Fig. 46.1
Sequence of phases in whiplash injury after collision. a 0–50 ms: After the impact there is no motion or body response. b 50–100 ms: The torso, pushed by the seat, is pushed forward and upward; meanwhile, the head remains stationary due to the inertial force. The ascending movement of the trunk compresses the cervical spine, which, around 60 ms after the impact, undergoes an “S-shape” deformation, with the lower cervical segments exceeding physiological extension and the upper cervical segments flexion. c 100–200 ms: The head reaches a peak movement in extension of 45°. d 200–400 ms: Head, neck, and trunk start to descend, and about 400 ms after the initial impact, the head achieves its maximum forward displacement
Whether or not the driver in rear-end crashed car is aware of the impeding collision is extremely important (Fig. 46.2). According to Mertz and Patrick (1967) and Stemper et al. (2005, 2006), aware subjects obtain maximum neck muscle contraction before the impact leading to elimination of the S-shape curvature and decrease in the extension magnitude and soft tissue distortion. In unaware subjects, the muscular reflex contraction has minimal effect because the maximum contraction levels are achieved after injury time (S-shape) (Kumar et al. 2005). The awareness or expectancy of the incoming collision is crucial in the whiplash process; despite this fact, it was rejected as predictive factor (Walton et al. 2009). It is known that 70–80 % of the patients suffering from WAD were unaware of the incoming collision (Radanov et al. 1995; Hartling et al. 2002; Hendriks et al. 2005; Atherton et al. 2006). Moreover, when pain is not the only outcome, it is found that a correlation exists between being unaware of the incoming collision and a poor recovery 12 weeks after injury (odds ratio, 3.803; 95 % CI, 1.197; 12.083) (Hendriks et al. 2005).
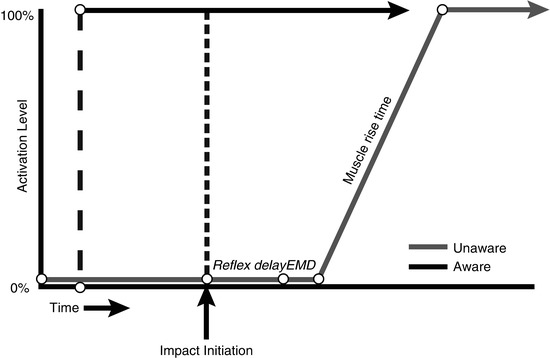
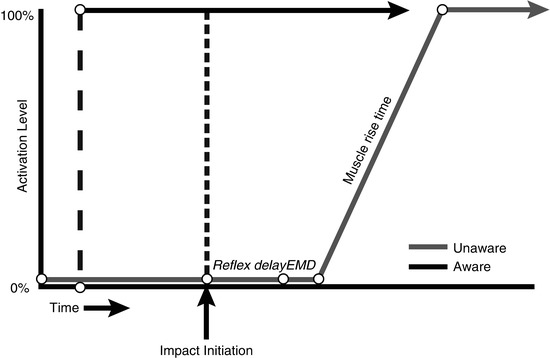
Fig. 46.2
Timing of neck muscle contraction in aware and unaware occupants. Reflex delay: time between the stimulus and initiation of electrical muscle activity. Electromechanical delay (EMD): the time between initiation of electrical muscle activity and mechanical force generation (Adapted from Stemper et al. (2006)). With kind permission from Dr. Brian D. Stemper, Department of Neurosurgery, Medical College of Wisconsin, USA
46.2.1 Pathological Considerations
Although current diagnostic techniques cannot identify injuries in WAD patients, tissue damage was observed during surgery of joint capsules (Johansson 2006) and at autopsy in the cervical intervertebral disks, articular surfaces, and capsules of the facet joints (Uhrenholt et al. 2002). The existence of tissue damage is also supported by increased concentration of neurofilament protein in the cerebrospinal fluid (Guéz et al. 2003).
46.2.2 Facet Joint and Capsular Ligament
Two nonexclusive mechanisms of injury have been proposed. First, damage of the cartilage and pinching of the synovia, with special relevance to the lower cervical vertebral column, where the peak of extension takes place during the S-shape phase. The second mechanism can be an excessive strain of the capsule.
46.2.3 Ligaments and Intervertebral Disk
Among the multiple ligaments surrounding the cervical vertebras, the anterior longitudinal ligaments and the anterior annulus fibrosus are at higher risk in rear-end collisions, while strains of supraspinous ligaments, interspinous ligaments, and ligamenta flava are more frequently damaged in frontal impacts. The intervertebral disk injuries occur more frequently in rear-end collisions, with the C5/C6 disk at the highest risk (Ivancic et al. 2004, 2005; Ito et al. 2005). However, these injuries to ligaments obtained in theoretical and cadaveric models are not consistently demonstrated in whiplash patients (Myran et al. 2011) despite initial positive reports using magnetic resonance imaging (MRI) (Krakenes and Kaale 2006).
46.2.4 Dorsal Root Ganglion
Cadaver and animal models suggest that rapid neck motion causes a trauma of the nerve roots and ganglions in the neck, because of foraminal narrowing or transient pressure gradients in blood flow. However, such a relationship has not yet been demonstrated in whiplash patients.
46.2.5 Neck Muscles
Clinical evidence supports the role of neck muscles in WAD. Microdialysis of the trapezius muscle demonstrated increased serotonin, IL-6, and kallidin levels, all involved in inflammatory processes and hyperalgesia (Gerdle et al. 2008a, b). Serum levels of C-reactive protein were also found elevated in WAD patients, and these values remained elevated after 3 months in those patients classified as moderate or severe disability using the Neck Disability Index, while no differences were found in serum IL-1β (Sterling et al. 2013). In the same study, a moderate negative correlation was found between TNF-α serum values and the amount of fatty muscle infiltrate and pain intensity at 3 months in WAD patients. This fatty infiltration in the muscle, considered indicative of pseudohypertrophy, has been extensively demonstrated in chronic stages of WAD by MRI studies (Elliott et al. 2006, 2008, 2010).
46.3 Imaging Studies
With current diagnostic tools, most of the above-mentioned possible sites of injuries are difficult to demonstrate. Standard imaging techniques such as radiography, computed tomography (CT), and MRI are inconclusive for the prognosis of the symptoms after a whiplash trauma (Williams et al. 2007). Besides the use of imaging techniques for clinical diagnosis, they have also been used to perform basic research to clarify the mechanisms underlying whiplash injury.
46.3.1 Neck Imaging
Linnman et al. (2011) visualized the neck region using positron emission tomography (PET), with the inflammatory marker 11C-D-deprenyl. Whiplash patients with persistent pain were compared to healthy volunteers and to another control group suffering from pain caused by ankle sprain. Elevated tracer uptake was found in WAD patients, especially around the spinous process of the second cervical vertebra, indicative of a persistent peripheral tissue inflammation (Fig. 46.3).
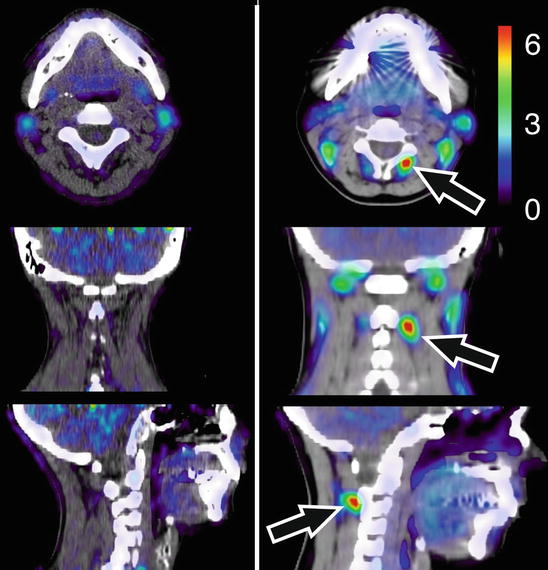
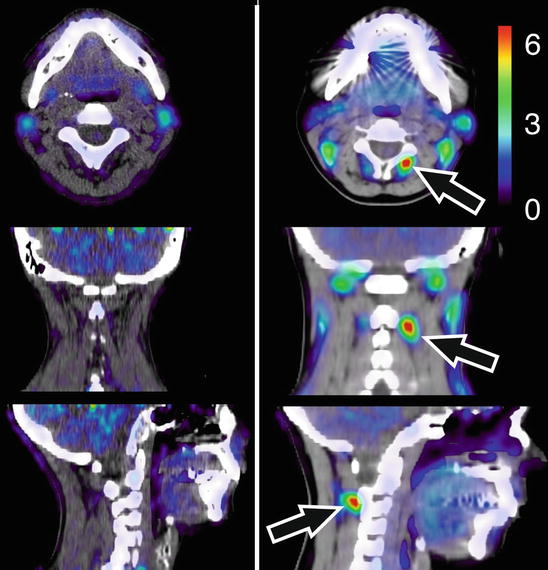
Fig. 46.3
11C-D-deprenyl (DDE) uptake in a representative healthy control (left) and a patient with whiplash-associated disorder (right). DDE uptake is expressed as standardized uptake value (SUV, see color bar in figure). A high DDE uptake can be seen in the patient’s adipose tissue on the right side of the spinous process of the second cervical vertebra, indicated by arrows (Adapted from Linnman et al. (2011)). With kind permission from Dr. Clas Linnman, P.A.I.N. Group, Boston Children’s Hospital, Harvard Medical School, USA; open-access license from PlosOne, www.plosone.org
46.3.2 Brain Imaging: Perfusion and Metabolism
Otte et al. (1995, 1997b) performed a series of functional neuroimaging studies (PET and single-photon emission computed tomography [SPECT]) in over 500 whiplash patients at rest using different tracers (99mTc-labelled hexamethyl propylene amine oxime [HMPAO], 99mTc-labelled ethylene biyldicysteinate dimer [ECD], and 18F-labelled fluorodeoxyglucose [FDG]). In many of these patients, compared to healthy controls, hypoperfusion and hypometabolism in the posterior parietal occipital cortex were observed, unilaterally or bilaterally (Fig. 46.4). Similar findings were recently obtained by Linnman et al. (2009) with PET scanning using H2 15O. Compared to healthy volunteers, they also found during rest an elevated regional cerebral blood flow (rCBF) bilaterally in the posterior parahippocampal, posterior cingulate gyri, right medial prefrontal gyrus, and right thalamus.
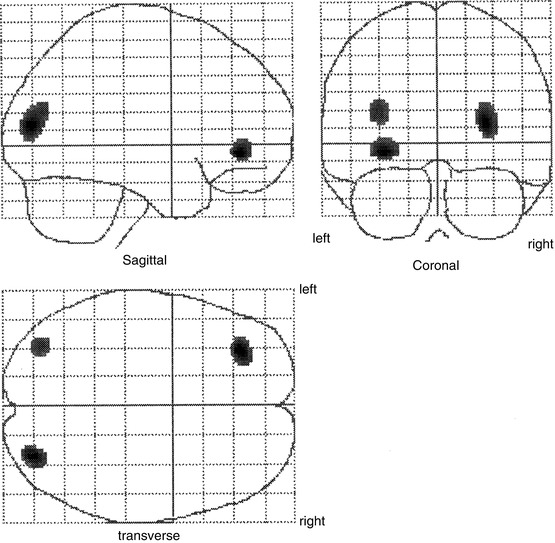
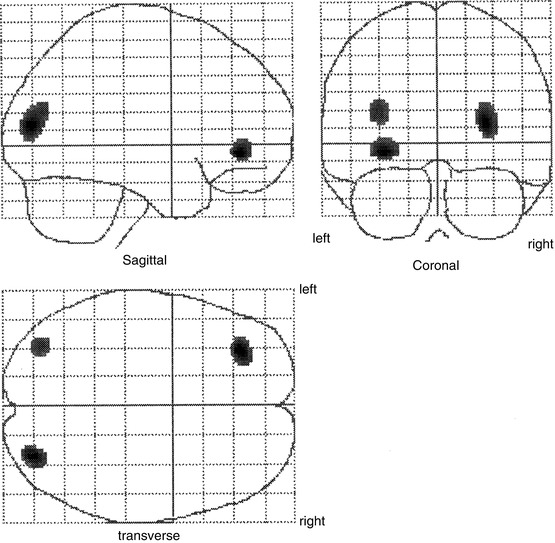
Fig. 46.4
Statistical parametric map projections; brain areas with significant decreased relative perfusion (p < 0.01) of 15 whiplash patients compared to 15 healthy controls. Statistically significant differences are displayed on sagittal, coronal, and transaxial projection of the brain: 99mTc-ECD SPECT. Note the hypoperfusion in the posterior parietal occipital region at both sides and in the frontal region on the left side. All patients remember that they looked to the right during the impact. The hypoperfusion frontal left and posterior parietal occipital right may be explained by a traumatic coup-contercoup mechanism, whereas the additional hypoperfusion in the posterior parietal occipital region on the left side can only be explained by the additional whiplash injury. Originally published in 1998 (Adapted from Otte et al. (2004))
Other studies support these results. Lorberboym et al. (2002) investigated whether there is a correlation between cerebral perfusion and P300 (an electrophysiological marker of cognitive ability). In WAD patients, a diminished perfusion of the temporal lobes was found, which correlates with abnormal P300 results. They concluded that the combination of these techniques may be useful in identifying subgroups of whiplash patients. Sundström et al. (2006), in a HMPAO SPECT study, did not find changes in the rCBF of whiplash patients compared with healthy controls, although they found two nonsignificant small regions with lower rCBF, one in the right temporal region and the other in the left temporoparietal region, detected at an uncorrected voxel level of p = 0.001. These findings are consistent with those reported by Otte et al., who are of the opinion that the different results between experiments may be due to the lower sensitivity of the HMPAO tracer compared with ECD in terms of retrieving small functional lesions (Otte et al. 1997a).
46.3.3 Other Brain Imaging Studies
An fMRI study of Freitag et al. (2001) found decreased activation during a coherent motion task of cortical areas V5 and V5a (middle temporal and middle superior temporal) in patients with WAD compared with asymptomatic patients after whiplash trauma and with healthy volunteers. These results are in accordance with previously mentioned results of hypoperfusion in the posterior parietal occipital region.
In a different research line, the availability of neurokinin 1 (NK1) receptors in WAD patients was studied, using the NK1-specific antagonist radioligand [11C]GR205171 (Linnman et al. 2010). This receptor is widely distributed throughout the brain and is the principal receptor for the neuropeptide substance P, which plays a role in level-setting systems and modulates pain perception, both peripherally and centrally. In their study, attenuated NK1 availability was found in WAD patients in frontal, insular, and cingulate cortex, hippocampus, amygdala, periaqueductal gray, and especially pronounced in the ventromedial prefrontal cortex, where NK1 attenuation correlates with behavioral measurements of fear and avoidance of movement. These results were recently revised showing an additional significant NK1 reduction in WAD patients in the left middle temporal gyrus and the right superior temporal gyrus (Linnman 2012) and reaffirming the importance of the posterior parietal occipital regions in the late whiplash syndrome (Otte 2012).
Two other MRI studies focused on brain’s morphology. No significant differences were found in ventricle-brain ratios between WAD patients and healthy volunteers (Sturzenegger et al. 2008), while adaptive gray matter changes in pain-processing structures were shown during the first year after the accident in WAD patients with headache (Obermann et al. 2009). In this last study, a decrease in gray matter of the anterior cingulate and dorsolateral prefrontal cortex was found 3 months after the trauma. These alterations resolved in parallel to the cessation of headache, 1 year after the injury. On the other hand, WAD patients who developed chronic headache showed an increase of gray matter in the midbrain (attributed to the periaqueductal gray), thalamus, and cerebellum 1 year after the accident.
46.4 Close Interaction Between the Neck and Midbrain
There is a close interaction between the neck and several midbrain structures, which involve the visual, vestibular, and somatosensory systems. This close interaction has been already proposed to be responsible for the sensorimotor disturbances in WAD patients (Treleaven 2008). However, the impact of the strong neuronal connection between neck afferents and the periaqueductal gray (PAG) and the disturbance of this system in WAD patients has never been considered thus far.
Interneurons in the intermediate zone of the upper cervical segments receive proprioceptive information from mechanoreceptors in facet joints, ligaments, and neck muscle spindles. This information is crucial for postural stability of the body and head as well as for determining the visual field (e.g., cervico-ocular, optokinetic, or vestibulospinal reflexes). The importance of this information is reflected in the high density of spindles in neck muscles (Liu et al. 2003).
The axons of these interneurons not only terminate on motoneurons of the ventral horn but also send ascending projections to different midbrain structures. Projections to the PAG originate from all parts of the spinal cord, but remarkably, more than 30 % of all the spino-PAG fibers originate from the C1–C3 spinal segments (Mouton et al. 2001; Klop et al. 2004). However, the function of these spino-PAG projecting neurons has not been studied. These neurons are distributed throughout lamina VI–VIII, with a specific cluster of neurons located laterally in the ventrolateral horn (Mouton et al. 2009). This cluster does not respond to natural stimuli, i.e., touch, pressure, pinch, cooling, noxious stimuli, joint movements, or deep muscle stimuli (Carstens and Trevino 1978; Yezierski and Broton 1991; Keay et al. 2001). And there is no information about the function of the other neurons in the rest of C1–C3 gray matter.
Also, the trigeminocervical complex maintains projections to the ventrolateral PAG (Akerman et al. 2011). This complex, constituted by the trigeminal nucleus caudalis and C1–C2 dorsal horn, is known to be involved in the development of migraine and headaches, such as those present in WAD. All these ascending pathways converge in a group of neurons located between the deep layers of the superior colliculus and the ventrolateral PAG (Fig. 46.5).
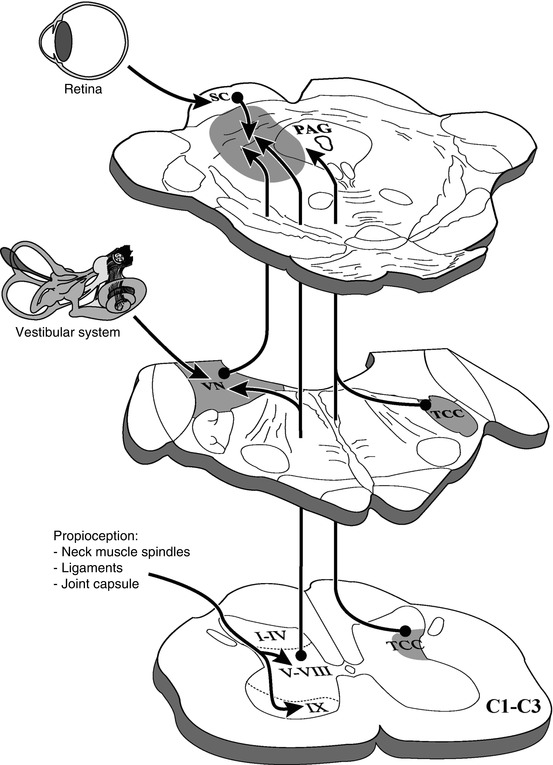
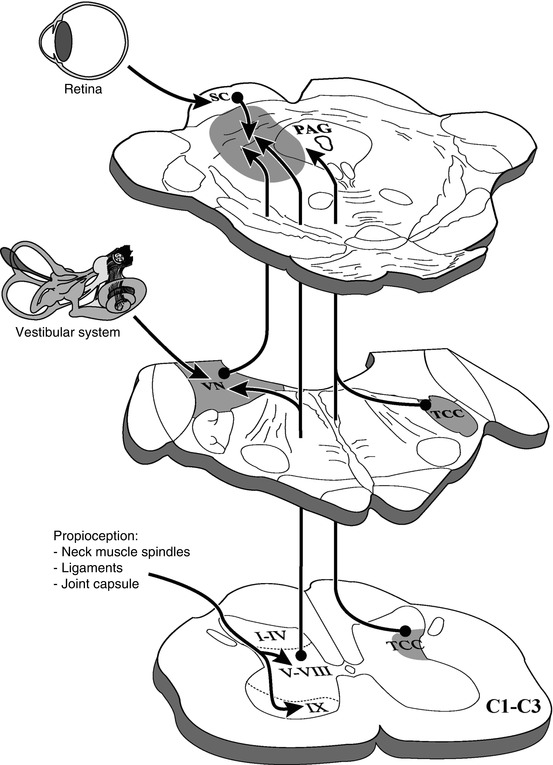
Fig. 46.5
Ascending afferents from the upper cervical segments (C1–C3) to the periaqueductal gray and its adjoining regions. TCC trigeminocervical complex, VN vestibular nuclei, PAG periaqueductal gray, SC superior colliculus
The PAG and its adjoining regions are involved in several basic functions, directly related to survival of the individual and survival of the species. Examples are pain modulation (Tracey and Mantyh 2007), micturition (Holstege 2005), blood pressure (Carrive et al. 1988), fight-flight response (Bandler and Shipley 1994), vocalization (Holstege 1989), respiration (Subramanian et al. 2008), and mating behavior (Holstege and Huynh 2011). In whiplash patients, the PAG and adjoining regions are shown to be involved in this disorder: lower NK1 receptor availability compared to healthy controls (Linnman et al. 2010) and gray matter changes in patients who develop chronic headache after a whiplash injury (Obermann et al. 2009).
The involvement of the PAG and adjoining areas in the pathophysiology of WAD can explain the alterations in pain perception, but also depressive-like symptoms (Northoff et al. 2011). The relation of the trigeminocervical complex and the PAG can explain the headaches and temporomandibular pain symptoms in many WAD patients. Also, many other WAD symptoms, like dizziness, visual disturbances, and alterations in postural control, can be explained by processing aberrant information from the neck muscles and other damaged neck parts in the PAG and adjoining regions.
46.5 Discussion and Conclusions
The lack of tools for clinicians to reach a diagnosis and to predict the outcome is a major problem in the acute phase of whiplash. Female gender, strong initial symptoms, history of previous neck pain, passive coping style, depressive mood, fear of movement, catastrophizing, and/or being involved in lawsuits are just a few predictive factors for chronicity (Carroll et al. 2008; Walton et al. 2009). From these factors, special interest falls on litigation. There is a discussion in the scientific community about the relevance of malingering, compensation, and adoption of a sick role, especially after a study reflecting that the number of claims decreased by about 25 % in Saskatchewan (Canada) when they changed to a no-fault insurance system without payment for pain and suffering (Cassidy et al. 2000). The discussion was supported also with the results of some experiments in Lithuania, where little awareness exists among the population that chronic symptoms may be the result of low-velocity car collisions and where few car drivers and passengers are covered by insurance (Schrader et al. 1996). However, there is no clear evidence to support that compensation and its related processes are involved in the health of WAD patients (Spearing et al. 2012a, b).
There are two basic questions to be addressed: Which injury in the neck explains the initial symptoms? and Which factors are related to the chronicity of symptoms?
In the present chapter, scientific data have been presented that support an injury mechanism, but further research is needed to define the factors involved in chronicity. In rear-end collisions, the inertia of the body generates a movement of the neck leading to the so-called S-shape. Around 60 ms after the impact, the neck is not protected by muscles and is exposed to a movement of extension in lower cervical vertebras, above physiological range, with a simultaneous movement of flexion in upper cervical vertebras. This produces different kinds of injury, implicating joints, capsule, ligaments, nerves, and muscles. The involvement of the cervical joints in the whiplash symptoms and the evidence of a process of central hypersensitivity (Banic et al. 2004; Stone et al. 2013) highlight the importance of an underlying biological mechanism in this disorder (Curatolo et al. 2004).
A crucial factor in this process is the “expectancy of the incoming collision,” as described in previous sections. The lack of better structured experiments regarding the influence of the unexpected impact on the development of late symptoms and the fact that the S-shape is reduced in aware subjects (Stemper et al. 2006) encourage further studies on this aspect. Moreover, pre-contraction of neck muscles could explain the results of the “placebo” rear-end collision study by Castro el al. (2001). In their experiments, all subjects were aware of the incoming impact because they knew the purpose of the experiment and, more importantly, the fake collision was preceded by a brake sound, giving hearing cues to the volunteers that allow them to contract their neck muscles. This erased the condition of placebo of this experimental setup, not allowing any clear conclusion of the experiment.
How can an injury caused by a low-velocity accident trigger such a broad variety of symptoms? Some functional imaging studies of the brain and of the cervical soft tissue are inconclusive in terms of the mechanism of action, as they only show the reaction to the trauma (musculoskeletal inflammation or hypoperfusion of the posterior parietal occipital region) rather than the origin (Otte et al. 1995; Linnman et al. 2011). We postulate an injury-induced mismatch in the midbrain between aberrant information from the damaged cervical muscles and other structures via the upper cervical cord to the mesencephalon (Mouton et al. 2005) on the one hand and the intact information from the vestibular and visual systems to the mesencephalon on the other hand (Fig. 46.5). This imbalance causes the sensorimotor disturbances (postural stability, head and eye movement control) and other symptoms observed in whiplash patients. It seems that the brain always seeks to reestablish its physiological homeostasis after the initial whiplash injury. However, if this conflict remains, for example, due to persistent damage of cervical components, the mesencephalic brain structures processing this information cannot appropriately associate it with vestibular and visual information, causing the diversity of symptoms related to whiplash injury, such as nausea, dizziness, headache, neck pain, and many other unpleasant feelings. Although a multitude of factors, including psychological traits, influences the outcome, whiplash always starts with the injury caused during the S-shape phase, the time period in which soft tissue of the neck is exposed to injury. It usually involves a subject who was unprepared for the incoming collision and for that reason did not switch on the protective function of the involved muscles. The subsequent continuous imbalance may create a permanent hyperarousal of the brain, depending on the strength of the cervical damage.
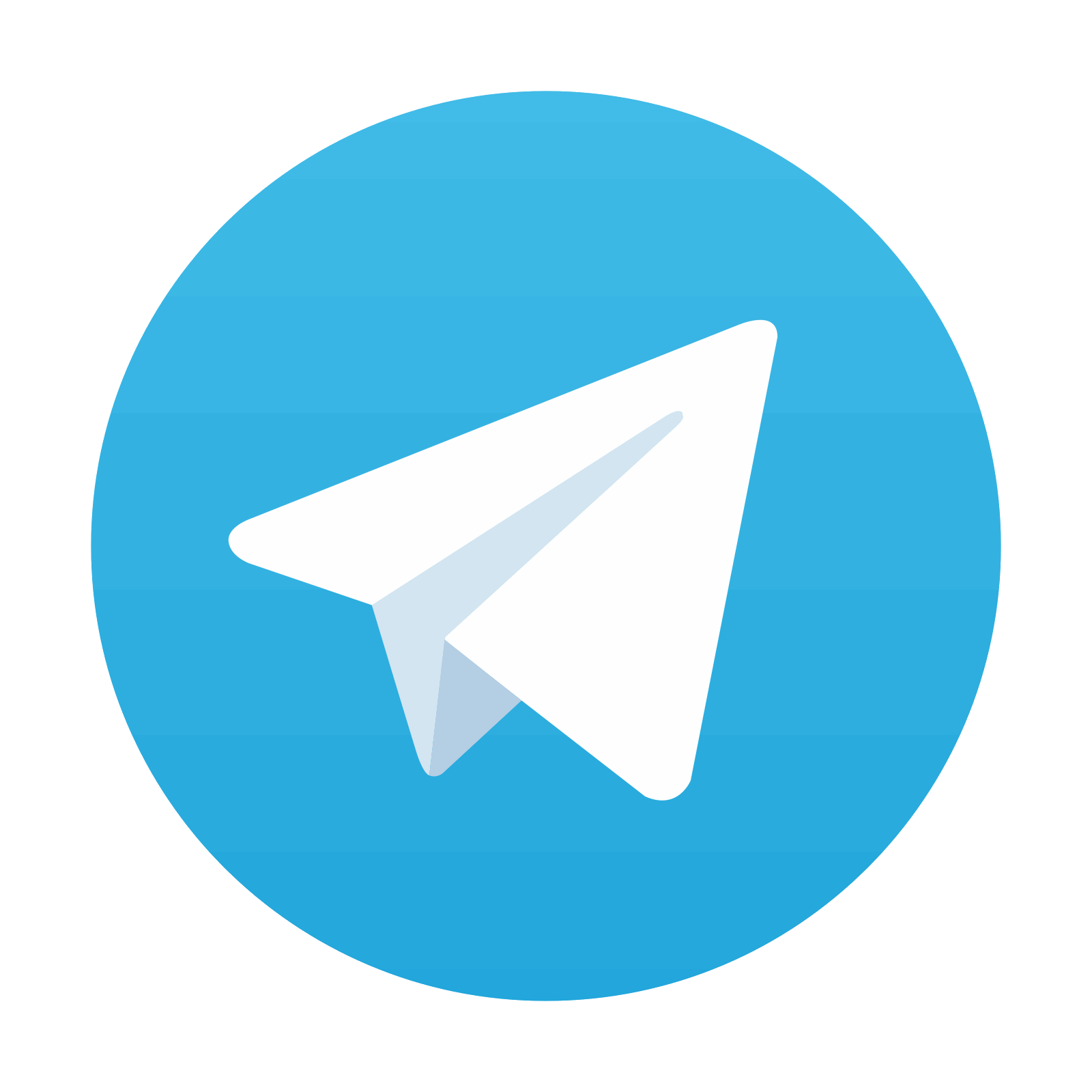
Stay updated, free articles. Join our Telegram channel

Full access? Get Clinical Tree
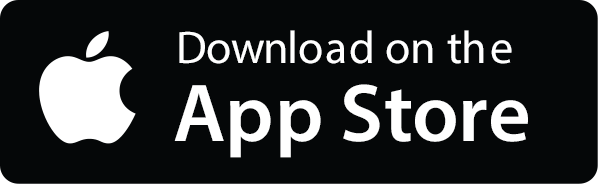
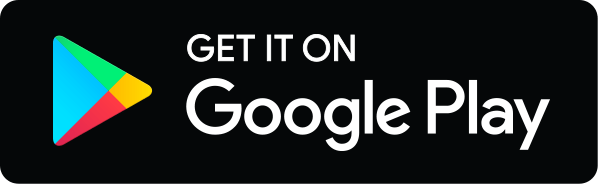