Chapter 13 Vascular Anomalies of the Brain
INTRODUCTION TO ANGIOGRAPHIC MODALITIES
Catheter Angiography
Cerebral angiography was one of the first diagnostic modalities for brain diseases, and it has remained crucially important and routinely used since its description by Egaz Moniz in 1927.1 However, there has been significant evolution from the direct carotid cut downs and cut film technique of the 1920s to digital subtraction angiography with three-dimensional (3D) reconstruction.
With the transition to non-ionic contrast, smaller 4- and 5-French, and more flexible catheters and guidewires with hydrophilic coating, the risk for permanent neurologic injury was reduced from 1% to 2.4%2,3 to less than 0.1% to 0.3%.4–6 However, given risk of local pain, bruising, and hematoma in up to 25% of cases, noninvasive alternatives should be considered whenever they will provide similar diagnostic information.
Cerebral angiography remains the gold standard in the diagnosis of dural arteriovenous fistulas (dAVFs), as well as small aneurysms and arteriovenous malformations (AVMs). In addition, super-selective angiography can provide functional and physiological data important for clinical decision analysis, although pressure measurements are not commonly performed. It is still strongly recommended that a magnetic resonance imaging (MRI) study and a four-vessel angiogram be obtained to delineate the anatomy of an AVM.7 Computed tomography (CT) angiography can outline enlarged abnormal cortical veins but may miss subtle dural venous drainage or small arteriovenous malformations.8 MRI may show important indirect signs such as edema and abnormal cortical enhancement. In our practice, all patients with unexplained subarachnoid, subdural, or atypical parenchymal hemorrhage undergo digital subtraction angiography (DSA).
Three-Dimensional Digital Subtraction Angiography
Excellent spatial resolution and lack of artifact has made 3D DSA an attractive tool for treatment planning and follow-up (Figure 13-1). It further increases the sensitivity for detection of aneurysms smaller than 3 mm9 and provides invaluable conformational information for endovascular treatment planning (Figure 13-2).
Computed Tomography Angiography
Multidetector CT angiography (MDCTA) has higher spatial resolution and decreased, but not eliminated, propensity to motion artifacts (Figure 13-33) than MRA.10,11 For these reasons, MDCTA has largely replaced MR angiography in our practice.
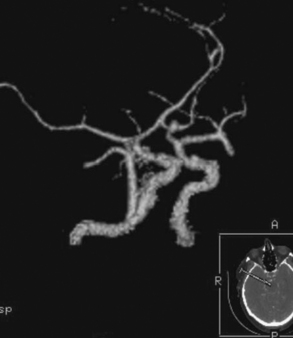
Figure 13-33 Computed tomography (CT) angiography with motion artifact. The CT angiogram suggested moderate perianeurysmal vasospasm, which was not confirmed by digital substraction angiography (see Figure 13-34 A and B,).
The sensitivity for detection of even small aneurysms has substantially increased. One study found MDCTA to be actually more sensitive than DSA for the detection of small aneurysms.12 Yet DSA remains the gold standard for aneurysm detection. Colen et al.13 found that during screening of patients with nontraumatic subarachnoid hemorrhage, MDCTA missed the primary aneurysm in 11 of 211 cases. Regions with overprojecting bone (such as the cavernous segment or supraclinoid carotid artery) may not be optimally visualized,14,15 and MDCTA has lower sensitivity in detecting branches incorporated into the aneurysmal sac.16
The bony structures of the sella are often manually excluded on the reconstruction and thus an aneurysm can easily be “removed” in the cavernous and supraclinoid region (as seen in Figure 13-31, F). Careful analysis of the source images is crucial to preserve high sensitivity. Inadequate bolus timing with poor arterial and dense venous filling can render interpretation of CT angiography much more challenging. Still, CT angiography can be superior to DSA for aneurysms with competing flow, such as some basilar tip, basilar trunk, or anterior communicating aneurysms because it avoids the problem of contrast “washout” (see Figure 13-18).
CT angiography is valuable for follow-up of aneurysms treated with titanium alloy clips, but it is not useful when cobalt alloy clips have been used.17 Image quality is also degraded by orientation of the clip perpendicular to the imaging axis or presence of multiple clips. Streak artifacts caused by platinum coils make it an unsuitable method to assess for recanalization of embolized aneurysms. (See Figures 13-3 and 13-4.)
In our institution, patients with subarachnoid or atypical intracranial hemorrhage are generally screened with CT angiography. An aneurysmal source of bleeding can often be identified in the emergency room, allowing triage to endovascular versus surgical treatment.
If the CT angiography is nondiagnostic, DSA is always performed to evaluate for a missed aneurysm or nonaneurysmal sources, such as a dural arteriovenous (AV) fistula. See Figure 13-5.
However, protocols relying directly on DSA are also very acceptable and may actually reduce cost.
Magnetic Resonance Angiography
TOF techniques exploit the contrast between the high signal intensity of inflowing, fully magnetized blood and the low signal intensity of saturated stationary tissue. Multiple thin imaging slices (two-dimensional [2D]) or larger slabs (3D) perpendicular to the axis of flow are acquired with a flow-compensated gradient-echo sequence. Three-dimensional TOF angiography allows greater spatial resolution and is more resistant to signal loss from disturbed flow than 2D TOF. However, PC MRA and TOF MRA are sensitive to artifacts caused by turbulent or slow flow (Figure 13-6, B). TOF is also affected by in-plane artifacts.
Contrast-enhanced (TOF) MR angiography has been employed to overcome these problems. It is performed by intravenously injecting a contrast agent to shorten selectively the T1 of the blood to produce the signal, rather than relying on flow. By implementing a T1-weighted imaging sequence during the first pass of the contrast agent, images can be produced showing arteries in striking contrast relative to surrounding stationary tissues and veins. However, synchronizing the acquisition with the arrival of the contrast agent is critical to ensure image quality. For a more detailed discussion on these MR angiography techniques, the reader is referred to the review authored by Aygun.18
Whereas MRA for follow-up of clipped aneurysms is limited by severe “blooming” artifact, it is usually adequate to monitor coiled aneurysms because it has a sensitivity of 75% for detecting a residual neck.19 See Figure 13-7. However, problems related to signal drop-out may occur (Figure 13-8).
No clear information has emerged on whether contrast-enhanced MR angiography is superior to conventional 3D TOF.19,20 Where available, contrast-enhanced 3-Tesla MRA may a superior diagnostic option.21
DSA remains the gold standard for the detection of small AV malformations and dural AV fistulas. Although specialized sequences such as 3T four-dimensional MRA22 and others23 show good correlation for size of feeders and AVM classification, DSA is still more reliable in the diagnosis of small AVMs, which can be missed even by repeated MRA studies.24 Contrast-enhanced MRA can be used to follow dural AV fistulas, but it is less sensitive than DSA (Figure 13-9).25
COMPUTED TOMOGRAPHY AND MAGNETIC RESONANCE IMAGING
CT without contrast is poorly sensitive for brain aneurysms. However, unruptured aneurysms may present with rim calcification or slight hyperdensity due to the blood contained in the aneurysm. Only relatively large (≥10mm) aneurysms can be seen on plain CT scan, however (Figure 13-10).
CT without contrast is the standard screening test when subarachnoid hemorrhage is suspected (see Chapter 12), but almost all aneurysm locations in the circle of Willis can produce diffuse and symmetric subarachnoid hemorrhage and a dedicated vascular study is necessary to define the source of bleeding. Sometimes an aneurysm can be seen as a hypodense region within dense subarachnoid hemorrhage, a finding often referred to as a “ghost sign.” The distribution of the blood can be helpful to predict the location of the ruptured aneurysm, especially when multiple aneurysms are present at the time of hemorrhage. The location of parenchymal hemorrhage is typically adjacent to the point of rupture (Figure 13-11).
Unenhanced CT of the brain has poor sensitivity for vascular malformations but sometimes outlines enlarged draining veins of AVMs (Figure 13-12, A). Administration of contrast significantly increases the sensitivity of CT scans for the diagnosis of vascular abnormalities.
MRI
MRI of the brain, although more sensitive than CT, is not adequate to rule out the presence of an aneurysm. However, given the frequent use of this technique as screening test for many neurologic conditions, most of the incidental aneurysms treated in our institution are identified by this technique. Indirect markers are additional flow voids adjacent to vessels or, less commonly, perianeurysmal gliosis (see Figure 13-39 B,).
Rarely, slow flow within the aneurysm can cause high signal of fluid-attenuated inversion recovery (FLAIR)/T2, which can be confused with focal thrombus or hemorrhage (Figure 13-13).
MRI is indispensable in the diagnosis and management planning of AVMs and is part of the routine diagnostic workup preceding the treatment of these lesions (see Figures 13-12 B–C, and 13-43 A–C,).
ANEURYSMS
General Concepts
Types of Aneurysms
Nonsaccular Aneurysms
Fusiform Aneurysms
Transitional Forms
Dolichoectasia
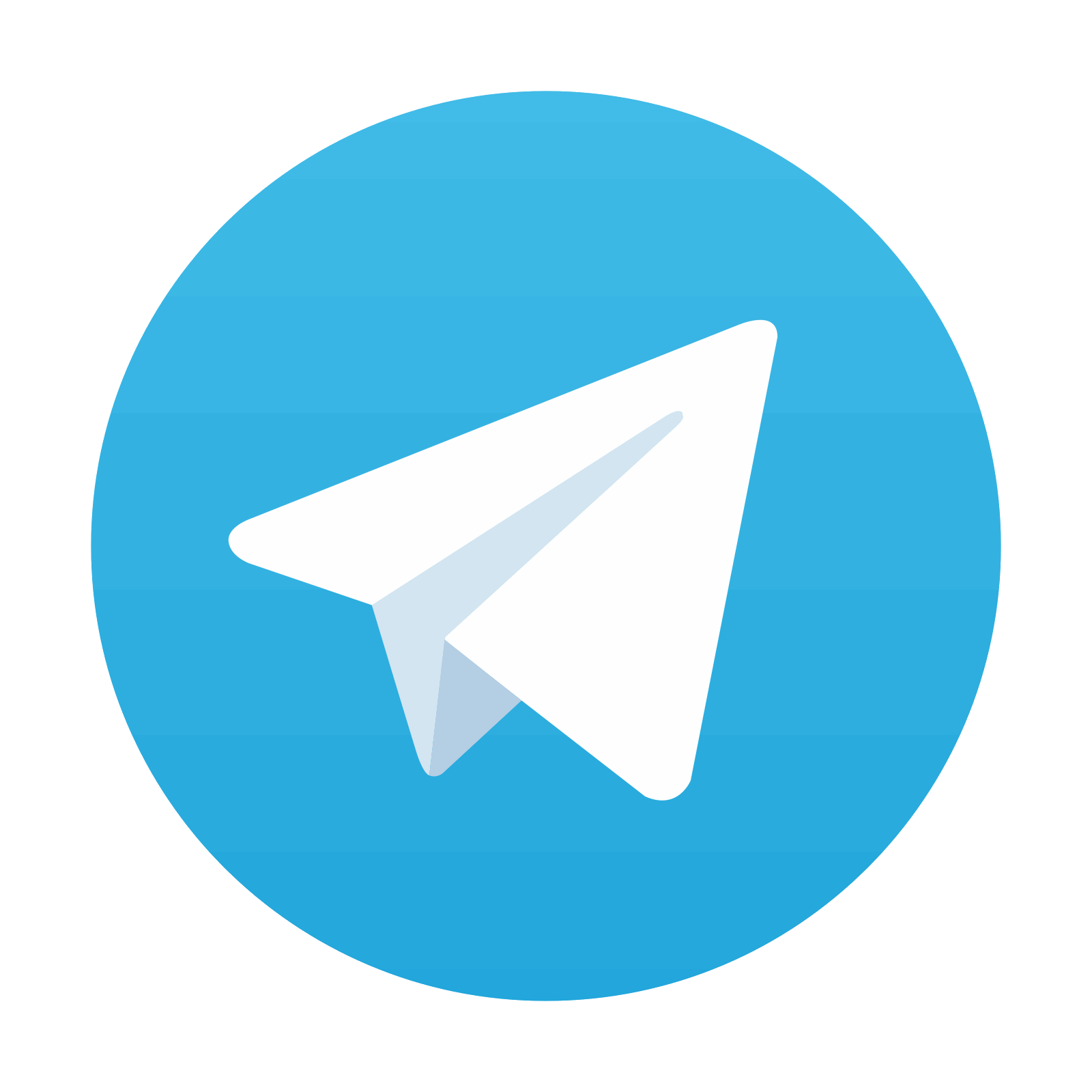
Stay updated, free articles. Join our Telegram channel

Full access? Get Clinical Tree
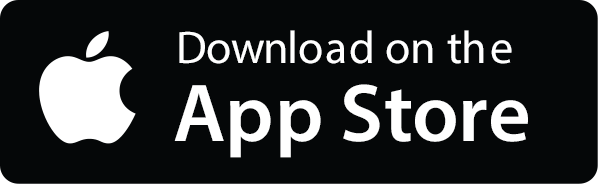
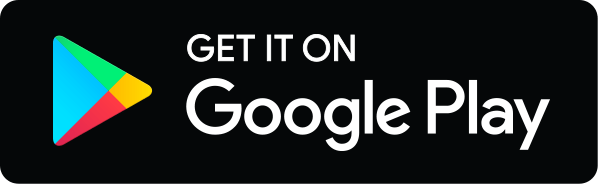