Fig. 4.1
Vascular anatomy that is typically interrogated in bedside vascular ultrasound
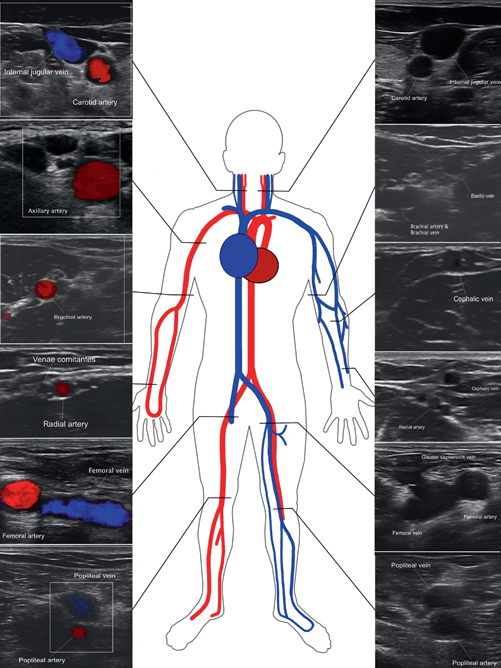
Fig. 4.2
Short-axis views of vascular anatomy typically interrogated in bedside vascular ultrasound
Venous Thromboembolism
Venous thromboembolism (VTE) represents a spectrum of disease, including both deep venous thrombosis (DVT) and pulmonary embolism (PE). DVT may present in the distal calf veins or more proximally involving the popliteal, femoral, or iliac veins. Clinical sequelae of DVT include: recurrence, post-thrombophlebitic syndrome, and chronic venous insufficiency. The most serious consequence of DVT is pulmonary embolism. It is estimated that over 90 % of cases of pulmonary embolism, emanate from the lower extremity veins [1, 2].
VTE is a common, yet often under recognized problem in the critically ill patient. These patients may have multiple risk factors for VTE that may be inherent, acquired, and/or treatment related. Rates of DVT in different ICU populations range from 10 % to up to 80 % and PE has been shown to be responsible for up to 15 % of in-hospital deaths [2–4]. Despite the increased incidence, DVT remains a challenge to diagnose in the critically ill. Clinical signs and symptoms of DVT may be absent or difficult to obtain in a sedated, mechanically ventilated patient. In the ICU population, studies have shown anywhere from 10 to 100 % of cases of DVT were clinically silent [4].
Diagnostic testing for DVT in the critically ill has its own challenges. Traditionally, clinical decision rules have embraced the use of d-dimer to determine the need for further diagnostic workup [5] Unfortunately, the use of highly sensitive d-dimer testing and traditional clinical prediction have been proven to not play a role in the ICU population [6, 7]. Contrast venography has long been considered the gold standard for diagnosis of DVT, however, this modality is technician–dependent, requires transport of potentially unstable patients, and maintains the risk of contrast-induced nephropathy [7]. Radiologist performed Duplex sonography of the lower extremities has been shown to be highly accurate for DVT in the general population with sensitivities ranging from 88 to 100 % and specificities from 92 to 100 % [8]. Similar to contrast venography, these studies are technician and radiologist dependent and may be difficult to obtain in a timely fashion.
There is evidence in the critical care and emergency medicine literature that clinician performed focused vascular ultrasound of the lower extremity is comparably accurate with reported sensitivities of 86 to 95 % and specificities of > 95 % [9–11]. The American College of Chest Physicians and the American College of Emergency Physicians recommend focused vascular ultrasound in their training curriculum [12, 13]. Furthermore, clinician-performed lower extremity ultrasound is rapid, reproducible and not technician-dependent which promotes rapid diagnosis and treatment of DVT.
History
The three general conditions for clot formation: stasis, hypercoagulability, and endothelial damage, were first noted in 1856 by a German physician, Rudolph Virchow. Virchow made the observation that clots found in the lungs on autopsy traveled from distant veins in the leg and coined these clots ‘embolia’ [14]. In his experiments, Virchow injected foreign bodies in the jugular veins of dogs to mimic clot traveling from the leg. Post-mortem, the foreign body was found encased in thrombus formed in-situ in the lung. Virchow theorized that the clot formed as a consequence of the foreign body , which caused: ‘irritation of the vessel’, ‘blood coagulation’, and ‘interruption of the blood stream’ [14].
It was not until late in the last century that these three factors were independently shown to cause thrombosis. Wound studies from World War I provided evidence that endothelial damage lead to thrombosis. Studies in the 1960s linked prolonged bed rest and stasis to the development of thrombosis. In 1965, the first inherited thrombophilia, anti-thrombin deficiency, was discovered [14]. It is controversial whether Virchow truly discovered the theory of thrombogenesis, however, his early observations have been acknowledged by numerous investigators and thus his triad stands today.
DVT in the ICU Population
The risk factors for VTE have expanded significantly from the original triad. ICU patients often present with known risk factors for VTE and may acquire more risk factors during the course of their stay. The most significant inherent patient risk factors are prior history of VTE and malignancy [15]. Mechanical ventilation is considered a risk factor for DVT due to diminished venous return from the heart as a consequence of positive pressure ventilation [15, 16]. Central venous catheters are a known cause of DVT with the relative risk increasing by 1.04 each catheter day [15, 16]. Surgical procedures with the highest rates of DVT include neurosurgical procedures and major orthopedic surgery of hip and knee [16, 17]. Rates of DVT post hip surgery or spinal cord surgery without prophylaxis have been reported to be as high as 50 and 90 %, respectively [16]. Finally, transfusions (especially platelets) and the administration of tranexamic acid are independent risk factors for DVT [3, 18].
Pathophysiology
The majority of lower extremity DVTs initiate in the lower extremity veins of the calf, specifically behind a valve in the soleal sinuses [19, 20]. These sinuses are a storage area for blood and feed the posterior tibial and peroneal veins. In the absence of calf muscle contraction, blood stasis occurs which leads to clot formation. It has been estimated that 40 % of these clots will spontaneously resolve, 40 % will organize into a stable clot, 20 % will propagate to the proximal lower extremity system, and a negligible amount will become pulmonary emboli [21]. About 80 % of calf vein clots are asymptomatic and these tend to occur most frequently in post-operative or immobilized patients [21].
Evidence has shown that compression ultrasound (CUS) without Doppler is sensitive and specific enough to exclude proximal DVT and it has become the first line test for diagnosing DVT [21, 22]. However, there remains controversy over how much of the lower venous system to scan. Crisp et al. advocate a rapid two-point compression US of the common femoral vein/saphenous junction and popliteal vein that has been shown to be 100 % sensitive for DVT above the knee [23]. Of note, these studies were done in symptomatic patients in a predominantly ambulatory setting. This limited approach has been shown not adequate enough for the critically ill, and it is recommended that imaging in the femoral region include a more comprehensive evaluation of the superficial femoral vein [12].
Some vascular labs routinely perform comprehensive evaluation of the lower extremity from the common femoral vein through the calf veins. CUS of the calf veins is more technically challenging, requires more training, and adds to the examination time [20]. In addition, sensitivity of CUS for calf vein thrombus has been reported at 60 to 80 % [7, 8]. Given this low sensitivity in the setting of a high-risk ICU population, a reasonable approach would be to perform serial CUS on days 3, 5, and 7 [24].This would potentially document any calf vein thrombus that subsequently organized and migrated to the upper leg veins.
Compression Ultrasound Technique
A high frequency, 5- to 10-MHz linear array probe is typically used. The obese patient may require use of the 2- to 5-MHz curvilinear probe for greater penetration. The patient should be supine in a reverse Trendelenburg position if clinically permissible to optimize venous return. Externally rotating the hip with the knee in flexion will facilitate compression in the inguinal region (Fig. 4.3).
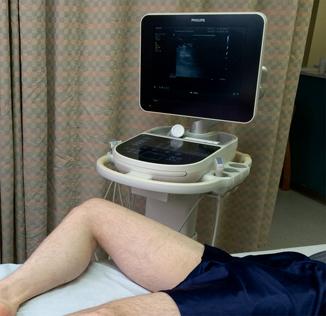
Fig. 4.3
Proper patient positioning for a lower extremity DVT ultrasound exam
Gel is applied to half of the transducer to confirm the location of the indicator in relation to the patient’s right side (Fig. 4.4). Once confirmed, the probe is covered with gel and applied in a transverse orientation to the inner aspect of the patient’s thigh slightly below the inguinal ligament. The common femoral vein and distally its confluence with the great saphenous vein will be appreciated medial to the femoral artery (see Fig. 4.2). The depth and focus on the ultrasound machine should be adjusted to optimize this view.
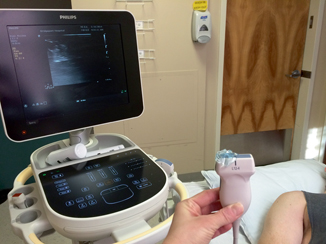
Fig. 4.4
Gel placed on half probe to confirm sidedness of study with patient and ultrasound machine
The lumen of the vein should be assessed for the presence of any haziness suggesting the presence of clot. If absent, graded compression should be applied externally to the thigh until the walls of the vein coapt and obliterate the lumen (Fig. 4.5). Lack of full compression is indicative of clot. The amount of compression needed to fully compress a patent vein may vary from patient to patient. In general, pressure which causes bending of the femoral artery should be sufficient for full venous compression.
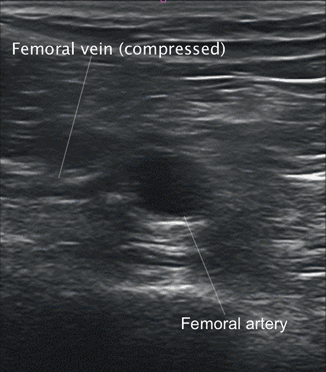
Fig. 4.5
Short-axis view showing compression of femoral vein
The probe is moved in transverse orientation down the inner thigh, compressing every 1–2 cm until the common femoral vein divides to form the femoral vein and the deep femoral vein. Graded external compression is applied in this area as well in 1- to 2-cm increments until the femoral vein passes into the adductor canal (about two-thirds of the way down the thigh) and is lost to further visualization.
The femoral vein resurfaces as the popliteal vein behind the knee in the popliteal fossa. This area is best visualized with the patient’s knee flexed about 45°. The popliteal vein will appear to be superior to the popliteal artery, however this is due to the posterior approach of the ultrasound probe (see Fig. 4.2). Graded compression in this area may be more difficult due to the smaller surface area and the potential instability of the flexed knee (Video 4.1). Supporting the patient with pillows may help stabilize the knee and facilitate scanning (see Table 4.1 for DVT ultrasound performance tips).
Table 4.1
Tips for maximizing success when performing ultrasound for DVT
Proper patient positioning: |
Hip externally rotated and knee flexed |
Support patient appropriately with pillows and/or blankets |
Consider reverse Trendelenburg if clinically acceptable |
Adjust height of bed or ultrasound machine to optimize operator ergonomics |
Appropriate probe selection for patient: |
High-frequency linear probe for non-obese patients |
Low-frequency curvilinear probe for adequate compression and penetration in obese patients |
Adjust depth and focus to maximize area of interest |
Compression: |
Begin gently and visualize paired vein and artery prior to compression |
Consider Doppler: |
Color Doppler may help define anatomy |
Spectral Doppler to demonstrate respiratory variation or augmentation |
Adjunctive Techniques
Technically difficult studies may benefit from the use of Doppler. Color Doppler is useful to confirm anatomy and/or the presence of clot. Pulsatile flow will distinguish the artery from the vein (Video 4.2) and lack of flow may be further evidence of venous clot or a confounding structure such as an abscess , hematoma, or lymph node .
Color Doppler may also used to demonstrate augmentation of the popliteal vein. External compression of the calf muscles will produce an increase in flow in the popliteal vein in the absence of DVT (Video 4.3) or a filling defect representing a DVT. Pulsed-wave Doppler may also be used to demonstrate respiratory variation seen predominantly in the common femoral vein in the absence of DVT (Fig. 4.6). Loss of respiratory variation in the common femoral vein may be suggestive of proximal thrombosis in the iliac vein.
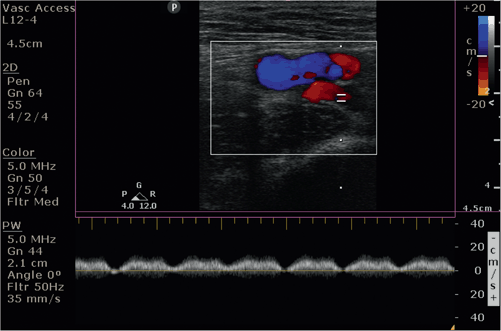
Fig. 4.6
Short-axis view with color-flow Doppler: Respiratory variation of the femoral vein
Upper Extremity DVT
Approximately 10 % of all DVTs occur in the upper extremity veins (subclavian, axillary and brachial veins) causing an estimated 7 to 17 % of Pes [25, 26]. Upper extremity DVTs are categorized as primary or secondary. Primary DVT may be caused by compression of the vein due anatomic abnormalities of the costoclavicular junction or injury to the vein in the setting of repetitive trauma or strenuous activity [25]. Secondary causes predominate in the ICU and include central venous catheters, malignancy, recent surgery, trauma, or cardiac procedure. Patients presenting with upper extremity DVT are more likely to have had a recent central venous catheter, cardiac procedure, infection, malignancy, or ICU stay [27]. The incidence of upper extremity DVT has increased concurrently with the increased use of central venous catheters particularly peripherally inserted central venous catheters (PICCs) [25–28]. Catheter characteristics which promote clot formation include luminal diameter, number of ports, incorrect tip positioning, and simultaneous infection [25].
Compression ultrasound of the upper extremity poses more challenges for the clinician operator. The anatomy of the upper extremity is more complex than the lower extremity with paired veins both above and below elbow (see Fig. 4.2). In addition, examination of the proximal axillary and mid subclavian vein is complicated by the presence of the clavicle that precludes compression of the vein. In lieu of compression, Color Doppler and spectral waveforms may be needed to demonstrate absence of clot. Flow in the upper extremity will appear biphasic at times due to the proximity of the heart as opposed to the monophasic flow seen in the lower extremities. Loss of biphasic flow in the upper extremity veins seen on spectral Doppler maybe suggestive of clot in the vein. Overall, the negative predictive value of CUS for upper extremity DVT is inferior to CUS for lower extremity DVT andadditional studies such as contrast venography, CT venography, or MR venography should be perused if there is continued clinical suspicion [25].
Pitfalls and Other Findings
Age of the Clot
Clot in the vessel often becomes more echogenic (hyperechoic) with age. However, slow blood flow may be echogenic as well and mimic clot. Use of color Doppler may help to distinguish what may appear to be clot prior to compressing the vessel. If color Doppler is limited due to slow blood flow, augmentation or the use of a tourniquet may enhance color Doppler signal. Acute thrombus is often not visualized at all in the lumen, which is why compression is imperative to make the diagnosis of DVT.
The Eye Does Not See What the Mind Does Not Know
The clinician should be aware of other pathology, which may be visualized during CUS. A Baker’s cyst is occasionally visualized in the popliteal fossa. This is a distension of the semimembranosus bursa and will appear as a cystic mass extending into the knee joint. Baker’s cysts have well-defined walls and will exhibit posterior acoustic enhancement. Color Doppler will demonstrate absence of flow. Rupture of the cysts will reveal fluid tracking into the subcutaneous tissue of the calf.
Other fluid collections such as abscesses and hematomas will appear to have an irregular shape and varying internal echogenicity with absence of flow with color Doppler. Soft tissue edema is characterized by the classic cobblestoning of the subcutaneous tissue, which may also be seen in the setting of cellulitis .
Point-of-Care Ultrasound as a Screening Tool
More ominous pathologies may be discovered including popliteal aneurysms, tumors, and arterial thrombus. The clinician should have a low threshold to refer any questionable or incidental findings for a formal radiology study.
Limitations of CUS in the ICU
Compression ultrasound of the proximal veins is most sensitive in patients who are symptomatic for DVT. In addition, CUS of the proximal veins precludes diagnosis of calf vein thrombus unless it extends into the popliteal region. Critically ill patients tend to be asymptomatic for DVT and have an elevated incidence of calf vein thrombus. Serial CUS at days 3, 5, and 7 is recommended if the initial study is negative. Finally, CUS may be technically challenging due to patient dressings, casts, limited mobility and patient size. If clinical suspicion is strong enough, alternate imaging such as venography, CT venography, or MR venography should be pursued.
Conclusions
The use of bedside ultrasound to diagnose DVT in critically ill patients is supported by the literature. Because of the body habitus challenges that may be encountered in some of the sickest patients, it is important for clinicians to scan a wide variety of patients regularly in order to understand vessel responsiveness to CUS, Doppler flow, and augmentation maneuver response in both pathologic and non-pathologic situations.
Ultrasound-guided Vascular Access
Adequate vascular access is a cornerstone to the management of a wide range of critical illness states. Given the importance of early resuscitation and restoration of adequate perfusion, the insertion of indwelling vascular catheters must be performed as efficiently as possible. Strategies for approaching this issue have historically relied on either superficial structures and their relation to underlying vascular anatomy or the direct visualization of vessels millimeters below the skin. Although these approaches to access are time-tested, practitioners of ultrasound have since questioned how well the classical methods are in achieving any given access. Overall, the widespread deployment of ultrasound has led an overall improvement in the successful establishment of access in diverse care settings. The following is a review of the modern usage of ultrasound for vascular access in critically ill patients.
Central Venous Catheters
Central venous catheters remain a popular means of vascular access in the intensive care unit. It is estimated that over 5 million central venous catheters are placed yearly in the United States [29]. With ultrasound becoming more widely available, several studies have demonstrated its efficacy, efficiency, and safety which has lead some organizations to advocate for ultrasound-guided technique as the standard of care when placing central venous catheters [30, 31]. Although placement related complications may have been significantly reduced through the use of ultrasound, cannulation of the central veins remain a source for significant infectious morbidity in the intensive care setting [32]. It is estimated that 80,000 bloodstream infections occur yearly which have been shown to not only increase hospital length of stay, but also increased health care costs, and possibly increased risks of death [33, 34]. Given that several indications for central venous access remain absolute (i.e., parenteral nutrition, hemodialysis, central medication administration, and hemodynamic monitoring), the use of central lines continue to be considered an “imperative” in the treatment of critically ill patients.
Two of the most common types of catheters used in the intensive care setting have received a significant amount of focus in the literature: Centrally inserted, non-tunneled central venous catheters, and peripherally inserted central catheters (PICCs) . Each have their own particular risk/benefit profiles and may be more or less beneficial to different patient populations.
Centrally Inserted, Non-Tunneled Central Venous Catheters
Although the concept of intravenous access as a means of administering blood and other “medicinal substances” has been known for centuries, the idea of obtaining access into the central venous circulation has only existed since the early 1950s [35]. Aubaniac has been described as the first person who published the method of accessing the subclavian vein for the purposes of resuscitating war victims in 1952 [36]. Shortly after this, descriptions of primary and adjuvant methods of access techniques entered the literature: Seldinger described wire-guided placement of catheters in 1952 [37]. Yoffa described the supraclavicular approach to subclavian access in 1965 [38], and Dudrick et al. described the successful delivery of parenteral nutrition via the central veins of puppies (1966) then humans (1967) [39, 40]. It wasn’t until 1978 when the use of ultrasound, then in the form of Doppler, was used to locate the internal jugular vein for the purpose of guiding central venous catheter placement [41]. In 1986, Yonei et al. reported their experience of using real-time, ultrasound guidance to place internal jugular central venous catheters [42]. In their letter to the editor, these authors reported no complications encountered with internal jugular central line placement over the span of 2 years [42]. Since this report, the use of ultrasound has been explored as a means of improving the safety of central line placement.
When accessing the central veins, several complications have been described when using traditional landmarks as a means of guiding access placement. In the 1970s and 1980s, the incidence of pneumothorax, arterial puncture, and hematomas have been described in 5 to 21 % of patients and unsuccessful cannulation was reported in as many as 35 % of patients [43–46].Since these early reports, practitioners began to ask whether ultrasound would be able to mitigate against the incidence of these complications. By 2003, as reported in a meta-analysis by Hind et al., several studies comparing ultrasound vs. landmark techniques showed fewer failed catheter placements, fewer complications, fewer attempts to successful access and quicker access rates using ultrasound depending on the site of cannulation [47]. Specifically, the internal jugular (IJ) had the most supportive evidence in favor of the superiority of ultrasound-guidance over landmark. As the technology become more available in a variety of care settings, ultrasound continued to repeatedly show its merits in the realm of safety and efficiency of access [48]. As a result, ultrasound-guided central venous access has not only been advocated as the standard of care in ICU settings, but ultrasound education has become an important component of resident training [31].
When placing a non-tunneled, central venous catheter using ultrasound , several techniques have been described to maximize success rates (see Table 4.2 for a summary). First, ideal patient positioning has been extensively studied using ultrasound to measure the diameter of the target vessel. For right subclavian approaches, maximal cross sectional area of the vein has been achieved in healthy subjects in the Trendelenburg position, shoulders neutral, with the head turned away from the proposed area of puncture [49]. For the left subclavian, maximal diameter can be achieved in Trendelenburg position with the head and shoulders neutral [50]. For internal jugular access, 15° of Trendelenburg, a small head support, and the rotation of the head close or at midline can maximize the diameter of the IJ [51], however, no head rotation has been shown to be as safe as head rotation 45° away from the side of puncture [52]. For femoral access, reverse Trendelenburg can be beneficial to maximizing the vein’s diameter [53]. Given that many of these studies were conducted on either healthy subjects and/or patients that were able to give informed consent, these ideals may not be achievable in all clinical settings, however, they can serve as a useful foundation that can be tailored to fit the situation.
Table 4.2
Tips for maximizing success in ultrasound-guided central venous access
Use a higher frequency (12 MHz) linear probe with the depth set to 3–6 cm |
Position patient appropriately (see text) |
Prepare skin with chlorhexadine |
Ensure differentiation of venous versus arterial structures through their response to compression; veins should easily compress completely and arteries should remain patent and pulsatile with moderate compression |
Ensure location of the tip of the access needle constantly by moving the ultrasound probe in parallel with the advancement of the needle |
Following placement of guidewire through puncture catheter, confirm intravenous course of guidewire using ultrasound prior to dilation and catheter placement |
Following securement of catheter and lumen flushing, line course and location can be confirmed through ultrasound interrogation of the adjacent veins and through saline flush ± air bubble enhanced echocardiography |
Consider pneumothorax or hemothorax evaluation using ultrasound |
How to position the ultrasound probe during central line placement has also been studied. When accessing the vessel, proceduralists can either ultrasound the vessel, remove the probe, and mark the skin at the proposed site of access (the “quick view” approach) or use the ultrasound images to guide the needle directly into the vessel. Airapetian et al. has shown that real-time guidance of internal jugular puncture can have a lower incidence of access related complications and increased success rates as opposed to the “quick view” approach [54]. Additionally, the incidence of catheter bacterial colonization is the same in the two techniques if performed using sterile technique [54]. When imaging a central vein, an operator can guide cannulation by means of a short-axis view (also known as the cross-sectional or transverse view; Fig. 4.7a) or a long-axis view (also known as the longitudinal view; Fig. 4.7b). Tammam et al. has shown that by using either view to guide access, there are fewer complications than standard landmark approaches to the IJ, however, there were no significant differences in access time, success rate, number of attempts, or mechanical complications between the two different ultrasound-guidance views [55]. Taking all this data into account, the authors of this chapter have been successful using the short-axis view and moving the probe to follow the progress of the needle. This allows for real-time imaging of the progression through structures/hazards superficial to the vessel. Regardless of approach, the use of ultrasound provides an added ability to visualize what happens below the surface of the skin that allows for an overall safer experience than relying on superficial features.
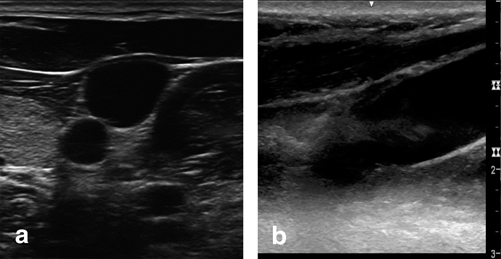
Fig. 4.7
Short-axis view (a) and long-axis (b) ultrasound views of the internal jugular vein. Images Video by Paul Possenti, PA
The modality to confirm the course and final position of central lines placed above the waist has traditionally been the post-procedural chest radiograph. Complications such as pneumothorax , hemothorax , and aberrant line courses can be readily visualized by this simple bedside study, however, there may be time delays depending on the responsiveness of the radiographer. Since bedside ultrasound has shown efficiency in the placement of central lines, questions have surfaced regarding its use in detecting placement related complications in comparison to chest x-ray (CXR). In one example, inadvertent arterial access and cannulation is a complication that may not be picked up until the abnormal course of the central line is observed on CXR. Gillman et al. have reported that by confirming that the guidewire is not inside the artery, one can ultimately avoid accidental tract dilation and arterial cannulation [56]. As a means of confirming the final course of a line, several studies have described different approaches. Direct visualization of intravenous catheter course can be combined with echo to evaluate whether the tip sits above or within the right atrium [57, 58]. As an adjunct that can enhance either direct or nearby tip visualization, saline injected with or without a small volume of bubbles through the catheter can be visualized on an echocardiographic view of the right atrium [59, 60]. To assess for pneumothorax , ultrasound has been described as a useful tool for diagnosis, however, given the relatively low incidence of pneumothorax following line placement, only a limited experience of its use has been reported [57, 58]. Overall, the bedside diagnosis of a variety of line related complications can be made through the use of ultrasound and taking the time to learn such methods may allow for earlier interventions.
In summary, ever since the 1950s central venous access has become a key component of managing critically ill patients. Placement safety and efficiency can be augmented with ultrasound. Other factors such as ideal patient positioning, probe positioning, and adequate experience can maximize the success of the process while hopefully reducing the incidence of complications.
Peripherally Inserted Central Venous Catheters (PICCs)
PICCs have been used in both the outpatient and inpatient settings. As a device, a PICC maintains the appeal of potentially minimizing patient discomfort while providing a “longer term” access for essential medications. In regards to placement, both nurses and physicians have published reports regarding the successful development of bedside ultrasound guided PICC services throughout the world [61, 62]. Despite their attractiveness, these catheters have been shown to potentially have significant complications when used in critically ill patients. Given that the catheter passes through relatively smaller diameter superficial veins on its way to the larger central venous system, stasis and/or localized damage could occur thus producing thrombosis and/or phlebitis. In one review, the incidence of these two complications among all hospitalized patients may be higher with PICCs as compared to standard central lines [63].Among intensive care patients, similar concerns of thrombotic complications in PICCs are highlighted through several reports [64–66]. Of note, there may be some populations (i.e. burns) that may not have as significant of a problem [67].
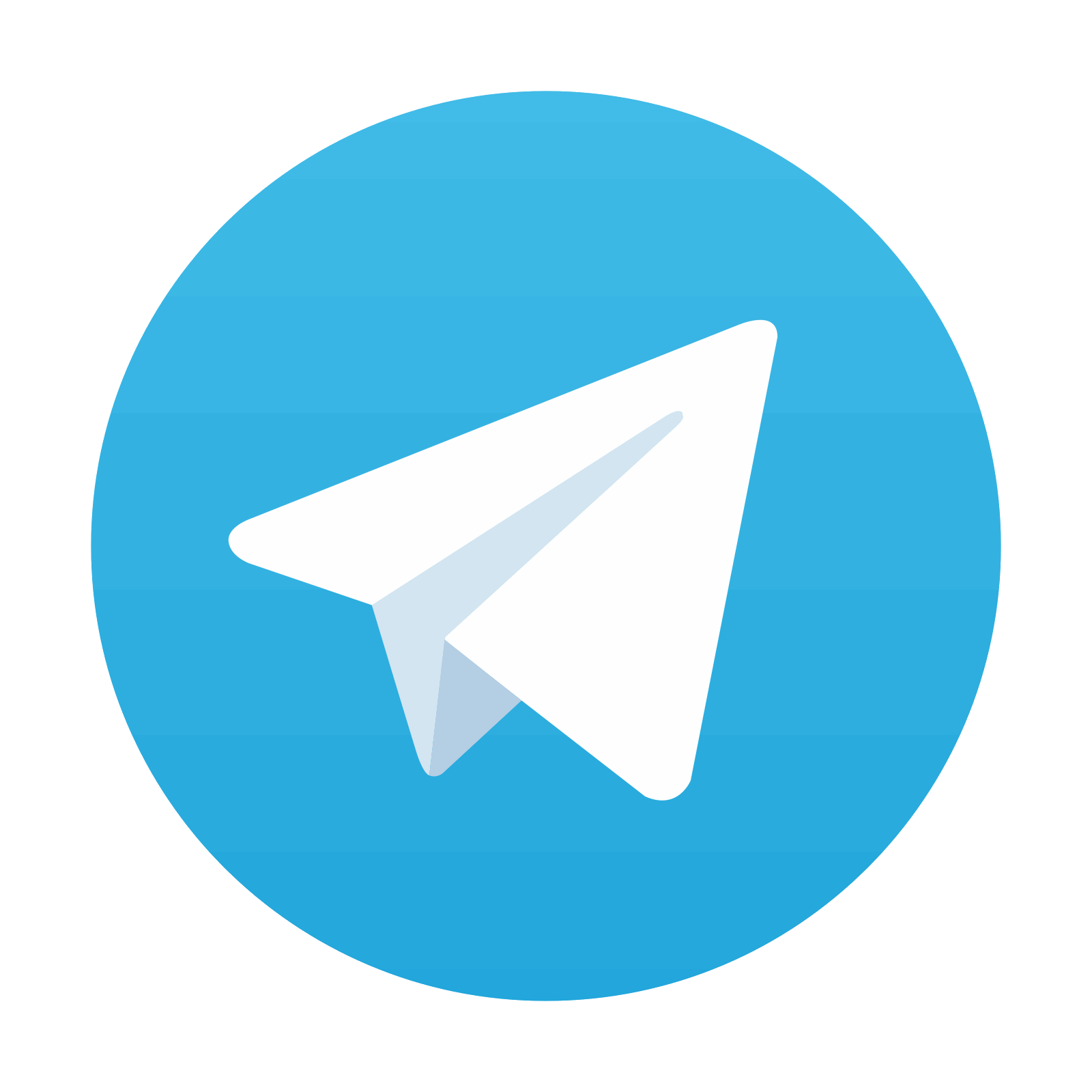
Stay updated, free articles. Join our Telegram channel

Full access? Get Clinical Tree
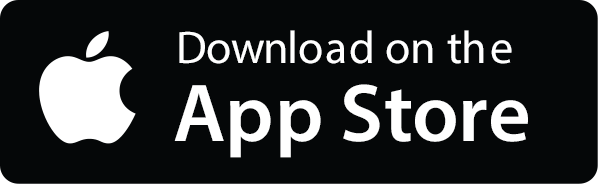
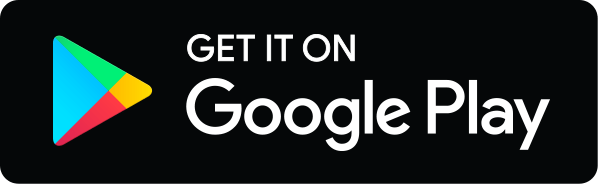