Vertebral Column
Esperanza Pacheco-Jacome
Kevin R. Moore
Sara O. Vargas
L. Santiago Medina
INTRODUCTION
Comprehensive imaging evaluation and characterization of vertebral column disorders is essential in the pediatric population. Proper selection of imaging and technique, which can narrow the differential diagnosis, is important for patient outcome. In this chapter, the currently available imaging modalities and important disorders affecting the vertebral column in infants and children are discussed. The characteristic imaging findings and management of congenital and acquired disorders involving the vertebral column in pediatric patients are also reviewed.
IMAGING TECHNIQUES
Radiography
In the evaluation of the vertebral column, radiographs still play an important role. They allow adequate labeling of the vertebrae (i.e., thoracic vs. lumbar) and global evaluation of the bone. Deformity and segmentation abnormalities can be accessed with erect frontal and lateral radiographs of the entire spine with spot images as needed.
In the cervical spine, ideally, a three-view examination including frontal, lateral, and open-mouth views should be obtained. Open-mouth view can be difficult to obtain in patients <5 years or uncooperative pediatric patients. In the thoracic spine, a minimum of a three-view examination including frontal, lateral, and swimmer views should be obtained in order to evaluate the cervicothoracic junction in detail. In the lumbar spine, a minimum of a four-view examination including frontal, lateral, and both oblique views should be obtained. Properly obtained oblique views are important in accurately evaluating the pars interarticularis for spondylolysis. Posteroanterior scoliosis radiographs decrease radiation exposure to the patient. Bending films in the scoliosis survey can be important for surgical planning.
Newer low-dose radiation technology, including low-dose radiation digital stereoradiography system (EOS orthopedic imaging system), allows conventional and 3D reconstructions of the spinal column in a weight-bearing standing or sitting position, allowing assessment in natural posture. This new technology delivers 8 to 10 times less radiation than conventional scoliosis radiographs.1 3D reconstruction in the erect position can also be obtained.2
Ultrasound
Ultrasound in the newborn period is useful to access the spinal canal contents, which is discussed in Chapter 4 of this book. However, the role of ultrasound in evaluating the osseous spinal column is very limited.
Computed Tomography
In the setting of acute trauma with no neurologic deficit, radiographs followed by computed tomography (CT) are the studies of choice. These allow detailed evaluation of the alignment and integrity of the vertebral column. For craniocervical pathology, such as basilar invagination or complex vertebral column anomalies, the combination of CT (with multiplanar and 3D reconstructions) and magnetic resonance imaging (MRI) allows detailed evaluation not only of the osseous structures but also of the soft tissues and spinal contents including the spinal cord.
Newer multidetector CT (MDCT) with dose reduction technique is ideal because of its ability to cover a long scan
range in a few seconds, reducing the need for sedation. The best results are obtained with 3-mm-thick multiplanar reconstructions. 3D reconstructions are always valuable because they allow optimized visualization of all anatomic structures and confirmation of the exact level of pathology. Because of the high complexity of some vertebral anomalies, CT findings should be correlated with radiographic findings. In addition, counting is ideally initiated from C1 or C2, and exact reference levels must be reported in detail in order to avoid unnecessary confusion and risk of error, especially when surgical intervention is being considered.
range in a few seconds, reducing the need for sedation. The best results are obtained with 3-mm-thick multiplanar reconstructions. 3D reconstructions are always valuable because they allow optimized visualization of all anatomic structures and confirmation of the exact level of pathology. Because of the high complexity of some vertebral anomalies, CT findings should be correlated with radiographic findings. In addition, counting is ideally initiated from C1 or C2, and exact reference levels must be reported in detail in order to avoid unnecessary confusion and risk of error, especially when surgical intervention is being considered.
CT Myelography
In pediatric patients with prior spine instrumentation, a CT myelogram may be required to evaluate the hardware and spinal canal because MRI is often limited by susceptibility artifact. In children with titanium hardware, MRI should be attempted first, because these metallic devices produce less susceptibility artifact than conventional ferrous hardware. Use of titanium instrumentation is currently limited but growing. In pediatric patients with complex meningoceles and vertebral column anomalies, CT myelography can be important in surgical planning to determine the exact relationship of the vertebral column, nerve roots, and cord with respect to the meningocele.
Magnetic Resonance Imaging
MRI plays an important role in the evaluation of the spinal column and its bone marrow. Familiarity with the normal progression of red to fatty marrow according to age is paramount in understanding normal bone marrow patterns and underlying pathology. T1-weighted, T2-weighted, and short tau inversion recovery (STIR) sequences are useful in determining normal bone marrow patterns versus early bone marrow disorders or edema. Pre- and postcontrast T1-weighted MR imaging including fat-saturated sequences allows further characterization of bone marrow-centered lesions. Diffusion-weighted (DW) MR imaging with its appropriate apparent diffusion coefficient (ADC) map is useful in evaluating cystic lesions when the differential diagnosis of dermoid/epidermoid versus arachnoid cyst is being considered. Restricted diffusion is more often seen in dermoid and/or epidermoid cystic lesions.3
Nuclear Medicine
18F-FDG-PET plays an important role in the evaluation of metastatic disease to the spine. Familiarity with differences in PET appearance of spinal column bone metastatic disease and pharmacologically induced bone marrow stimulation is of crucial importance. Metastatic disease tends to be more focal and asymmetric, whereas bone marrow-stimulating agents tend to cause more diffuse and symmetric uptake.
Bone scintigraphy with spine 3D SPECT has a special role in evaluating nonfebrile back pain in the older child or adolescent with suspected spondylolysis. Stress changes associated with active pars defects are well-visualized as areas of focally increased radiopharmaceutical uptake.
EMBRYOLOGY OF THE VERTEBRA
The development of the vertebral column passes through three different stages: (1) membranous (precartilaginous) stage, (2) chondrification, and (3) ossification.
Membranous Stage
The intraembryonic mesoderm, (derived from the primitive neuroectoderm) located on each side of the notochord (a mesodermal structure arising from the Hensen node, located between the primitive ectoderm and endoderm) and neural tube, forms a longitudinal column of paraxial mesoderm. By the end of the 3rd week, the paraxial mesoderm divides into somites, located on each side of the developing neural tube and notochord.4 There are 44 pairs of somites aligned rostrocaudally. The first somite is transient, somites 2 to 4 form the basiocciput, and the others form the axial skeleton, associated musculature, and adjacent dermis of the skin.
During the 4th week, cellular migration occurs. At each somite level, the dermatomes form dorsolaterally, myotomes form medially, and sclerotomes form ventromedially. The sclerotomes (spine) then migrate in three different directions at each level, maintaining their segmental arrangement. Ventromedially, cells migrate and surround the notochord, forming the membranous vertebral column that separates the notochord from the neural tube and gut. Ventrolaterally, cells form two processes: costal (ventral), which later gives origin to the thoracic ribs, and lateral, which gives origin to the transverse processes. Dorsally, cells form the vertebral arches behind the neural tube4,5,6 (Fig. 5.1).
On about day 24, the process of resegmentation occurs at the level of the membranous vertebral bodies. Each sclerotomic segment differentiates into a cephalic portion (less condensed) and a caudal portion (more condensed). In the midportion of each sclerotome appears a horizontal sclerotomic cleft (fissure of von Ebner), where each sclerotome divides. The caudal half of each sclerotome then fuses with the cephalic half of the subjacent sclerotome, forming the precartilaginous vertebral centrum. The intervertebral discs form during this time, from the denser caudal portion of the sclerotome. Cells from the caudal portion move cranially to the middle part of each segment, forming the peripheral part of the disc; the annulus fibrosus, which surrounds the notochord, and the enchondral growth plates of the vertebral centra. The intersegmental arteries that were between the somites now enter the center of the forming vertebral bodies.5,6,7 The process of resegmentation occurs bilaterally and symmetrically and probably starts at the thoracic region, progressing toward both ends of the embryo (Fig. 5.2).
Chondrification
During the 6th week, chondrification centers appear in the membranous vertebra. The chondrification centers first develop at the cervicothoracic level and then extend downward into the caudal spine. Two chondrification centers appear in each centrum (body) and fuse at the end of the 8th week to form a cartilaginous centrum. The chondrification centers for the neural arches and pars (one on each side of the vertebra) develop later than the centrum, eventually fusing with each other and with the centrum. The transverse and spinous processes develop from extension
of chondrification centers in the vertebral arches. With formation and joining of the chondrification centers, there is squeezing of the notochord cells and intervertebral tissue into the disc spaces where the notochord becomes the nucleus pulposus of the disc.4,7
of chondrification centers in the vertebral arches. With formation and joining of the chondrification centers, there is squeezing of the notochord cells and intervertebral tissue into the disc spaces where the notochord becomes the nucleus pulposus of the disc.4,7
Ossification
Ossification begins during the 8th week and ends at about 25 years of age. In the prenatal period, three centers appear by the end of the embryonic period (8 weeks): one for the centrum (body), divided into anterior and posterior components; one ossification center for each pars; and one for each half of the vertebral arch. At birth, each vertebra consists of five bony parts connected by cartilage: the vertebral body, two halves of the neural arch, and two pars ossification centers. The ossification centers do not appear simultaneously at the same level. At 8 weeks, the ossification centers of the posterior arches are first seen in the cervical region, while the vertebral bodies are seen in the lower thoracic and upper lumbar levels, which quickly propagate cranially and caudally4,7 (Fig. 5.3). During the postnatal period, the halves of each vertebra fuse between years 3 and 5. The arches articulate with the centrum at the cartilaginous neurocentral joints, which grow as the cord enlarges.
Variations from this pattern of ossification occurs at the C1 and C2 level, where the ossification center of the C1 body forms the odontoid process of C2 and the ossification center of the arch of C1 joins anteriorly to form a ring.4 The C1 vertebra has no vertebral body, and there is no intervertebral disc between C1 and C2.4
C1 (atlas) is formed by three ossification centers, which include the anterior arch and two neural arches. The anterior arch is ossified in about 20% of neonates and in 80% by 1 year of age. The posterior neural arches appear by the 7th week of the fetal life, and by age 3, they fuse posteriorly. The posterior and anterior arches fuse around 7 years of age (Fig. 5.4).
At birth, C2 (axis) has four ossification centers: one for each of the two neural arches, one for the body, and one for the odontoid process. In fetal life, the odontoid process forms from two ossification centers that fuse by month 7. The os terminalis is a secondary ossification center at the tip of the odontoid process that fuses by age 12. The odontoid process fuses with the body of C2 by 3 to 6 years of age. The interface of the odontoid with the body is known as the subdental synchondrosis, which may be seen until age 11 and could be mistaken for a fracture. The neural arches fuse posteriorly by age 2 to 3 and anteriorly with the odontoid process between 3 and 6 years of age8,9 (Fig. 5.5).
After puberty, there are multiple secondary vertebral ossification centers, which include the tip of the spinous process, the tip of each transverse process, and the superior and inferior rims of each vertebral body. By about 25 years, the secondary centers fuse with the rest of the bone4 (Fig. 5.6).
ANATOMY OF THE VERTEBRAE
As described earlier, the bony vertebrae originate from primitive mesoderm, which surrounds the neural tube and notochord. Usually, there are 7 cervical vertebrae, 12 thoracic, 5 lumbar, 5 sacral, and 4 or 5 coccygeal segments. One ossification center appears for each sacral segment in childhood and adolescence. S2 occurs at about 5 to 10 years, S3 occurs from 10 to 15 years, and S3 may occur as late as 20 years.10 By adulthood, the sacrum has become a single bone.
Transitional anatomy, sometimes with supernumerary or deficient vertebrae, may be seen at the lumbosacral junction (“sacralization” of L5, “lumbarization” of S1), in the cervical, thoracic, or lumbar spine, or in the ribs (cervical rib(s), absent 12th rib(s)).
The morphology of the C1 and C2 vertebrae differs from the other vertebrae and deserves a special discussion. C1 (atlas) is the first spinal vertebra and, in conjunction with C2, connects the skull to the spine. The anterior arch presents a central anterior tubercle for the attachment of the longus colli muscle and anterior longitudinal ligament. Inside the arch is the fovea dentis, a place for the C1 articulation with the odontoid process. The posterior arch is the site of attachment of the posterior atlanto-occipital membrane. It has a groove-foramen for the vertebral artery and ends in a posterior tubercle, which is a rudimentary spinous process. The lateral masses support the weight of the head. They include the superior facets, which articulate with the occipital condyle and allow anterior-posterior movement. The inferior facets articulate with C2 (axis) and allow rotatory movement (Fig. 5.7).
C2 or axis has a special feature, the odontoid process (dens) that articulates with C1. The superior articular facets are mildly convex and oriented upward and laterally. The inferior facets articulate in the same direction as the other cervical vertebrae. The transverse processes are small and perforated, containing the vertebral foramina. The laminae are thick, and the spinous process is large and strong, presenting a bifid end (Figs. 5.8 and 5.9).
The thoracic and lumbar vertebrae are morphologically similar to one another, having an anterior mass or body, a posterior ring or arch, two transverse processes, two superior and inferior articulating facets, and a single posterior spinous process. The thoracic vertebrae have additional costal processes that articulate with the ribs11 (Fig. 5.10).
In ˜50% of neonates, the vertebral bodies have an ovoid configuration on radiography, with a fairly square shape in the thoracic spine. On the lateral view, the vertebrae have a “bone-within-bone” appearance. This configuration is also common in premature infants. There is a single fused ossification center in the vertebral body of a neonate and one ossification center in each neural arch. Anterior and posterior clefts of the vertebral bodies of neonates may be secondary to vascular channels. The anterior cleft may persist for a time in the thoracic spine, and the posterior cleft may be seen in adults. The junction of the neural arches is cartilaginous, which may be mistaken for spina bifida on radiographs. Normal developmental clefts are seen in the neonatal lumbar spine and, to a lesser degree, in the thoracic spine. There is a coronal cleft, which is thought to be related either to a notochord remnant or lack of fusion of the anterior and posterior ossification centers. It is present more often in boys and disappears in a few weeks or months10(Fig. 5.11). The oval configuration of the vertebrae remains approximately until 2 years of age, and then, the vertebrae become rectangular with rounded corners,
which are filled with non-radioopaque cartilage. Later in childhood, further changes in the spine occur, eventually leading to the adult morphology.
which are filled with non-radioopaque cartilage. Later in childhood, further changes in the spine occur, eventually leading to the adult morphology.
![]() FIGURE 5.8 C2 vertebral anatomy. A, Odontoid process (dens). B, Body. C, Superior articular facet. D, Transverse process. E, Inferior articular facet. F, Spinous process. G, Lateral mass. H, Lamina. |
![]() FIGURE 5.9 Lower cervical vertebral anatomy. A, Body. B, Transverse process. C, Vertebral foramen. D, Superior articular facet. E, Lamina. F, Spinous process. G, Lateral mass. |
![]() FIGURE 5.10 Lumbar vertebral anatomy. A, Body. B, Pedicle. C, Transverse process. D, Articulating apophysis. E, Lamina. F, Spinous process. |
![]() FIGURE 5.12 Vertebral morphology of the older child. A: The configuration of the vertebral body is more square in a 2 1/2-year-old child when compared to the ovoid neonatal shape (Fig. 5.11A). B: Square vertebral morphology in a 14-year-old boy. Ring apophyses (arrow) are demonstrated at the superior and inferior endplates. |
The cervical vertebral bodies develop cortical borders superiorly and inferiorly, and the transverse processes enlarge. The lumbar vertebral bodies develop superior and inferior concavity. The ring apophyses begin to appear in the midthoracic and upper lumbar spine at about 6 years of age. They are prominent in later childhood and fused to the vertebral bodies by age 18. These apophyses appear at the corners of the vertebra with a “C”-shaped configuration, and with vertebral growth, a beaked appearance may result10,12 (Fig. 5.12).
BONE MARROW AND INTERVERTEBRAL DISC
MRI is the modality of choice for evaluating the bone marrow in the developing pediatric spine. In the neonate, the bone marrow is markedly hypointense on the T1-weighted MR images when compared with the intervertebral disc. The vertebrae are hypo- to isointense on the T1-weighted MR images during the first year of life, and then iso- to hyperintense from 1 to 5 years. Subsequently, the vertebrae are hyperintense to the disc on the T1-weighted MR images in older pediatric population. The neonatal anterior vertebral vascular channel disappears in childhood, while the posterior channel may persist into adulthood. Until 6 months of age, the cartilage of the vertebral endplates is hyperintense. Then, it becomes isointense to muscle until 2 years of age, after which time the cartilage is not well seen due to further ossification. The spinous process is initially T1 hypointense and becomes hyperintense after 6 months of age. Bone marrow fatty deposition occurs in stress-related situations that might be seen as a normal variant in the lumbar spine in adolescents13 (Fig. 5.13).
Two cartilaginous articular plates, the fibrous ring or annulus fibrosus, and the nucleus pulposus compose the intervertebral disc. The annulus fibrosus is made up of connective tissue lamellae that extend from one vertebral surface to the next. The nucleus pulposus is a notochord remnant compressed in the central portion of the disc. In the newborn, the nucleus pulposus is ovoid, and the annulus fibrosus and the cartilaginous endplates are large. By the second decade, an anterior notch forms in the nucleus pulposus, giving it the shape of a thick “C.” By this time, the annulus fibrosus bulges convexly into the cartilage, which has thinned significantly.13
SPINAL GROWTH
The neonatal spine when viewed laterally has a subtle cervical and lumbosacral lordosis with a gentle thoracic kyphosis. The cervical curvature accentuates with development of head support in the first year of life, and the lumbar lordosis with ambulation by 2 years of age. Then, it becomes more prominent during childhood. The final normal curvatures of the spine typically become fixed after puberty.10,12
In the neonate, the cervical spine is 25% of the total spinal length, the thoracic spine 50%, and lumbar spine 25%. By adulthood, the proportions change: the cervical spine comprises one-fifth to one-sixth of the length, and the lumbar segment increases to nearly one-third of the total length.12 The longitudinal growth of the spine is due to cartilage proliferation in the upper and lower zones of the primary ossification centers of the vertebral bodies, and no growth
is seen in the annular cartilages.14 The growth of each vertebra and of the entire spine is modified by the stress of weight bearing. At birth, the average total spine length, not including the sacrum, is 20 cm; at 2 years of age, 45 cm; at puberty, 50 cm; and by adulthood, 75 cm.10
is seen in the annular cartilages.14 The growth of each vertebra and of the entire spine is modified by the stress of weight bearing. At birth, the average total spine length, not including the sacrum, is 20 cm; at 2 years of age, 45 cm; at puberty, 50 cm; and by adulthood, 75 cm.10
ANATOMIC VARIANTS
Anatomic variants of the vertebral column, that can be frequently encountered in the pediatric population, are discussed in this section.
The lateral masses of C1 may be laterally displaced from the odontoid process by as much as 6 mm, which is known as the “pseudo-Jefferson fracture” appearance (Fig. 5.14).
Pseudosubluxation is physiologic anterolisthesis of C2 on C3, and C3 on C4 to a lesser extent. It may resemble a true injury in severe cases. Radiographic evaluation with neutral, flexion, and extension views of the spine with a line from the anterior aspect of the posterior arch of C1 to the anterior aspect of the spinous process of C3 is helpful. The anterior margin of the posterior arch of C1 and the anterior margins of the C2 and C3 spinous processes should align within 1 mm of each other on the flexion and extension view cervical spine radiographs. If a line drawn along the posterior margin of the odontoid intersects or touches the upper posterior corner of C3, no dislocation is present15 (Fig. 5.15).
The variability in the C1-C2 interspinous distance in the younger child is related to ligamentous laxity, which allows spinal hypermobility. However, this distance variability may also be seen in older children. Distances of up to 10 mm or even 12 mm may be normal15 (Fig. 5.16).
Anterior vertebral wedging may be seen as the vertebral bodies evolve from oval to rectangular shape. It is most evident at C3. The etiology of this wedging may be related to spine hypermobility15 (Fig. 5.17).
Normal ossification centers, such as the os terminalis of the odontoid, which may be seen normally until 8 years of
age, vertebral ring apophyses, and lack of fusion of the arch of C1 may be confused with fractures15 (Fig. 5.18).
age, vertebral ring apophyses, and lack of fusion of the arch of C1 may be confused with fractures15 (Fig. 5.18).
![]() FIGURE 5.17 Vertebral wedging. Lateral radiograph in an infant girl shows multiple level anterior vertebral wedging. Vertebral wedging is most prominent at the C3 level. |
Limbus vertebra is a nontraumatic intraosseous herniation of the nucleus pulposus (Schmorl node), causing fragmentation and corner triangular endplate separation16 (Fig. 5.19).
CONGENITAL AND DEVELOPMENTAL ANOMALIES
Absence or Hypoplasia of the Odontoid
Absence or hypoplasia of the odontoid is a rare abnormality with associated underdevelopment of the apical and alar ligaments, which leads to cervical hypermobility17 (Figs. 5.21 and 5.22).
Os Odontoideum
Os odontoideum is an accessory rounded bony center at the upper dens. The etiology is not clear and may be congenital due to lack of fusion between this center (which originates from the proatlas) and the axis (C2). There is also a traumatic theory about the origin of the os odontoideum, in which interference of the blood supply to the fractured apical segment is implicated. The alar ligament displaces the segment cranially. The os odontoideum should not be confused with the normal ossification center, os terminalis, at the tip of the odontoid, which appears at age 3 and fuses with the dens by age 1218 (Fig. 5.23).
Vertebral Chondrification or Ossification Developmental Anomalies
These are multiple defects caused by alteration of the normal development of the vertebral cartilage and ossification centers. These defects may be seen in the body or posterior elements of the vertebra10 (Fig. 5.24).
Anomalies Involving Vertebral Body
Asomia (Agenesis)
Asomia (agenesis) is an uncommon anomaly, which is believed to represent a developmental failure of the vertebral body cartilaginous centers, secondary to vascular insufficiency. Asomia may be a simple abnormality or part of a generalized spinal dysraphism. The posterior elements often remain present.19
Hemivertebra
Classical hemivertebra is a relatively common defect where there is absence of half of the vertebral body and neural arch due to developmental failure of the corresponding cartilaginous centers. It is currently believed to be related to ischemic changes. The classical lateral hemivertebrae assume a wedge-shaped configuration with the apex toward the midline. Hemivertebrae may be single or multiple. They may represent an isolated anomaly or present as part of a complex congenital spinal abnormality. Ventral or dorsal hemivertebrae may also occur as a consequence of failure of formation of the right and left cartilaginous centers, respectively.19,20
Sagittal Cleft (Butterfly Vertebra)
Sagittal cleft (butterfly vertebra) is due to the lack of fusion of the two halves of the vertebral body, resulting in two hemivertebrae separated by a sagittal cleft. It is believed to be related to an adhesion between the ectoderm and endoderm that forces the notochord to divide. Sagittal cleft of the vertebral body is relatively common and is usually seen in the thoracic and lumbar spine. It may be associated with abnormality of the intervertebral disc, anterior spina bifida with or without meningocele, diastematomyelia, VACTERL association, and other conditions19,20,21 (Fig. 5.25).
Coronal Cleft
Coronal cleft originates from lack of fusion of the ventral and dorsal ossification centers, separated by a cartilaginous cleft. It usually presents in males in the newborn period and is rarely seen after 5 years of age. Coronal cleft may be isolated with no clinical importance or associated with other anomalies such as chondrodysplasia punctata.19,20,21,22
Anomalies Involving Posterior Elements of the Vertebra
Malformations of the posterior elements result from abnormal development of the neural arch ossification centers and range from mild (spina bifida occulta) to severe (complete absence)18,19,21 (Fig. 5.26).
Spina Bifida Occulta
Spina bifida occulta is the most common neural arch anomaly, consisting of lack of fusion of the posterior neural arches. The defect may be seen at a single level. The most frequent levels, in descending order, are L5, S1, C1, C7, T1, and the lower thoracic region. Spina bifida occulta is most frequently seen as a solitary defect; however, it may also accompany more complex malformations.4,20
![]() FIGURE 5.21 Odontoid anomalies. 3D-reconstructed images of C2 simulate abnormalities of the odontoid process. A: Os odontoideum. B: Hypoplasia of the odontoid. C: Aplasia of the odontoid. |
Spinal Dysraphism
Spinal dysraphism comprises a group of congenital anomalies of the central nervous system, in which there is maldevelopment of the cutaneous ectoderm (skin), neuroectoderm (neural tube), and bony mesodermal structures. It may involve multiple and consecutive vertebral segments and be associated with other mesenchymal and neural abnormalities. The neural-tissue containing defect may lack skin covering (open dysraphism), with or without protrusion of subarachnoid fluid (myelomeningocele or myelocele, respectively), or be covered by skin and subcutaneous tissue (closed dysraphism), with or without protrusion of the subarachnoid fluid (lipomyelomeningocele or lipomyelocele, respectively). More uncommonly, the externalized meningeal sac contains only subarachnoid fluid without neural elements (e.g., meningocele)1,20,23 (Fig. 5.27).
SEGMENTATION ABNORMALITIES
Complete “Block Vertebra”
This congenital abnormality is secondary to failure in the process of segmentation during fetal life that causes two or more adjacent vertebrae to be partially or completely fused in a single bony block. One or more vertebra(e) may be involved, without or with a rudimentary intervertebral disc. In order of frequency, block vertebra is most common in the lumbar, cervical, and thoracic regions. The morphologic anomalies of the block, such as concave configuration and narrow AP diameter, are due to growth deficiency at the fusion site. There may be associated disorders of embryogenesis in the levels above and below the “block vertebra,” such as hemivertebrae or absent vertebra.
Klippel-Feil Syndrome
Klippel-Feil syndrome is a congenital segmentation abnormality, characterized by fusion of two or more cervical vertebrae. The majority of affected pediatric patients have a short neck; limited motion, especially rotation; and a low hairline. There are three types described. In type I, there is extensive fusion of the cervical and upper thoracic vertebrae, usually associated with other anomalies. Type II demonstrates focal
vertebral fusion of one or two interspaces, usually at the C2-C3 or C5-C6 levels. Type III is associated with thoracic and lumbar spinal anomalies in addition to the findings of type I or type II Klippel-Feil syndrome.
vertebral fusion of one or two interspaces, usually at the C2-C3 or C5-C6 levels. Type III is associated with thoracic and lumbar spinal anomalies in addition to the findings of type I or type II Klippel-Feil syndrome.
Klippel-Feil syndrome is associated with other bony developmental abnormalities such as vertebral ossification malformations, basilar invagination, and Sprengel deformity. Neural and bony anomalies, in particular Chiari I malformation and diastematomyelia, may occur. Associated renal malformations include renal agenesis, hydronephrosis, horseshoe kidney, duplicated collecting system, renal ectopia, and bilateral tubular ectasia. Congenital heart disease (coarctation of the aorta, atrial septal defect), conductive hearing loss, and rib fusion may also be associated4,25,26,27 (Fig. 5.29).
Sprengel Deformity
This congenital abnormality occurs secondary to failure of scapular caudal migration during fetal development because of a fibrous osseous connection. The omovertebral bone articulates with an upper transverse process of the cervical spine. Sprengel deformity is associated with Klippel-Feil syndrome, spina bifida, torticollis, kyphoscoliosis, and atrophy of the regional muscles. The affected scapula is elevated with the inferior angle rotated laterally. Rigault classifies the abnormality in three grades: grade I, superomedial angle lower than the T2 but above the T4 transverse process; grade II, superomedial angle between the C5 and T2 transverse processes; and grade III, superomedial angle above the C5 transverse process4,20,28 (Fig. 5.30).
Caudal Regression Syndrome
Caudal regression syndrome is a congenital disorder in which the lower spine and/or sacrum is malformed. In addition to vertebral and/or sacral bone, other local tissues derived from the embryonic caudal cell mass may be affected. The caudal cell mass is a conglomerate of totipotential cells responsible for formation of the filum terminale, ventriculus terminalis, conus medullaris, distal lumbosacral vertebra, and urorectal septum, which unites with the cloaca to form the urogenital sinus and the anorectal channel.
Approximately 20% of infants with caudal regression syndrome are born to a mother with diabetes mellitus. There is also an association with OEIS (omphalocele, cloacal exstrophy, imperforate anus, and spinal deformities) complex. VACTERL syndrome (vertebral anomalies, anorectal malformations, cardiac anomalies, tracheoesophageal fistula, renal and limb anomalies) is seen in about 10% of affected patients.
Pang’s classification of lumbosacral dysgenesis includes:
Type I: Sacral and partial lumbar agenesis
Type II: Complete sacral agenesis
Type III: Partial sacral agenesis, distal to S2 (most common)
Type IV: Hemisacrum (second most common)
Type V: Coccygeal agenesis
The MRI findings of caudal regression syndrome include missing distal vertebrae and a “wedge-shaped” configuration of the distal cord when it is located above L1. When the spinal cord ends below L1, there may be other associated findings such as tethered cord, diastematomyelia, fatty or thickened filum, hydromyelia, and mild sacral hypogenesis. Associated abnormalities of the genitourinary and gastrointestinal systems are common23,29 (Fig. 5.31).
Diastematomyelia
Diastematomyelia is a condition in which all or part of the spinal cord is split. This abnormality originates from aberrant division of the notochord during embryonic life. If the canal of Kovalevsky persists (communication of the yolk sac with the amniotic cavity), or if there is any adhesion between the ectoderm and endoderm, an obstacle to the notochord cells is present. The notochord either deviates to one side or splits to pass around both sides of the obstacle.
The two hemicords may be symmetric or asymmetric, and the length of separation varies. Two types have been described. In type I (40% to 70%), there are two individual sacs separated by an osseous or cartilaginous septum, each containing a hemicord. In type II, there is a single dural sac containing both hemicords, which may be tethered by a nerve root or fibrous bands.
Diastematomyelia is associated with low position of the conus medullaris, thickened filum terminale, segmentation and ossification abnormalities, scoliosis, Sprengel deformity, Klippel-Feil syndrome, Chiari malformation, dermal sinus, lipomyelomeningocele, open dysraphism involving
one hemicord (hemimyelocele or hemimyelomeningocele), meningocele manqué, teratoma, and horseshoe kidney23,24,30,31 (Fig. 5.32).
one hemicord (hemimyelocele or hemimyelomeningocele), meningocele manqué, teratoma, and horseshoe kidney23,24,30,31 (Fig. 5.32).
Currarino Triad
Currarino triad is a rare syndrome first described by Kennedy in 1926 and by Currarino in 1981. It is characterized by anorectal malformation (anal stenosis, anal ectopia, imperforate anus), sacrococcygeal osseous defect (scimitar, comma, or crescent-shaped), and presacral mass, most commonly teratoma or meningocele. In 50% of affected patients, this syndrome is autosomal dominant32,33 (Fig. 5.33). Mutations in the gene MNX1 have been reported in almost all familial and many sporadic cases of Currarino triad.
Sacrococcygeal Teratoma
Sacrococcygeal teratomas are rare congenital neoplasms presenting in ˜1 in 40,000 infants, more commonly in females. They derive from pluripotential cells, are thought to originate from Hensen node, and contain components arising from the three germinal layers. Sacrococcygeal teratomas are classified according to their position in the pelvis. Type I is almost completely external with a minimal portion in the presacral region (47%). Type II is both external and presacral in location (35%). Type III is mainly located in the pelvis and abdomen (8%). Type IV is a presacral mass (10%).23,32,34
The majority of sacrococcygeal teratomas are mixed solid and cystic lesions, with only 5% presenting as a cystic neoplasm. Calcifications may be seen in up to 50% of cases. When associated with sacrococcygeal defects, anorectal stenosis, cutaneous stigmata, and genitourinary anomalies, a familial form with dominant inheritance is suggested23,32,34 (Fig. 5.34).
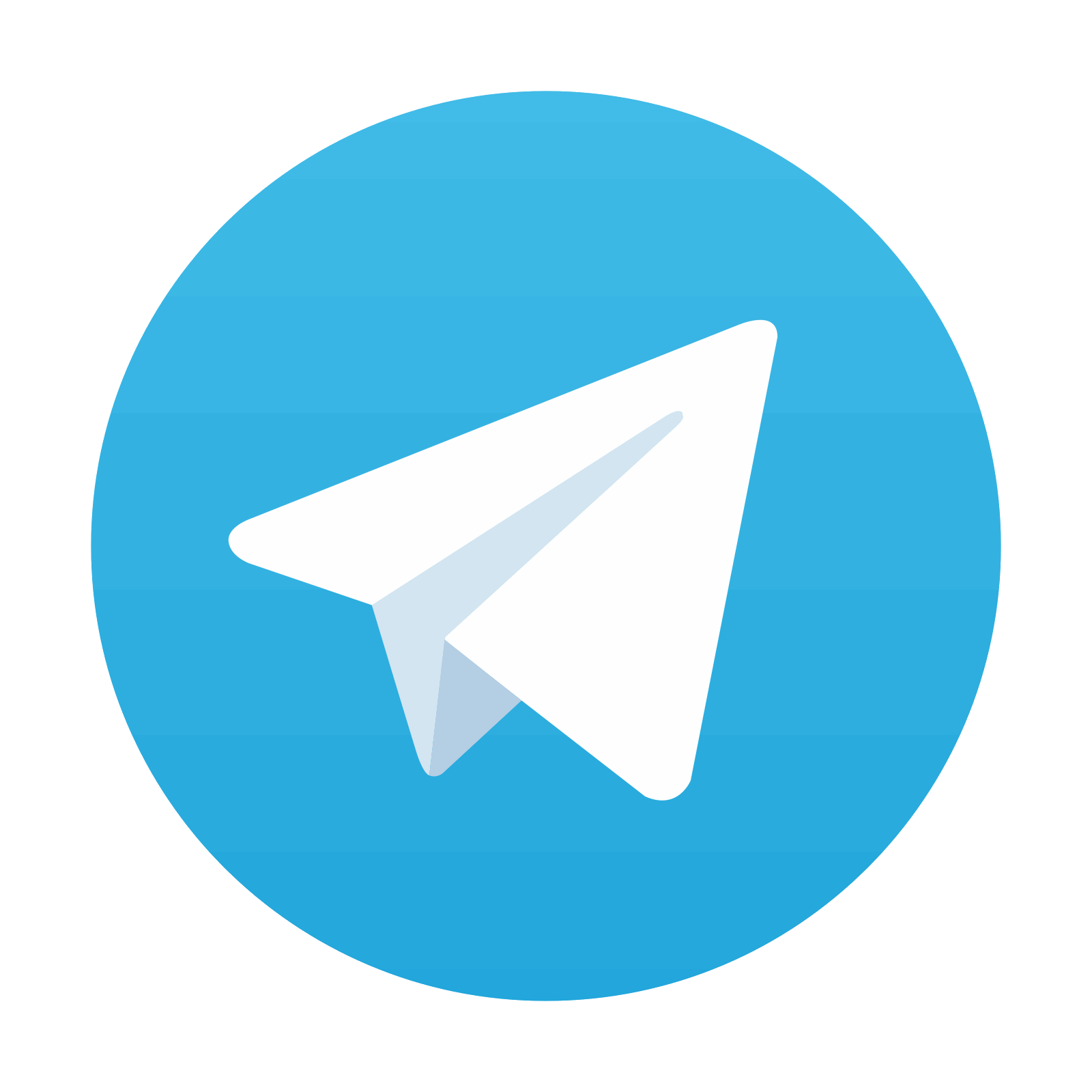
Stay updated, free articles. Join our Telegram channel

Full access? Get Clinical Tree
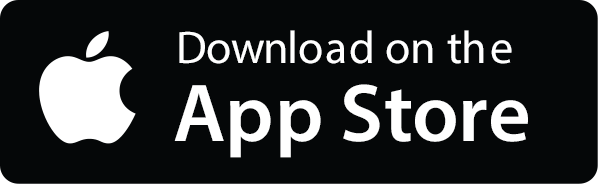
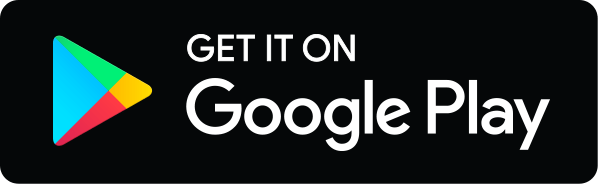