Fig. 18.1
T2-weighted MR images of the patient showing an infarct in the left temporal area (a: 1st stroke), with another infarct in the right frontal area (b: 2nd stroke), and a third in the right parietal area (c). Miller and Boeve (2009)
This patient meets the NINDS-AIREN criteria for probable VaD, since there were impairments of both memory and of two or more cognitive domains, evidence of cerebrovascular disease by focal signs on neurologic examinations and brain imaging, and a temporal relationship between the dementia and the strokes. After the first attack, he exhibited mild language impairment, which gradually improved to the extent that he was able to manage daily activities. Following the patient’s second stroke, his abnormal behavior and impaired judgment rendered him functionally impaired and withdrawn from complex activities, which again slightly improved over time. After the third stroke, his condition declined significantly, and he was not able to perform basic activities such as dressing and eating. Over the course of approximately 2 years, this case exemplifies the clinical features of stepwise deterioration and a fluctuating course of acute deficits followed by partial recovery. These were caused by three territorial infarctions without subcortical ischemic changes or lacunes, typical of MID.
The total lesion volume size, the number of lesions, and the localization of such lesions are crucial factors for causing MID (in general, the larger infarct size, the more severe the dementia) (De Reuck et al. 1981; Erkinjuntti 1987). However, a quantitative correlation between the degree of cognitive deficits and either lesion volumes or lesion locations has not been clearly elucidated (Erkinjuntti et al. 1999). The NINDS-AIREN criteria provide only a limited suggestion that bilateral anterior cerebral artery distribution, posterior cerebral artery distribution, parietotemporal and temporo-occipital association areas, and superior frontal and parietal watershed territories can be considered major candidate areas for VaD (Román et al. 1993). Multiple infarctions in any combination of these restricted cortical deficits will result in MID.
18.2.2 PET and SPECT in MID
In the 1980s and 1990s, when the term MID was applied to cover all subtypes of VaD, advances in PET and SPECT technology were introduced as tools to distinguish between Alzheimer’s disease (AD) and MID. While hypoperfusion or glucose hypometabolism in the temporoparietal area was consistently seen in AD cases, in MID, variable patterns of perfusion or metabolic defect were found to be dependent on the sites of cerebral infarction. One 18F-2-fluoro-2-deoxyglucose (FDG)-PET study reported that patients with MID exhibited global reductions in glucose metabolic rates with focal and asymmetric hypometabolism compared to normal controls. However, patients with AD showed hypometabolism in all areas with relative sparing of the primary motor and sensory cortex (Benson et al. 1983). Gemmell et al. compared the pattern of hypoperfusion seen in patients with AD to that of patients with MID using SPECT with 123I-isopropylamphetamine (IMP). They observed a reduction in IMP uptake in parieto-occipital regions in AD patients but in multiple cortical areas in MID patients (Gemmell et al. 1984). Since then, further studies have supported the utility of IMP SPECT in helping with the differential diagnosis of AD from MID and vice versa (Cohen et al. 1986). In a 133Xe inhalation SPECT study, the reduction of cerebral blood flow (CBF) in the temporoparietal area was identified in AD, but patchy whole-brain reduction was seen in MID (Komatani et al. 1988). There have also been several group studies using 99mTc hexamethylpropyleneamine oxime (HMPAO) SPECT to distinguish the different patterns of perfusion seen in AD and MID. One study showed that bilateral temporoparietal perfusion deficits were observed in AD patients, while no typical pattern was detected in MID patients, with images of MID patients ranging from normal to very patchy uptake (Gemmell et al. 1987). In another SPECT study with 99mTc HMPAO involving 17 patients with AD, 10 with MID, and 12 normal controls, tracer uptake in the temporoparietal region was compared. A statistically significant decrease in tracer uptake was found in the case of AD patients compared with normal controls. However, there was no significant difference in tracer uptake between AD and MID, even though AD demonstrated generally reduced uptake compared to that of MID (Weinstein et al. 1991).
Launes et al. introduced criteria for SPECT imaging findings in MID as follows: (1) a single large defect or multiple perfusion defects but no bilateral posterior parietotemporal hypoperfusion and (2) crossed cerebellar diaschisis often present (Launes et al. 1991). Based on these criteria, 99mTc HMPAO SPECT images were assessed by specialists who were blinded to the clinical diagnosis. Twenty-five (75.8 %) out of 33 patients with clinical MID were found to be compatible with MID diagnosis based on SPECT imaging criteria. Subsequently, increased uptake in the anterior parietal region (McKeith et al. 1993) or asymmetric multiple focal perfusion defects (Osimani et al. 1994) in patients with MID were reported. Butler et al. also evaluated SPECT images of clinical MID patients according to their own criteria for MID as follows: (1) single or multiple well-defined, wedge-shaped perfusion deficits and (2) nonsymmetrical distribution related to the main vascular territories. The researchers found that 8 out of 11 patients with clinical MID corresponded to their SPECT criteria for MID and regional cerebral blood flow (rCBF) in the temporal lobe significantly correlated with disease severity (Butler et al. 1995).
In the late 1990s, the use of PET scanning emerged for the study of functional activity in patients with MID and multiple infarct without dementia (MS). A study by De Reuck et al. found decreases in both mean rCBF and regional cerebral metabolic rates for oxygen (rCMRO) in all cerebral regions only in patients with MID but similar regional oxygen extraction rates in patients with both MID and MS (De Reuck et al. 1998). The same authors also conducted a PET study to analyze vasoreactivity following intravenous acetazolamide administration. They found moderate loss of vasoreactivity in frontal, temporal, and parietal cortices in MID patients (De Reuck et al. 1999).
The number of MID functional neuroimaging studies has since declined. This may be because structural MRI is now more widely available and functional data for MID from SPECT or PET imaging largely mirrors that obtained from structural MRIs.
18.3 Strategic Infarct Dementia
18.3.1 Introduction
The term strategic infarct dementia (SID) refers to dementia caused by an isolated cerebral ischemic or hemorrhagic lesion. In such cases, the location of the lesion is far more important than the size of the lesion itself. Lesions may be located in specific brain sites that are essential for higher cortical functions, and lesion location can be either cortical or subcortical. Cortical lesions that most often lead to SID occur in the angular gyrus (Benson and Cummings 1982), inferomedial temporal lobe (Ott and Saver 1993), basal forebrain (Alexander and Freedman 1984; Damasio et al. 1985), and retrosplenium (Saito et al. 2003; Valenstein et al. 1987). Most of these cortical lesions involve watershed territories of major intracerebral arteries, including the bilateral basal forebrain (anterior cerebral artery (ACA)/middle cerebral artery (MCA)), angular gyrus (ACA/MCA/posterior cerebral artery (PCA)), and temporo-occipital junction and inferior temporal gyrus (MCA/PCA). Subcortical lesions involving structures such as the thalamus, left internal capsular genu (Chukwudelunzu et al. 2001; Pantoni et al. 2001; Tatemichi et al. 1992), and caudate nuclei (Bhatia and Marsden 1994; Caplan et al. 1990; Mendez et al. 1989) can also cause SID. In cases with SID arising from subcortical lesions, the disruption of the cortical-subcortical connection (indispensible for normal cognitive functioning) has been proposed as the underlying mechanism. Mechanisms for several subcortical infarctions have been investigated by observing functional brain imaging findings.
18.3.2 FDG-PET and SPECT in SID
To date, there have been few studies employing functional neuroimaging to investigate single cortical SID. In contrast, for single subcortical SID, case studies have reported functional neuroimaging findings both in patients with internal capsular infarction and in those with thalamic infarction. The following lists some details of these findings.
1.
Left internal capsular infarction: A decrease in cerebral glucose metabolism in the ipsilateral (left) temporal, occipitotemporal cortices and contralateral cerebellum was reported in one study (Chukwudelunzu et al. 2001). Another study reported the same findings in the left frontotemporoparietal regions, left caudate head, left thalamus, left internal capsule, and right cerebellar hemisphere (Moreaud et al. 1996). A study using SPECT in six patients with capsular genu infarctions revealed reduced perfusion of the inferior and medial frontal cortices (Tatemichi et al. 1992). The interruption of thalamocortical projection through inferior and anterior thalamic peduncles and resulting cortical deactivation were suggested as causative mechanisms (Tatemichi et al. 1992). A typical case of left internal capsular infarction presenting as SID is shown in (Fig. 18.2).
2.
Thalamic infarction: Thalamic lesions involving the anterior, dorsomedial, and various other thalamic infarctions have been reported to cause amnesic and/or behavioral symptoms. Van der Werf et al. noted two types of neuropsychological deficits in thalamic infarction: memory dysfunction (amnesia) and frontal executive dysfunction (Van der Werf et al. 2000). They suggested that amnesic syndrome is dependent on the integrity of the mammillothalamic tract, while various nuclei including the mediodorsal nucleus, midline nuclei, or the intralaminar nuclei may be responsible for frontal dysexecutive-type dysfunction. A study using FDG-PET showed significant hypometabolism in the ipsilateral cortex in patients with unilateral thalamic infarction and neuropsychological deficits (Baron et al. 1986). Disruption of the thalamocortical connection was suggested as a possible mechanism explaining the relationship between cortical hypometabolism and neuropsychological deficit.
Another case report of a patient with small left polar thalamic infarction showed focal hypometabolism in the ipsilateral posterior cingulate cortex and thalamus, but not in the widespread cortical region (Clarke et al. 1994). In that study, the patient exhibited pure amnesia with only slight frontal-type dysfunction. Damage to the mammillothalamic tract of the anterior thalamic nuclei and its projection to the posterior cingulate cortex were proposed as mechanisms to explain the patient’s prominent amnesia and focal hypometabolism in the posterior cingulate cortex. This finding is consistent with the suggestion by Van der Werf et al. that lesions of the mammillothalamic tract are a sufficient and necessary condition for the amnesic syndromes that follow thalamic damage.
These case reports can be explained within the context of the diaschisis, which refers to a decrease in regional cortical metabolism and CBF in an anatomically separate but functionally related area from subcortical lesions. Whether cortical hypometabolism or hypoperfusion associated with diaschisis directly translates into clinical manifestation remains controversial (Baron et al. 1992; Bowler et al. 1995). Further functional neuroimaging studies on this topic involving a large number of patients are needed.
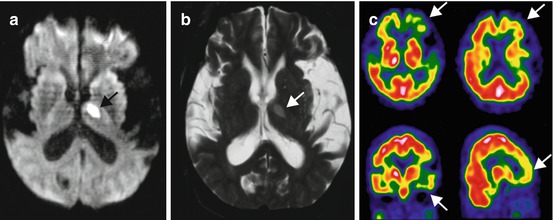
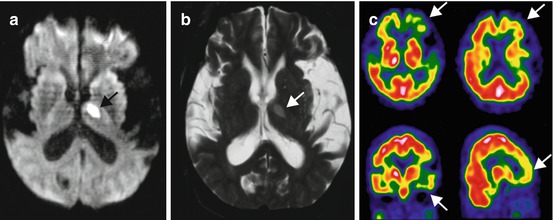
Fig. 18.2
Diffusion-weighted (a) and T2-weighted (b) MR imaging and brain perfusion SPECT using Tc-99m HMPAO (c) in a 56-year-old male patient. He presented with mental confusion of a sudden onset. After the improvement of mental confusion, he exhibited profound memory dysfunction and impairment in activities of daily living. A focal high signal intensity lesion was noted in the left genu of the internal capsule (arrows). Brain SPECT revealed severe hypoperfusion in the left frontotemporal areas (arrows) (Image courtesy of Kyung Won Park (Department of Neurology, College of Medicine, Dong-A University Hospital))
18.4 Subcortical Vascular Dementia
18.4.1 Introduction
Subcortical vascular dementia (SVaD) or subcortical ischemic vascular dementia is associated with small vessel disease that manifests as white matter hyperintensities (WMH), lacunes, and microbleeds located in subcortical areas. Subcortical vascular mild cognitive impairment (svMCI) is a prodromal state of SVaD, and subcortical vascular cognitive impairment (SVCI) refers to cognitive impairment state due to subcortical vascular lesions, which incorporates SVaD and svMCI. SVaD, one of the most common causes of dementia (Dubois and Hebert 2001), displays insidious onset and progresses slowly. It is clinically important in that early detection and intervention can slow down the progression of the disease. However, consensus has not been reached among the medical community in terms of criteria specific for SVaD. Erkinjuntti et al. proposed one criteria for SVaD, which incorporates lacunar state and Binswanger’s disease (Erkinjuntti et al. 2000). Although these criteria were proposed for the specific research objectives, it remains too complicated for practical usage. Thus, we prefer the combination of DSM-IV clinical criteria (American Psychiatric Association 1994) and significant WMH on MRI that meets, for instance, grade III of Fazekas ischemia criteria (Fazekas et al. 1987). For the diagnosis of svMCI, we applied modified Petersen’s criteria (Petersen 2004) and the same image criteria as SVaD (Seo et al. 2010).
SVaD is relatively homogenous and characterized by progressive executive dysfunction, retrieval memory loss, mood changes, and short-stepping gait (Steffens et al. 2003). Cognitive changes in SVaD differ from those in AD dementia, in that frontal executive dysfunction and mild memory deficits of retrieval are the primary clinical manifestation (Roman et al. 2002). This is in contrast to AD dementia, where memory deficits of encoding are the most prominent feature. Mood changes, depression, apathy, emotional bluntness, and psychomotor retardation are the main features of SVaD (Chin et al. 2012; Steffens et al. 2003). The following describes the case of a typical patient with SVaD:
Case
A 76-year-old man with a history of hypertension and diabetes presented with personality change such as apathy and irritability. He also complained of forgetfulness but could recollect when provided with a cue. Two years later, his posture became stooped and the speed of her gait slowed. Upon examination, he showed poor performance in frontal executive functions. His delayed memory was found to be impaired, while recognition memory was relatively preserved. He also exhibited mild lower body parkinsonism. An MRI scan revealed severe WMH and many lacunes. Amyloid retention could not be observed with PiB-PET, suggesting a diagnosis of pure SVaD (Fig. 18.5a).
The underlying mechanisms of frontal-related symptoms in SVaD are not fully understood. The prevailing model involves interrupted prefrontal-subcortical loops by lacunes or white matter lesions located in subcortical areas, and the disrupted circuit may give rise to deafferentation of cortical or subcortical neurons. On the other hand, diffuse ischemia due to reduced CBF may directly damage the cortical neurons. Imaging studies using PET and SPECT have provided functional information in relation to regional metabolic patterns and CBF, useful for understanding the pathophysiology of SVaD.
One of the major concerns regarding studies of SVaD is that the large proportion of SVaD patients often has concomitant AD pathology (Jagust et al. 2008). With the recent development of amyloid imaging (especially Pittsburgh compound B (PIB)) (Klunk et al. 2004), amyloid plaque can be quantified in vivo. Therefore, SVaD patients without concomitant amyloid can be considered as having pure SVaD. Indeed, a study from our group has reported that 30 % of SVaD patients have concomitant AD pathology (Lee et al. 2011).
18.4.2 PET and SPECT in SVaD
PET and SPECT techniques can provide unique information regarding functional and neurochemical changes which occur early in the course of degenerative disease and is thus widely used for the differential diagnosis of dementia. PET can be used to measure regional oxygen (15O isotope) and glucose (18F isotope) metabolism, blood flow, and blood volume (Brooks 1991), while SPECT is also useful for measuring blood flow.
To better understand the pattern of CBF and the oxygen extraction fraction (OEF) in SVaD, the pathophysiological mechanisms involving microcirculation in small vessel disease need to be understood. Under normal conditions, autoregulation compensates for decreased CBF. That is, when cerebral perfusion pressure falls, blood vessels dilate to maintain rCBF. With further decline of rCBF, OEF increases to maintain rCMRO2 (Roman et al. 2002). Increased OEF is thus a transitional feature of ischemia. In SVaD, regional perfusion is reduced but OEF is preserved (Pakrasi and O’Brien 2005), and where blood vessels fail to dilate, autoregulatory reserve is decreased. This phenomenon can be seen in a study by Yao et al. using 15O PET in Binswanger’s-type dementia, which showed that CBF and CMRO2 were markedly reduced in both the white matter and parietal, frontal, and temporal cortices, while no abnormalities were detected by brain MRI (Yao et al. 1990). Tohgi et al. found increased regional OEF, decreased rCBF, and decreased rCMRO2 in patients with deep WMH, suggesting that oxidative metabolism is preserved relative to disturbed perfusion (Tohgi et al. 1998). The pattern of cerebral perfusion measured by SPECT using N-isopropyl-p-123I iodoamphetamine as a tracer showed that in Binswanger’s-type dementia, decreased perfusion was seen in the thalamus, basal ganglia, and frontal areas, whereas in AD dementia, decreased perfusion was seen in the parietal and temporal areas (Tohgi et al. 1991). A similar pattern was seen in another study using SPECT, which revealed that patients with Binswanger’s-type dementia had greater CBF reduction in frontal and anterior cingulate cortices, whereas patients with AD dementia had greater CBF reduction in temporoparietal and posterior cingulate cortices (Hanyu et al. 2004). In a study of SVaD, reduced rCBF was noted in the right thalamus, left caudate nucleus, and cingulate, bilateral superior temporal and left ventral subcallosal gyri (Yang et al. 2002). For the lacunar type of SVaD, a pattern of rCBF measured by SPECT showed similar patterns with Binswanger’s type (Shim et al. 2006). A typical pattern of CBF in a patient with SVaD is shown in Fig. 18.3.
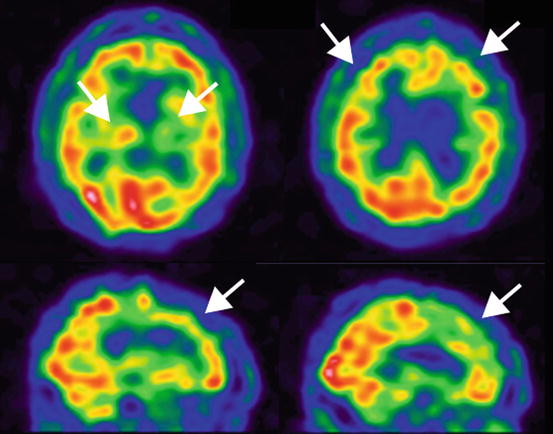
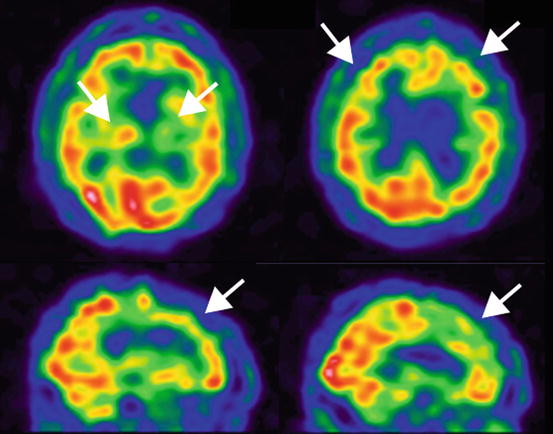
Fig. 18.3
Cerebral blood flow in a patient with subcortical vascular dementia. 99mTc-ECD SPECT in a 76-year-old man. Decreased rCBF was noted in bilateral frontal and basal ganglia (arrows) (Courtesy of Yong S. Shim (Department of Neurology, Bucheon St. Mary’s Hospital, Catholic University of Korea))
The pattern of glucose hypometabolism is widely used to differentiate various types of dementias, as impaired function usually precedes atrophy. With FDG-PET, SVaD patients show characteristic decreased metabolism in the frontal lobe and deep nuclei, whereas AD dementia patients show decreased metabolism in temporoparietal regions (Mendez et al. 1999; Pascual et al. 2010). Even in the svMCI stage, FDG-PET shows characteristic patterns in the subcortical type, distinct from that of amnestic MCI (Fig. 18.4) (Seo et al. 2009).
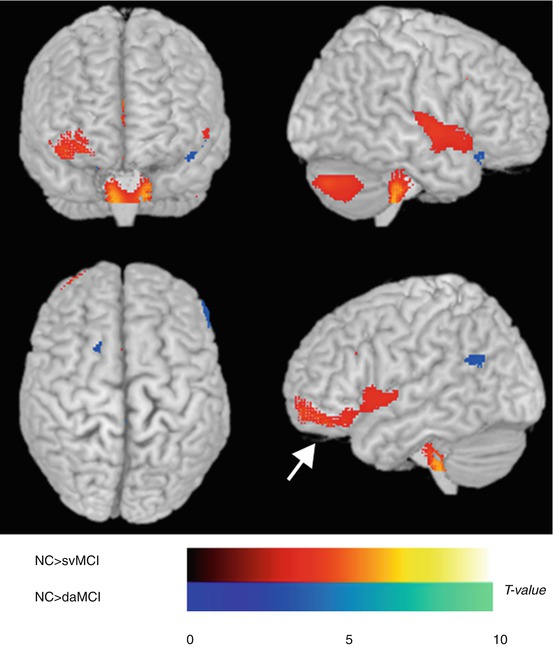
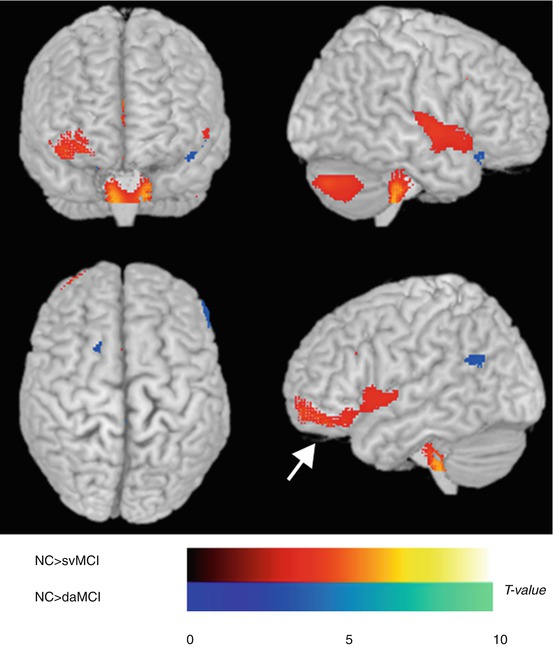
Fig. 18.4
Statistical parametric mapping analysis of regional glucose metabolism. Hypometabolic brain regions are depicted on a glass brain. The areas in red (arrow) represent the brain regions that were more hypometabolic in subcortical vascular MCI than in normal controls, and those in blue represent brain regions that were more hypometabolic in amnestic MCI than in normal controls (Seo et al. (2009), with permission)
Frontal executive dysfunction in SVaD can be further explained by the findings of a study using FDG-PET, which revealed that white matter lesions impair glucose metabolism in the frontal lobe regardless of their location (Tullberg et al. 2004). These findings are consistent with our results showing cortical thinning patterns in SVaD (Kim et al. 2012; Seo et al. 2010).
18.4.3 Amyloid PET in SVaD
With the recent development of amyloid imaging techniques, pure SVaD can be more appropriately identified (Lee et al. 2011). Two-thirds of SVaD patients have been found to be negative for PIB binding, and these patients are usually of younger age with lower MMSE scores, less lacunes, and less severe hippocampal atrophy compared to most SVaD patients positive for PIB binding (Fig. 18.5). The areas of PIB binding in SVaD patients are often similar to those of AD dementia patients (Lee et al. 2011).
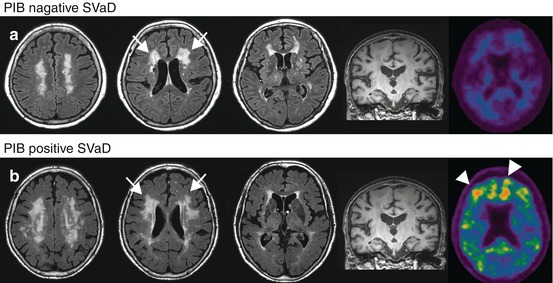
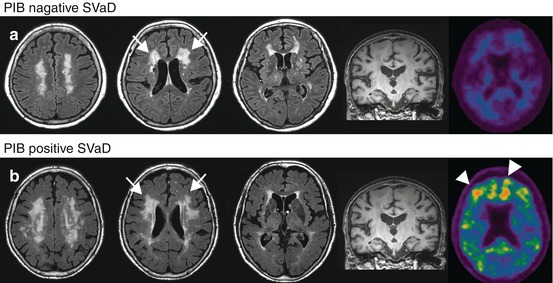
Fig. 18.5
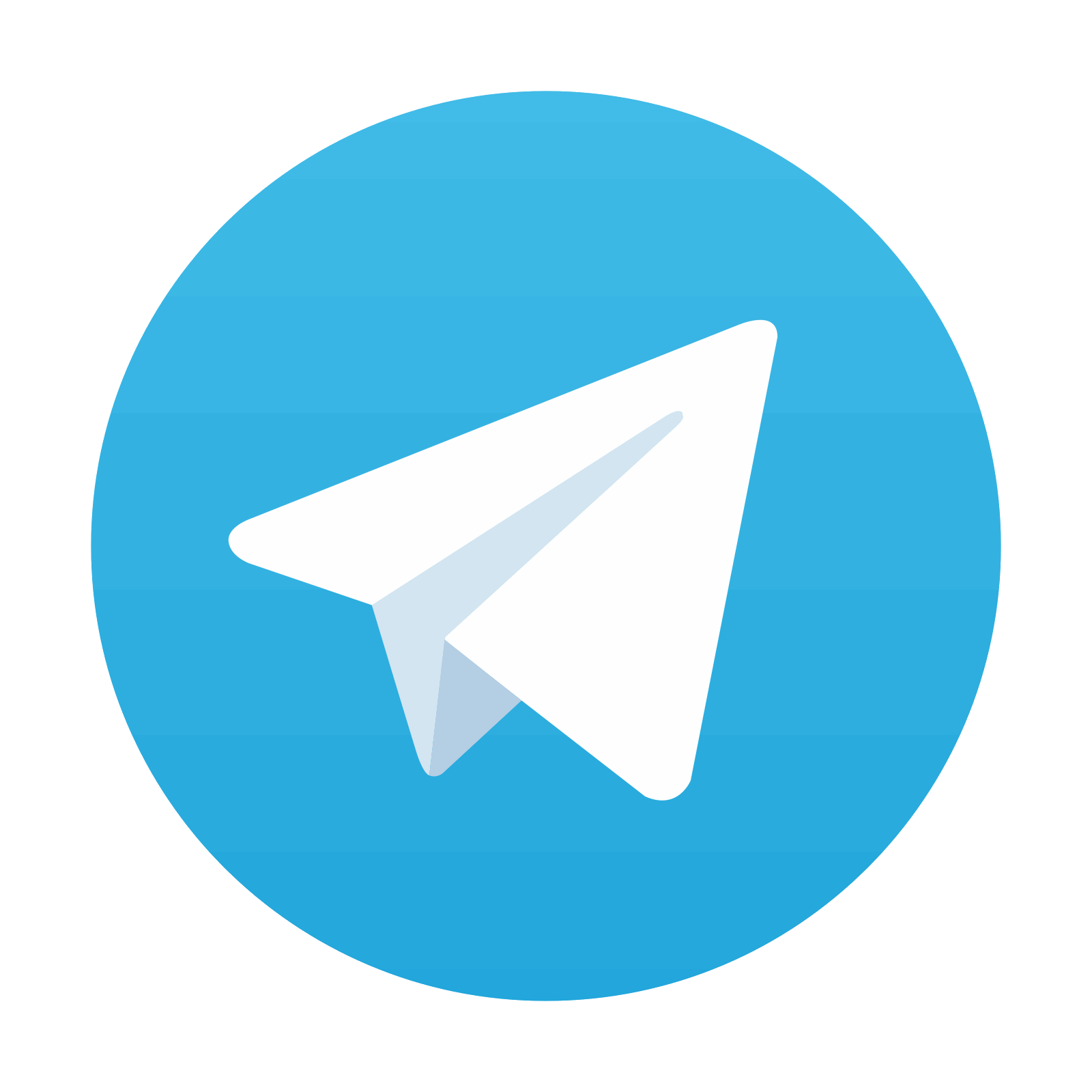
Brain MRI and PIB-PET of PIB-negative SVaD (a) and PIB-positive SVaD (b) patients. Both cases show extensive white matter lesions (arrows). However, PIB retention (arrowheads) was observed only in case (b) (Lee et al. (2011), with permission)
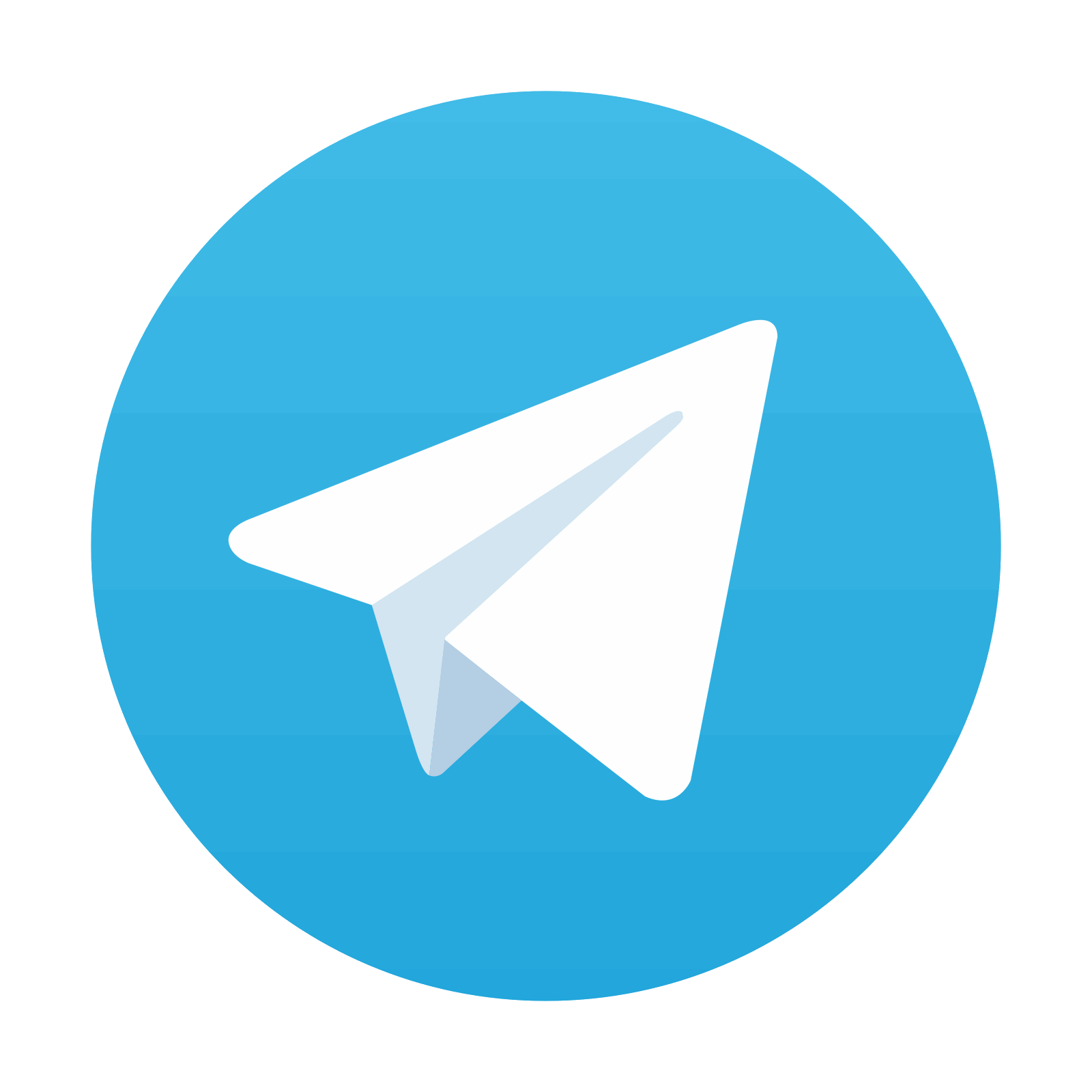
Stay updated, free articles. Join our Telegram channel

Full access? Get Clinical Tree
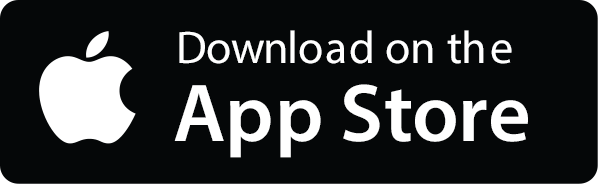
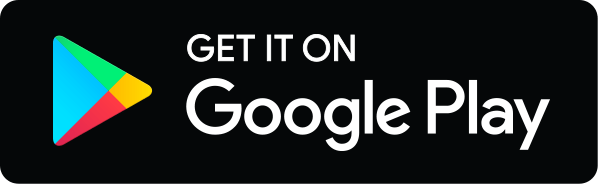
