteries, and the thyrocervical trunk among some examples.
▪ Bronchopulmonary Arterial Collateral Flow
Several studies have demonstrated the presence of collateral circulatory pathways between the pulmonary arterial system and bronchial arterial system early in life, but in the normal adult lung, this system recedes. While likely still present, there is no demonstrable flow within the collateral vessels.
However, anastomotic flow has been observed in cases of diseased lung, such as in cases of fibrotic interstitial lung disease,42 atypical mycobacterial infection, or lung cancer. The result is much higher pressure systemic blood flow (bronchial) entering the lower pressure pulmonary circuit. The result of which is vascular breakdown and ultimate hemoptysis. In cases of pulmonary embolism, flow through these channels can compensate for lack of pulmonary flow and explains why pulmonary infarction is seen in only a minority of cases.
▪ Cardiac Vessels
Knowledge of the anatomy and physiology of the coronary circulation including macro- and microcirculation is essential for understanding the mechanisms that lead to its dysfunction. Noninvasive techniques for evaluation of coronary artery anatomy include transthoracic echocardiography, transesophageal echocardiography, coronary magnetic resonance angiography (MRA), and coronary computed tomography angiography (CTA). Coronary MRA and CTA have emerged in recent years as recommended noninvasive imaging of epicardial coronary arteries. Although CTA is the best technique for demonstration of medium-sized epicardial coronary vessels, none of the imaging tests listed above has adequate spatial and temporal resolutions to directly demonstrate microvessels in clinical practice.
▪ Coronary Arteries
Recent histologic analysis in mouse and cardiac organ culture have shown that coronary vessels arise from the angiogenic sprouts of the sinus venosus, the major vein that returns circulating blood to the embryonic heart.45 Some sprouting venous endothelial cells dedifferentiate into arteries and capillaries as they invade the myocardium and some remain on the surface and differentiate into veins.45 Coronary arteries are variable in anatomic origin, course, and branching. In the majority of hearts, two coronary arteries exist, the right coronary artery (RCA) and the left main coronary artery bifurcating into the left anterior descending (LAD) and the left circumflex (LCx) arteries (Figs. 1.8 and 1.9). In 10% to 15%, the left main coronary artery trifurcates into the LAD, LCx, and ramus intermedius arteries. The main coronary vessels are considered arteries, and the smaller, more distal vessels are generally termed coronary branches. In spite of this generalization, it is not uncommon to call some small branches “artery,” for example, sinoatrial node artery or posterior descending artery (PDA).
The relationship between the coronary artery branches at the crux cordis defines the type of coronary artery dominance and is determined by the artery giving rise to the posterior interventricular branch (PDA)46,47,48 and 49 (Fig. 1.9). The PDA and posterolateral branches that supply the inferior wall can originate from the RCA only (right dominant, 65% to 85%), from the left circumflex artery (left dominant), or from both arteries (codominant). In another definition, coronary dominance describes the artery that gives rise to the atrioventricular node artery. In most patients, the PDA arises from the distal portion of the coronary artery, but some patients have early takeoff of the PDA from the mid RCA (split RCA), which then courses toward the apex along the diaphragmatic surface of the right ventricle. Dual
origin of the PDA is not uncommon, one from the mid RCA (marginal branch) and the second from the distal RCA. This variant has been reported in up to 30% of individuals.50 In patients presenting with an acute coronary syndrome, left coronary dominance appears to be independently associated with increased long-term mortality, especially with involvement of the inferior wall of the heart.51 In most individuals with left coronary dominance, the RCA is usually small and an unreliable source of left myocardial perfusion.52
origin of the PDA is not uncommon, one from the mid RCA (marginal branch) and the second from the distal RCA. This variant has been reported in up to 30% of individuals.50 In patients presenting with an acute coronary syndrome, left coronary dominance appears to be independently associated with increased long-term mortality, especially with involvement of the inferior wall of the heart.51 In most individuals with left coronary dominance, the RCA is usually small and an unreliable source of left myocardial perfusion.52
▪ Segmental Blood Supply
The standard 17-myocardial segment model of the left ventricle is suggested by the American Heart Association for all cardiac imaging modalities to facilitate a more detailed analysis of regional left ventricular function and perfusion53 (Fig. 1.10). However, the adopted model shows significant variability and overlap of the segments assigned to a specific territory that makes it difficult to generalize data among different studies (Fig. 1.11).
The vascular supply to the inferior wall of the left ventricle has a spectrum of variations. This part is mainly supplied by the RCA and the LCx. The LAD artery that wraps around the apex can supply some of the apical inferior wall. The lateral wall territory is supplied by the LCx in vast majority of individual but can be shared with a large diagonal from the LAD or the ramus branch. Myocardial blood supply in the inferolateral region corresponds to the LCx or RCA distribution, and the inferoseptal region can be supplied by the LAD or RCA.54 In a correlative MR study, the presence of hyperenhancement in the basal and mid anterior and anteroseptal or apical anterior wall has been 100% specific for LAD artery occlusion.55 The LAD infarcts frequently involve the midanterolateral, apical lateral, and apical inferior walls. No segment is 100% specific for the RCA occlusion. Combination of hyperenhancement in the anterolateral and inferolateral walls is 100% specific for the LCx occlusion, and when extended to the inferior wall, is also 100% specific for a dominant or codominant LCx occlusion. Hybrid imaging combined with the anatomic
information of vascular distribution provided by CTA is useful to study myocardial tissue perfusion abnormalities and the coronary anatomy for more accurate assignment to culprit vessels and to improve monitoring of targeted therapy.56,57 CT studies have shown common overlap between the LAD and the RCA perfusion territories at the inferoseptal region and between the LAD and the LCx territories at the anterolateral region. It is important to realize that the LAD territory can be larger than the AHA-proposed 17-segment model, due to the involvement of the anterolateral and all the apical segments of the left ventricle.54
information of vascular distribution provided by CTA is useful to study myocardial tissue perfusion abnormalities and the coronary anatomy for more accurate assignment to culprit vessels and to improve monitoring of targeted therapy.56,57 CT studies have shown common overlap between the LAD and the RCA perfusion territories at the inferoseptal region and between the LAD and the LCx territories at the anterolateral region. It is important to realize that the LAD territory can be larger than the AHA-proposed 17-segment model, due to the involvement of the anterolateral and all the apical segments of the left ventricle.54
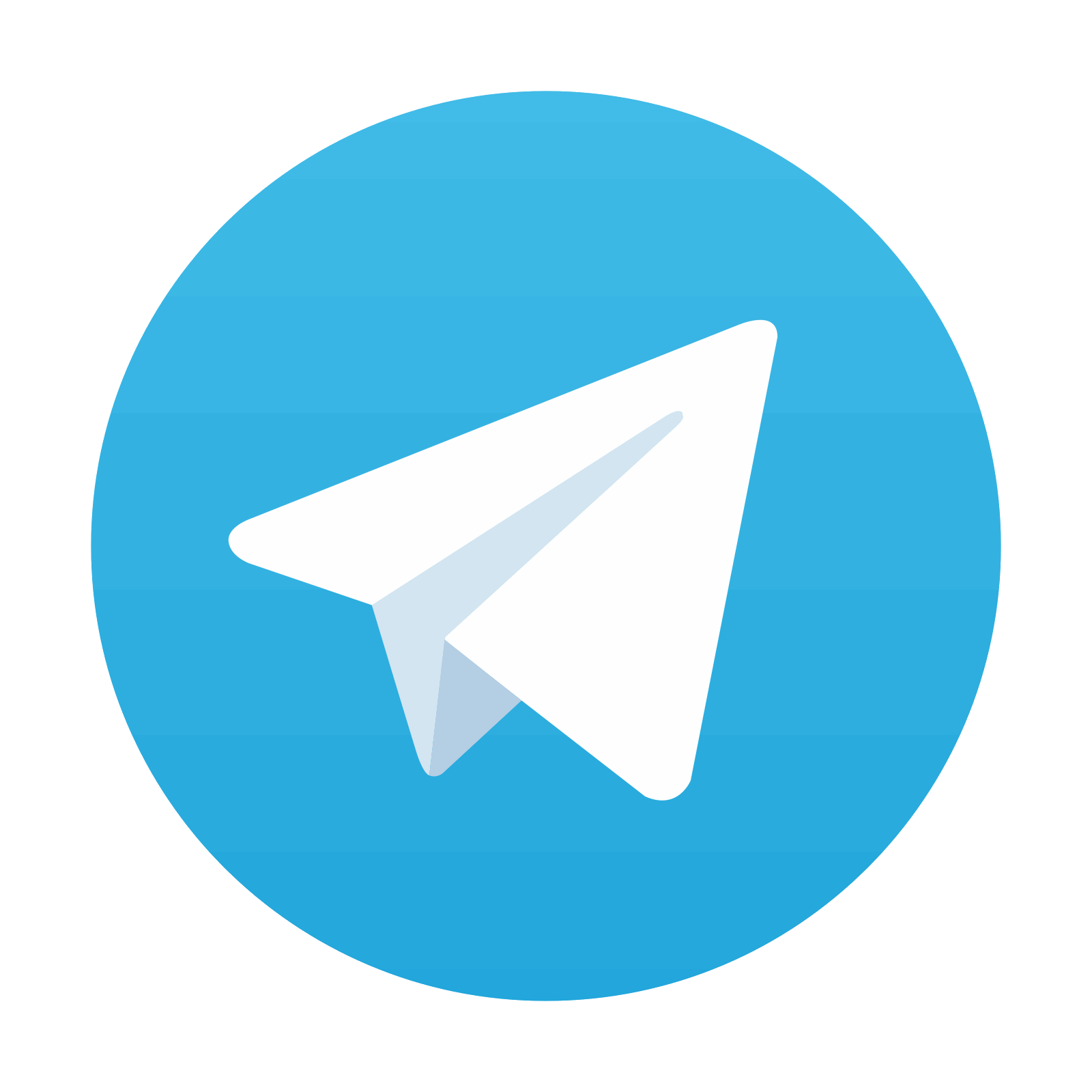
Stay updated, free articles. Join our Telegram channel

Full access? Get Clinical Tree
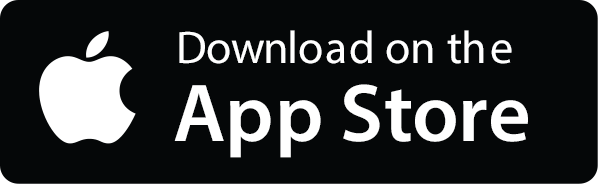
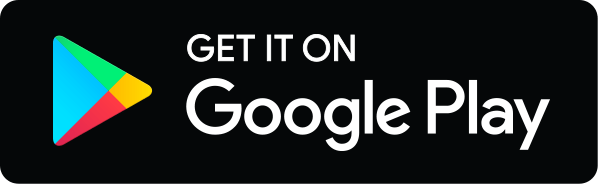