White Matter and Neurodegenerative Diseases
Jerome A. Barakos
Derk D. Purcell
In contrast to gray matter, which contains neuronal cell bodies, white matter is composed of the long processes of these neurons. The axonal processes are wrapped by myelin sheaths, and it is the lipid composition of these sheaths for which white matter is named. In this chapter, a host of diseases characterized by the involvement of white matter are described. This is followed by a discussion of hydrocephalus and neurodegenerative disorders.
The marked sensitivity of T2-weighted images (T2WI) allows white matter lesions to be readily detected, providing high sensitivity to lesion detection. However, the difficulty confronting the radiologist is that a wide gamut of diseases may involve the white matter, and thus white matter lesions are often nonspecific in nature, that is, low specificity. The specificity of lesion characterization arises when combining an understanding of various white matter diseases and their corresponding clinical features with lesion morphology and anatomic distribution. This combination of clinical information and imaging data is the cornerstone of what enables the radiologist to generate an accurate and meaningful differential diagnostic list.
Cerebral white matter diseases are classified into two broad categories: demyelinating and dysmyelinating. Demyelination is an acquired disorder that affects normal myelin. The vast majority of white matter diseases, especially in the adult, fall into this category and are the principal focus of this chapter. In contrast, dysmyelination is an inherited disorder affecting the formation or maintenance of myelin, and thus is typically encountered in the pediatric population. Dysmyelination is rare and is discussed later in this chapter.
Demyelinating Diseases
Demyelinating disease can be divided into four main categories on the basis of etiology: (1) primary, (2) ischemic, (3) infectious, and (4) toxic and metabolic (Table 7.1).
Primary Demyelination
Multiple sclerosis (MS) is the classic example of a primary demyelinating disease. MS is a disease characterized by immune dysfunction with the production of abnormal immunoglobulins and T cells, which are activated against myelin and mediate the damage associated with the disease. It is a chronic, relapsing, often disabling disease affecting more than a quarter of a million people in the United States alone. The age of onset is between 20 and 40 years, with only 10% of cases presenting in individuals older than 50 years. There is a female predominance of almost two to one. Although several environmental factors have been associated with MS, such as higher geographic latitudes and upper socioeconomic status, the etiology of MS remains unclear.
Establishing a diagnosis of MS is challenging, because no specific examination, laboratory test, or physical finding, taken in isolation, is unequivocally diagnostic or pathognomonic of this disorder. At the same time, diagnosing a patient with MS is portentous, as there are significant implications on many aspects of their life, including eligibility for health insurance. However, establishing the diagnosis is important because promising therapies are available, including β-interferon and antineoplastic drugs. These agents suppress the activity of the T cells, B cells, and macrophages that are thought to lead the attack on the myelin sheath.
The classic clinical definition of MS is multiple CNS lesions separated in both time and space. Patients may present with virtually any neurologic deficit, but they most commonly present with limb weakness, paresthesia, vertigo, and visual or urinary disturbances. Important characteristics of MS symptoms are their multiplicity and tendency to vary over time. The clinical course of MS is characterized by unpredictable relapses and remissions of symptoms. The diagnosis can be supported with clinical studies, which include visual, somatosensory, or motor-evoked potentials and analysis of CSF for oligoclonal banding, immunoglobulin G index, and presence of myelin basic protein. Histopathologically, active MS lesions represent areas of selective destruction of myelin sheaths and perivenular inflammation, with relative sparing of the underlying axons. These lesions may occur throughout the white matter of the CNS, including the spinal cord. The inflammatory demyelination interrupts nerve conduction and nerve function, producing the symptoms of MS. Note that histopathologically, the inflammation is a key differentiating feature between MS and other white matter conditions, such as osmotic myelinolysis (central pontine and extrapontine myelinolysis) and posterior reversible encephalopathy syndrome (PRES), which lack inflammatory changes. MR is the most sensitive indicator in the detection of MS plaques, but imaging findings alone should never be considered diagnostic.
In clinically confirmed cases of MS, MR typically demonstrates lesions in more than 90% of cases. This compares with far less than 50% for CT and 70% to 85% for laboratory tests such as brain stem–evoked potentials and CSF oligoclonal bands. Nevertheless, the ultimate diagnosis rests with the careful combination of clinical symptoms, history, and clinical testing, including MR imaging.
In clinically confirmed cases of MS, MR typically demonstrates lesions in more than 90% of cases. This compares with far less than 50% for CT and 70% to 85% for laboratory tests such as brain stem–evoked potentials and CSF oligoclonal bands. Nevertheless, the ultimate diagnosis rests with the careful combination of clinical symptoms, history, and clinical testing, including MR imaging.
Table 7.1 Classification of White Matter Diseases | |||||
---|---|---|---|---|---|
|
A variety of T2WI techniques have been described for optimizing the detection of white matter lesions, with fluid-attenuated inversion recovery (FLAIR) sequences leading the way. As the name suggests, FLAIR imaging has the advantage of providing heavy T2 weighting while suppressing signal from CSF. As such, FLAIR images provide improved lesion conspicuity of periventricular lesions, which may otherwise be obscured by the bright signal of CSF on FSE T2WIs. Comparative studies have demonstrated that FLAIR imaging provides the best visualization of supratentorial white matter lesions. However, the FLAIR sequence may have mild limitations when imaging the posterior fossa and spine, partly because of pulsation artifacts. In these anatomic regions, both proton density and short tau inversion recovery (STIR) imaging are valuable.
MS plaques are typically round or ovoid, with a periventricular or juxtacortical location (Fig. 7.1). Lesions are bright on T2WIs, reflecting active inflammation or chronic scarring, and only a fraction of MS plaques will demonstrate contrast enhancement. Enhancing lesions are indicative of acute lesions with active demyelination and disruption of the blood–brain barrier. In older lesions, without residual inflammatory reaction, abnormal high signal on T2WIs persists, reflecting residual scarring. Within the CNS, cells can mount only a limited response to neuronal injury. This scarring typically manifests as a focal proliferation of astroglia at the site of injury, termed “gliosis.” In severe cases of MS, actual loss of neuronal tissue may occur and the white matter lesions may actually have dark signal on T1WIs, often referred to as the “dark lesions” of MS. These lesions are prognostically significant because they reflect actual loss of underlying neuronal tissue rather than simple demyelination and are in keeping with a more advanced stage of this disease. Additionally, in chronic cases of MS, there is diffuse loss of deep cerebral white matter, with associated thinning of the corpus callosum and ex vacuo ventriculomegaly.
Although many white matter lesions are nonspecific in nature, the pattern suggestive of MS includes lesions that are periependymal, abutting the ependymal surface, as well as lesions involving the posterior fossa structures, other than for the central pons. The pons is excluded because most lesions in this location are either ischemic in nature or the result of osmotic demyelination, discussed later in this chapter. The periventricular lesions suggestive of MS are often ovoid and aligned perpendicular to the long axis of the ventricles. This pattern is the result of the alignment of the lesions along the perivenular spaces. Additional characteristic features include lesions along the callosal septal interface, as well as lesions that are confluent in nature and greater than 6 mm in diameter with a periventricular location.
In addition to the periventricular white matter, the cerebellar and cerebral peduncles as well as the corpus callosum, medulla, and spinal cord can be involved in MS. Ischemic changes are rare in these locations; as a result, if periventricular lesions are accompanied by lesions in any of these areas, this dramatically increases the specificity for the diagnosis of MS. The pons is excluded from this list of posterior fossa structures due to its proclivity for small vessel ischemic injury. In contrast, because ischemic changes rarely involve the medulla and cerebellar/cerebral peduncles, the presence of lesions in these areas is a useful differential diagnostic factor in suggesting MS. This is particularly important in patients older than 50 years, because it is difficult to decide whether multifocal white matter lesions are the result of ischemia or a demyelinating process. Additional concepts for making this distinction are discussed in the next subsection.
Although the periependymal lesions and posterior fossa location of white matter lesions describe above are certainly suggestive of MS, these findings are not diagnostic of MS as numerous other conditions outlined below, such as lupus, antiphospholipid syndrome, and other angiopathic conditions may be the cause. It is a disservice to both the patient and the referring clinician for the radiologist to constantly parrot a differential list, which includes MS in every patient who may have a few punctate white matter foci. With the quality of modern-day MR imaging and the exquisite sequences such as thin section 3D volume FLAIR, a significant number of all MR scans will reveal white matter lesions, even in the young, 0 to 40 years of age. In this age group, studies have revealed white matter lesions in over 50%. It is important to note that these ubiquitous lesions are often punctate measuring on the order of 1 to 2 mm, and quite different from the periependymal lesions of MS. In contrast to MS lesions, these hyperintense foci are typically located within the subcortical and deep white matter, often clustered in the frontal lobes and associated with perivascular spaces (Fig. 7.2). In fact these punctate foci may simply represent mild gliosis associated with the perivascular space. It should also be pointed out that these punctate foci of hyperintensity are not associated with traumatic etiology. Several poorly controlled studies in the early days of MR created a lore in this regard, which has since been disproven. In this regard, Chapter 3 highlights the characteristic imaging features of
diffuse axonal injury including microbleeds as hallmarks of traumatic pathology.
diffuse axonal injury including microbleeds as hallmarks of traumatic pathology.
MS lesions may also present as a large, conglomerate, deep white matter mass that can be mistaken for a neoplasm (Fig. 7.3). These lesions are referred to as tumefactive MS or tumefactive demyelinating lesions (TDL) and differentiation from malignancy may be challenging, with lesions not uncommonly making it to biopsy before the correct diagnosis is established. A useful imaging finding that often differentiates these conglomerate MS plaques from neoplasms is that they often demonstrate a peripheral crescentic rim of contrast enhancement, which represents the advancing leading edge of active demyelination. Detecting this pattern of enhancement and searching carefully for other more characteristic periventricular or posterior fossa lesions are essential clues in distinguishing TDL from neoplasm.
The spinal cord may also be involved with MS, and whenever a focal abnormality of the spinal cord is detected, a demyelinating MS plaque must be in the differential diagnosis. Demyelinating plaques may have mild mass effect as well as contrast enhancement, thus mimicking a neoplasm. The majority of spinal cord MS lesions (70% to 80%) will have associated plaques in the brain. In the setting of a cord lesion, performing an MR scan of the head may confirm the diagnosis, thus avoiding a spinal cord biopsy (see Chapter 10).
Ischemic Demyelination
Age-Related Demyelination. Small-vessel ischemic changes within the deep cerebral white matter are seen with such frequency in middle age (>50 years) that they are considered a normal part of aging. This represents an arteriosclerotic vasculopathy of the penetrating cerebral arteries. The deep white matter is more susceptible to ischemic injury than gray
matter, because it is supplied by long, small-caliber penetrating end arteries, without significant collateral supply. In contrast, cortical gray matter, as well as parts of the brain stem such as the midbrain and medulla, have robust collateral blood supply, thus minimizing the risk of ischemia. The deep penetrating vessels supplying the white matter become narrowed by arteriosclerosis and lipohyalin deposits. The result is the formation of small ischemic lesions, primarily involving the deep cerebral and periventricular white matter as well as the basal ganglia (Fig. 7.4). The cortex, subcortical “U” fibers, central corpus callosum, medulla, midbrain, and cerebellar peduncles are usually spared because of their dual blood supply, which decreases their vulnerability to hypoperfusion. As previously described, if lesions are identified in these locations, a cause other than ischemia should be entertained.
matter, because it is supplied by long, small-caliber penetrating end arteries, without significant collateral supply. In contrast, cortical gray matter, as well as parts of the brain stem such as the midbrain and medulla, have robust collateral blood supply, thus minimizing the risk of ischemia. The deep penetrating vessels supplying the white matter become narrowed by arteriosclerosis and lipohyalin deposits. The result is the formation of small ischemic lesions, primarily involving the deep cerebral and periventricular white matter as well as the basal ganglia (Fig. 7.4). The cortex, subcortical “U” fibers, central corpus callosum, medulla, midbrain, and cerebellar peduncles are usually spared because of their dual blood supply, which decreases their vulnerability to hypoperfusion. As previously described, if lesions are identified in these locations, a cause other than ischemia should be entertained.
Histologically, areas of infarction demonstrate axonal atrophy with diminished myelin. Early neuropathologists noted the areas of paleness associated with these changes and coined the term “myelin pallor.” These white matter changes have received many names over the years, including leukoaraiosis, microangiopathic leukoencephalopathy, and subcortical arteriosclerotic encephalopathy. None of these terms are very satisfying, as they do not accurately reflect all the changes observed histologically and overstate the clinical significance of these lesions. A more appropriate term may simply be “age-related white matter changes.” These small ischemic white matter lesions are often asymptomatic, and clinical correlation is always required before a diagnosis of subcortical arteriosclerotic encephalopathy or multi-infarct dementia (Binswanger disease) is made. The white matter infarcts just described differ from lacunar infarcts. Lacunae refer to small infarcts (5 to 10 mm) occurring within the basal ganglia, typically the upper two-thirds of the putamina. Both lacunar and deep white matter infarcts have similar etiologies and are the result of disease involving the deep penetrating arteries.
Differentiating white matter lesions related to ischemic changes from MS lesions can be difficult, especially in the older patient. This is important because 10% of patients who present with MS are older than 50 years of age. Clinical testing and history are helpful. Additionally, deep white matter infarcts tend to spare the subcortical arcuate fibers and the corpus callosum, both of which can be involved with MS. Involvement of the callosal–septal interface is quite specific for MS.
Nonspecific punctuate white matter lesions (small bright lesions on T2WIs) are more prominent in any patient with a vasculopathy, whether related to atherosclerosis (age, hypertension, diabetes, hyperlipidemia, coronary artery disease); hypercoagulable conditions; vasculitis (lupus, sarcoid, polyarteritis nodosa, Behçet syndrome); or drug-related vasculopathy. In younger individuals with punctuate white matter lesions, if a definable pathology exists, hypercoagulable states, as well as embolic and vasculitic etiologies, figure prominently
(Figs. 7.5 to 7.8). Hypercoagulable conditions include a diverse set of diseases with the common theme of increased risk of microvascular thrombotic disease. Serum testing can be used to evaluate for the presence of these disease conditions, which include homocystinemia, antiphospholipid syndrome, Factor V Leiden, prothrombin gene mutation, and deficiencies of natural proteins that prevent clotting (the anticoagulant proteins such as antithrombin, protein C, and protein S deficiencies). A classic case presentation is that of a young adult female with prior miscarriages presenting with headaches/migraines and ischemic white matter changes. These findings are suggestive of antiphospholipid syndrome (aka phospholipid antibody syndrome), where circulating antiphospholipid antibodies (cardiolipin or lupus anticoagulant antibodies) lead to a hypercoagulable state with resultant white matter and ischemic changes.
(Figs. 7.5 to 7.8). Hypercoagulable conditions include a diverse set of diseases with the common theme of increased risk of microvascular thrombotic disease. Serum testing can be used to evaluate for the presence of these disease conditions, which include homocystinemia, antiphospholipid syndrome, Factor V Leiden, prothrombin gene mutation, and deficiencies of natural proteins that prevent clotting (the anticoagulant proteins such as antithrombin, protein C, and protein S deficiencies). A classic case presentation is that of a young adult female with prior miscarriages presenting with headaches/migraines and ischemic white matter changes. These findings are suggestive of antiphospholipid syndrome (aka phospholipid antibody syndrome), where circulating antiphospholipid antibodies (cardiolipin or lupus anticoagulant antibodies) lead to a hypercoagulable state with resultant white matter and ischemic changes.
In the young adult population presenting with small white matter lesions, in addition to hypercoagulable conditions and migrainous ischemia, consider cardiogenic embolic etiologies. An echocardiogram plays an important role in the evaluation
of a potential patent foramen ovale or valvular vegetation. In many normal children and young adults, subcortical lesions and periventricular hyperintensities are common; they are reported to be present in these locations in 5% and 75%, respectively, of the young normal population. Commonly these punctuate foci of white matter T2 hyperintensity will have no known etiology despite evaluation for all the conditions outlined earlier. In this setting, these lesions may simply reflect a small focus of gliosis associated with normal perivascular space or simply the gliotic residue of a remote unspecified insult, such as an immune-mediated postviral condition. A radiologist can do considerable disservice to both patient and doctor by suggesting these punctate foci are potentially MS or posttraumatic in nature.
of a potential patent foramen ovale or valvular vegetation. In many normal children and young adults, subcortical lesions and periventricular hyperintensities are common; they are reported to be present in these locations in 5% and 75%, respectively, of the young normal population. Commonly these punctuate foci of white matter T2 hyperintensity will have no known etiology despite evaluation for all the conditions outlined earlier. In this setting, these lesions may simply reflect a small focus of gliosis associated with normal perivascular space or simply the gliotic residue of a remote unspecified insult, such as an immune-mediated postviral condition. A radiologist can do considerable disservice to both patient and doctor by suggesting these punctate foci are potentially MS or posttraumatic in nature.
Ependymitis granularis is a normal anatomic finding that may mimic pathology. Ependymitis granularis consists of an area of high signal on a T2WI along the tips of the frontal horns (Fig. 7.9). These foci of signal range in width from several millimeters to a centimeter. Histologic studies of this subependymal area reveal a loose network of axons with low myelin count. This porous ependyma allows transependymal flow of CSF, resulting in a focal area of T2 prolongation. Unfortunately, this entity has been given a name that sounds more like a disease entity than a simple histologic observation. Similarly, with the use of FLAIR imaging, a region of periventricular T2 hyperintensity can be noted about the ventricular trigones as a normal finding. With age, prominent periventricular T2 hyperintensity may be noted along the entire length of the lateral ventricles as a normal finding, and this may be referred to as senescent periventricular hyperintensity or periventricular halo.
Prominent perivascular spaces can also mimic deep white matter or lacunar infarcts. As blood vessels penetrate into the brain parenchyma, they are enveloped by CSF and a thin sheath of pia. These CSF-filled perivascular clefts are called Virchow–Robin spaces and present as punctate foci of high signal on T2WIs (Fig. 7.10). They are typically located in the centrum semiovale (high cerebral hemispheric white matter) and the lower basal ganglia at the level of the anterior commissure, where the lenticulostriate arteries enter the brain parenchyma. These perivascular spaces are typically 1 to 2 mm in diameter but can be considerably larger. They can be seen as a normal variant at any age but become more prominent with increasing age as atrophy occurs.
An important means for differentiating a periventricular space from a parenchymal lesion is the use of the proton density-weighted (first-echo T2W) or FLAIR images. On the proton density-weighted sequence, CSF has similar signal intensity as white matter. A perivascular space is composed of CSF and will parallel CSF signal intensity on all sequences (i.e., isointense to brain parenchyma on proton density sequences). In contrast, ischemic lesions, unless cavitated with cystic change, will be bright on the proton density sequence as a result of the presence of associated gliosis. Both a deep infarct and a perivascular space will be bright on the second-echo T2WI, but only the infarct will remain bright on the first-echo image. Similarly, on a FLAIR image, because fluid signal is attenuated, only true parenchymal lesions with gliosis will yield abnormal signal. On occasion, however, a small amount of persistent T2 hyperintensity can be associated with perivascular spaces on the proton density or FLAIR sequences, and this may account for many of the incidental punctate foci of hyperintensities noted in the young. An additional differentiating feature between giant perivascular spaces and lacunae is location. Lacunar infarcts tend to occur in the upper two-thirds of the corpus striatum because they reflect end-arteriole infarcts in the distal vascular distribution. In contrast, periventricular spaces are typically smaller, bilateral, and often
symmetric within the inferior third of the striatum, where the vessels enter the anterior perforated substance.
symmetric within the inferior third of the striatum, where the vessels enter the anterior perforated substance.
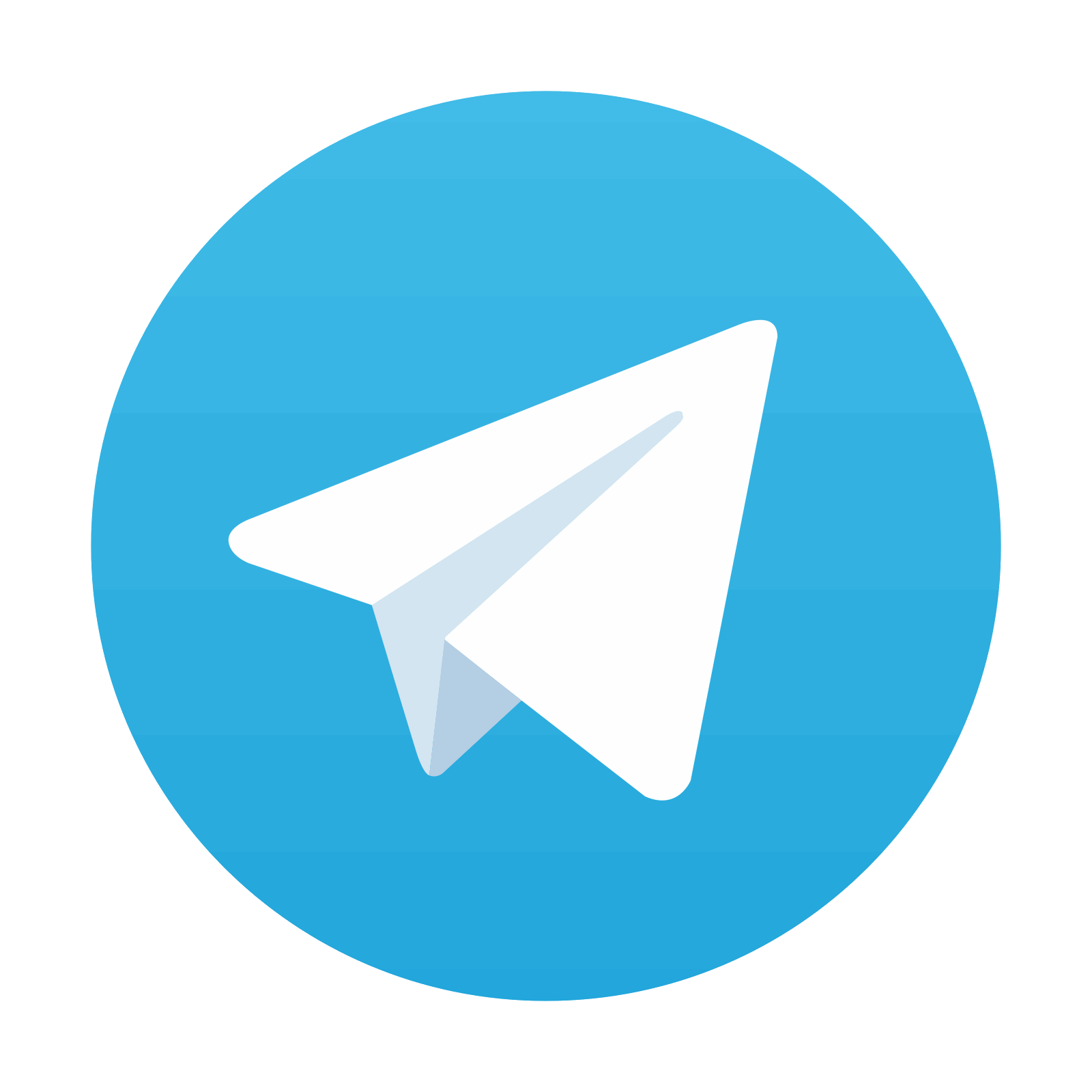
Stay updated, free articles. Join our Telegram channel

Full access? Get Clinical Tree
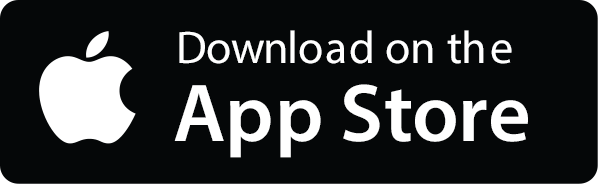
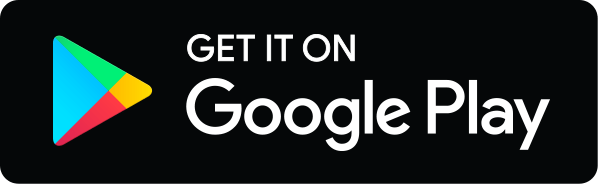