1. The structure must have a nerve supply
2. The structure should be capable of causing pain similar to what is clinically observed (e.g., when provoked in normal volunteers)
3. The structure should be susceptible to painful disease or injury; such disorders should be detectable by clinical, imaging, biomechanical, or post-mortem tests
4. The structure should be shown to be a source of pain in actual patients, using reliable and valid diagnostic tests
Table 3.2
Vertebral column structures with pain-fiber innervation
Structure |
Innervation characteristics |
1. Fibrous capsules of facet joints |
Plexus formations of unmyelinated nerve fibers, dense |
2. Ligaments: anterior longitudinal, posterior longitudinal, interspinous |
Free nerve endings |
3. Periosteum |
Plexus formations of unmyelinated nerve fibers, dense |
4. Fascia and tendons attached to periosteum |
Plexus formations of unmyelinated nerve fibers, dense |
5. Dura mater |
Plexus formations of unmyelinated nerve fibers, less dense |
6. Epidural adipose tissue |
Plexus formations of unmyelinated nerve fibers, variably dense |
7. Adventitial walls of arteries and arterioles to facet joints and cancellous bone |
Plexus formations of unmyelinated nerve fibers |
8. Walls of epidural and paravertebral veins |
Plexus formations of unmyelinated nerve fibers, dense |
9. Walls of blood vessels to paravertebral muscles |
Plexus formations of unmyelinated nerve fibers, dense |
10. Posterior anulus fibrosus of intervertebral disc |
Free nerve endings at the superficial posterior anulus |
The International Association for the Study of Pain (IASP) has published standardized definitions for spinal pain, based on perceived location of pain (Table 3.3) [11]. For example, lumbar spinal pain is pain felt to originate in an area of the low back below the T12 spinous process, above the S1 spinous process, and medial to the lateral borders of the erector spinae muscles. Sacral spine pain is felt to originate in the sacrum below the S1 spinous process, above the sacrococcygeal joints, and medial to a line between the posterior superior and inferior iliac spines [11]. Pain perceived as being localized around the spine is termed axial pain. In contrast, radiculitis or referred pain is perceived more peripherally.
Table 3.3
Spinal pain defined by IASP
Cervical spinal or radicular pain syndromes |
Thoracic spinal or radicular pain syndromes |
Lumbar spinal or radicular pain syndromes |
Sacral spinal or radicular pain syndromes |
Coccygeal pain syndromes |
Diffuse or generalized spinal pain |
Low back pain of psychological origin with spinal referral |
Pain originating from an area of the body with relatively low sensory innervation may be perceived in, or referred to, a different part of the body with greater sensory innervation from the same spinal root level. This somatic referred pain is due to convergence of high and low sensory inputs at the central nervous system level, such as spinal segmental level or thalamus [17, 21]. For example, low back pain is often perceived as spreading to the buttock. The low back is innervated by the lumbosacral dorsal rami, while the buttock is innervated by the ventral rami of the same spinal segmental levels. The quality of somatic referred pain is deep, constant aching that is diffuse and hard to localize. While it is more often an origin of referred pain, sometimes pain is referred to the lumbar spine. Pain can be referred to the lumbar spine from the lower thoracic spine, abdominal organs, or sacroiliac joints. Muscles may also refer pain to the spine [22].
Distinct from somatic referred pain, radicular pain is perceived as arising from a limb or other structure due to ectopic activation of sensory afferent fibers, typically at a spinal root level [21]. The classic quality of radicular pain is intermittent shooting or lancinating and is perceived as traveling down a limb in a narrow band, congruent with the quality of pain that has been produced by experimental stimulation of injured nerve roots [17]. However, in clinical practice, there is variation in pain referral from radiculitis; such pain patterns can be described as dermatomal, myotomal, sclerotomal, or even dynatomal [23–25]. Radicular pain is not equivalent to radiculopathy. Radiculopathy involves motor or sensory conduction block and does not cause pain by itself, but can accompany radicular or referred pain [17].
Evaluation of pain disorders begins with a careful history and evaluation, and this may often suggest that the anatomical source is the spine. The next step for the pain physician often involves a decision to pursue spine imaging or analysis of imaging that has already been ordered by referring physicians. As noted earlier, the utility of spine imaging is controversial. Low back pain with or without radiculopathy may be self-limited and may not require imaging. However, if red flags are present (Table 3.4), imaging of the spine may be warranted [7]. ACR guidelines for chronic neck pain are less clear, especially regarding whiplash. For chronic neck pain, patients with neurologic features may need MRI or CT regardless of X-ray findings, and patients without neurologic features generally do not require imaging beyond X-rays. If X-rays reveal bony or disc destruction, MRI or CT with contrast may be indicated [8]. More details on specific imaging modalities will follow below, and figures will provide examples.
Table 3.4
Red flags of back pain that may prompt spine imaging
1. Recent significant trauma, or milder trauma, age >50 |
2. Unexplained weight loss |
3. Unexplained fever |
4. Immunosuppression |
5. History of cancer |
6. IV drug use |
7. Prolonged use of corticosteroids, osteoporosis |
8. Age >70 |
9. Focal neurologic deficit with progressive or disabling symptoms |
10. Duration longer than 6 weeks |
Spine Anatomy and Function
Viewed as a whole, the typical adult spine has primary curvatures that are kyphotic in the thoracic, sacral, and coccygeal spine and secondary lordotic curvatures that develop after birth in the cervical and lumbar spine (Fig. 3.1). In the frontal plane there are typically no curvatures. A frontal curvature of more than 10° is scoliosis; thoracic kyphosis is typically limited to 30–35° and lumbar lordosis 50–60°.
A functional segmental unit of the spine consists of three joints that join adjacent vertebrae: anteriorly, the intervertebral disc (Fig. 3.2) and posteriorly, the two facet joints at that level [27, 28]. This functional unit was first described by Junghanns as a “mobile segment [29, 30].” The functional unit model applies to all mobile segments of the spine except at the atlantooccipital (C0–C1) and atlantoaxial (C1–C2) junctions, where there are no intervertebral discs. The muscles and nerves of the spine are intimately related to the functional unit. The following sections describe the specific anatomical structures of the spine, with an emphasis on function and pain.
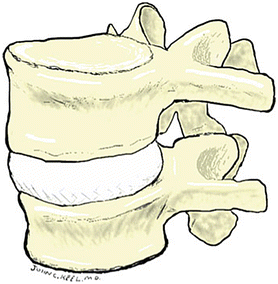
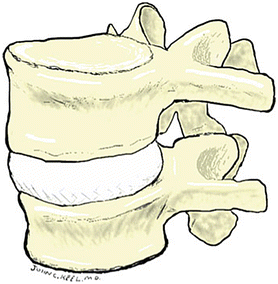
Fig. 3.2
Functional segmental unit
Osteology
The vertebral column as a whole consists of 33 vertebrae: 7 cervical, 12 thoracic, 5 lumbar, 5 fused sacral, and 4 fused coccygeal [31]. Vertebrae are irregular, composite bones, consisting of a ventral body and a dorsal neural arch. The exception is the atlas, which has no vertebral body. The load-bearing system of the vertebral bones can be understood as a tripod: one vertebral body interface and two (paired) facet joint interfaces [32]. For example, lumbar vertebral bodies bear 80–90 % of the weight load, and lumbar pedicles and laminae function as struts, supporting the tripod “legs.” Pedicles connect the anterior and posterior weight-bearing elements, spanning from the transverse process to the body. Transverse processes originate at the junction of the pedicle and the lamina, while the spinous process originates at the junction of right and left laminae. The functional significance of the transverse and spinous processes is to increase the moment arm of muscles attaching to these sites [17, 32]. The vertebral bodies have thin cortical bone and cancellous trabecular bone in vertical, oblique, and horizontal patterns [31]. This overlapping trabecular medullary structure provides great strength, but the relative lack of overlap in the anterior portion may predispose to compression fractures.
Cervical vertebrae (Fig. 3.3) bear the least weight, and their vertebral bodies are relatively narrow in AP dimension and have distinct uncinate processes at their superolateral borders, which articulate with the vertebral body above (uncovertebral joint). Vertebrae C1–C6 have bilateral foramen transversarium containing the vertebral artery (C7 has this foramen without the vertebral artery). As shown in the figure, the vertebral artery is very susceptible to injury in the anterolateral approach for cervical transforaminal injections (Fig. 3.4). The transverse processes have anterior and posterior projections for attachment of muscles, and the projections form a groove which contains the exiting spinal nerve. The cervical articular pillars are oblique to the horizontal plane and appear stacked as a column. The atlas, C1, has no vertebral body and has unique articular processes for occipital condyle and axis. The axis, C2, is most recognized by the odontoid process or dens. The occipital-atlantoaxial complex allows nodding and rotational movements: about 50 % of flexion-extension is at C0–C1, and 50 % of rotation is at C1–C2. C6 transverse process anterior tubercle is known as Chassaignac’s tubercle (also known as carotid tubercle, an important landmark for stellate ganglion blocks). Vertebra C7 has a larger inferior body, a large spinous process (vertebra prominens), and steeply sloping articular pillars.
The defining features of the 12 thoracic vertebrae are the costotransverse and costovertebral articulations of the ribs (Fig. 3.5). Thoracic facets are arranged in the coronal plane in a way that allows rotation and side bending.
Typical features of lumbar vertebrae are shown (Fig. 3.6). L5 is usually largest and more wedge-shaped when viewed laterally, which allows the superior aspect of L5 to be closer to horizontal [34]. The L5 disc is also wedge-shaped, allowing 16° average angle between the respective superior and inferior surfaces of S1 and L5 [35]. L5 has thick transverse processes but has the smallest lumbar spinous process. L1 has the shortest and L3 the longest transverse processes [36]. L5–S1 facets tend to be more flat, while other levels are more curved [31]. Because L5 is angled, more of its axial load is transmitted by its posterior elements, including the transverse processes and iliolumbar ligaments [37]. The lumbar vertebral bodies have indentations in the posterior aspects of superior and inferior rims, vestigial uncinate processes that may contribute to posterolateral disc protrusions [17]. The mamillary process is an attachment site for longissimus and rotator muscles. The accessory process is thought to be a vestigial costal process and is an attachment site for longissimus muscles. The mamilloaccessory ligament joins these two processes; this structure may be an obstacle in medial branch blocks or radiofrequency ablation.
The sacrum (Fig. 3.7) is formed of five fused vertebrae and vestigial costal elements. The sacrum articulates with the ilium. The sacrum has a central canal which is the terminal portion of the spinal canal. The ventral primary rami of S1–S4 exit via anterior foramina, and the posterior rami exit the posterior foramina. The center of mass is anterior to the vertebral column in 75 % of adults, typically 2 cm anterior to S2 [31]. The sacrum is tilted forward so that the most superior sacral end plate is 50–53° from horizontal [31, 38]. The coccyx is usually four vertebrae, with the first being larger and having cornua.
Arthrology
Movements of the functional unit of the spine include flexion, extension, side bending, and rotation. The zygapophyseal joints limit motion depending on their orientation and function to protect the intervertebral discs from rotational and translational strains [36]. The cervical spine has the greatest mobility. The thoracic spine is limited by the rib cage, as well as long, overlapping spinous processes. The lumbar spine is a relatively mobile section between the less mobile thoracic and sacral sections of the spine.
A facet joint is a diarthrodial joint formed from the superior articular process of the more caudal vertebra and the inferior articular process of the more cephalad vertebra. The facet joint capsule exists at the dorsal, superior, and inferior margins and consists of the ligamentum flavum itself anteriorly [31]. The superior and inferior aspects of the joint capsule have loose pockets, subcapsular recesses that contain fat. Facet joints contain hyaline cartilage and meniscoid structures: fibroadipose meniscoids, adipose tissue pads, and connective tissue rims [17, 31, 39].
Facet joints have been known to cause pain since Goldwaithe’s report in 1911 [40]. Ghormley coined the term “facet syndrome” to describe lumbosacral pain in 1933. Mooney and Robertson demonstrated referred pain from facet joints [41]. Lumbar facet joints bear about 20 % of the axial load and 40 % of the torsional and shear strength (as a pair). Recalling the tripod model, if the intervertebral disc is degenerated, the facets may bear up to 70 % of the axial load in that segment. They also bear more axial load with increased lumbar lordosis [27, 42]. Cervical and thoracic facet joints are also pain generators.
The sacroiliac joints consist of a C-shaped synovial portion and a syndesmosis portion (Fig. 3.8). The articular surface of the ilium is fibrocartilage, while the sacrum is hyaline cartilage that is much thicker [43]. Normal motion is slight, 2–3° in the transverse or longitudinal planes. Nutation (nodding) is the rotation of the sacrum that causes forward tilting in relation to the ilium. Counternutation is the opposite movement, where the sacrum tilts posteriorly in relation to the ilium [27]. The sacroiliac joints can also be a source of pain.
Brief mention is warranted that other spine joints are sometimes considered pain generators: atlantooccipital, atlantoaxial, uncovertebral, costotransverse, and costovertebral, have all been described as sources of pain. A transitional lumbosacral junction may have an assimilation joint, wherein a large L5 transverse process articulates with the sacrum. A pars defect may form a pseudoarthrosis that can be painful.
Intervertebral Disc: A Special Joint
Mixter and Barr, respectively, from the neurosurgery and orthopedic surgery services of Massachusetts General Hospital, are often cited as the first to recognize the importance of intervertebral disc herniations, although Mixter and Barr themselves cite several prior reports [44]. Although the intervertebral disc has been compared to a diarthrodial joint, it is correctly classified as a symphysis, a major cartilaginous synarthrosis [45]. Adjacent vertebral levels are separated by an intervertebral disc (except at C0–C1 and C1–C2). The functions of the disc are to distribute force and allow movement between adjacent vertebral bodies and to hold the vertebral bodies together. The discs also comprise 20–25 % of the total length of the spine, separating the vertebrae, allowing nerve roots and vessels to travel between vertebrae [27].
The intervertebral disc is the largest avascular structure in the body [46]. Each disc contains superior and inferior cartilaginous end plates, anulus fibrosus, and nucleus pulposus. Nucleus pulposus is 70–90 % water, variable with age, and proteoglycans are the majority of the remainder. Type II collagen fibers join with proteoglycans, forming the matrix of the nucleus. Embedded in the proteoglycans, toward the end plate regions, are chondrocytes that synthesize the substance of the nucleus.
The nucleus pulposus functions to bear weight and distribute forces across vertebral segments. The healthy nucleus distributes load very evenly, in a manner as a fluid, according to Pascal’s law, a mechanism that dissipates force and prevents injury [32]. With age, the glycosaminoglycan and water content of the nucleus decrease, and the fibrocartilaginous content increases. This transfers load bearing to the anulus and other structures.
The anulus fibrosus is the external ring of the disc and is formed by 10–20 sheets of parallel collagen fibers (lamellae), each sheet having fibers oriented in alternating directions 30–70° from vertical. The anulus is mostly water (60 %). Collagen is half of the remaining portion. The anulus can be divided into three zones: the outer zone is made of fibrocartilaginous Sharpey’s fibers that attach to the vertebral body. The intermediate and inner zones are also fibrocartilage, but do not attach to the vertebral body. The posterolateral fibers of the lumbar anulus are most prone to injury and degeneration. While the posterocentral disc may be protected by attachments of posterior longitudinal ligament, there are no extrinsic ligament attachments to support the posterolateral disc [47]. The lumbar posterior anulus is thinner radially due to the eccentric location of the nucleus. It is also longitudinally thinner, due to the lordotic curve of the lumbar spine, which means that a given amount of displacement causes relatively greater strain on these shorter fibers.
Vertebral end plates are cartilaginous structures at the superior and inferior aspect of each vertebral body, between the body and the disc. They are firmly attached to the disc by the collagenous fibers of the lamellae (intermediate and inner zones), which invest the fibrocartilaginous side of the end plate. Blood vessels on the surface of the vertebral bodies contact the hyaline side of the end plate, and nutrients diffuse through the end plate to the disc, a process of imbibition [ 48].
Several studies have confirmed nerve fibers in the outer portions of the anulus fibrosus, especially the posterolateral disc, likely rendering the disc capable of becoming a pain generator. Disc innervation may arise from sinuvertebral nerve, ventral rami direct branches, and rami communicans (Table 3.5) [17, 49–52, 54–57].
Table 3.5
Human studies on sensory innervation of intervertebral disc
Reference | Finding |
---|---|
Roofe [49] | Nerve fibers in disc and adjacent PLL |
Malinsky [50] | Nerve endings in lateral outer 1/3 of annulus, encapsulated and non-encapsulated |
Rabischong et al. [51] | Confirmed above |
Yoshizawa et al. [52] | Confirmed above |
Also reported morphology similar to other known nociceptors | |
Bogduk [53] | Confirmed above |
Immunohistochemical studies demonstrating sensory and autonomic fibers in the annulus |
Pressure within the disc is affected by body position. The natural lordotic posture of the lumbar spine reduces vertical pressure on the disc. Direct vertical pressure increases fluid pressure within the disc. This can even lead to herniation of nucleus pulposus through defects in the end plate, known as Schmorl’s nodes [first observed by Luschka [31]]. Fluid shifts in the disc also account for the 1–2 cm decrease in height that can be observed after a day of upright posture compared to the morning [58]. Disc pressure when standing is only 35 % of pressure when seated, while lifting greatly increases disc pressure and can be estimated at ten times the weight of the object lifted, as demonstrated in Nachemson’s classic study [59].
Disc herniations can be classified as protrusion, extrusion, or sequestration. A protrusion has a base wider than the greatest extent of herniation (Fig. 3.9). Nucleus material extends into the epidural space in an extrusion, and the extent of herniation may exceed the width of the base (Fig. 3.10). In a sequestration, the nucleus material has been extruded as a fragment into the epidural space and has lost continuity with the disc. For further standardized terminology for description of location and type of disc herniations, the reader is referred to “Nomenclature and Classification of Lumbar Disc Pathology. Recommendations of the Combined Task Forces of the North American Spine Society, American Society of Spine Radiology, and American Society of Neuroradiology [47].”
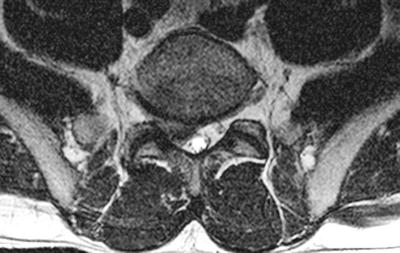
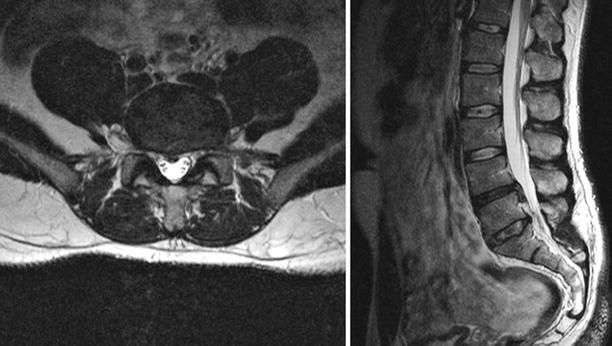
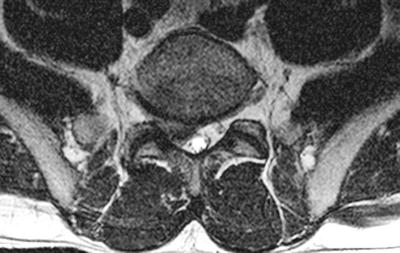
Fig. 3.9
Lumbar disc protrusion, rightward at L5–S1
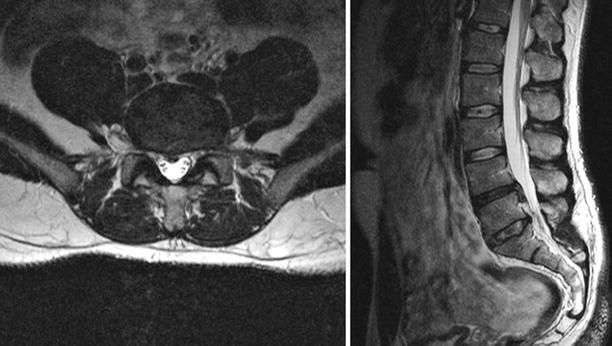
Fig. 3.10
Lumbar disc extrusion, central at L4–L5 with caudal subligamentous migration
Ligaments of the Spine
Ligaments of the spine are detailed in Tables 3.6, 3.7, and 3.8 [17, 32]. Of great practical interest to the pain physician is the ligamentum flavum, a paired structure, running from lumbar to cervical spine, composed of highly elastic fibers. There may be a narrow gap between the right and left halves [36]. It forms the posterior boundary of the epidural space as approached between vertebral lamina and may contribute to the boundary in the intervertebral foramen. On right and left, a ligamentum flavum attaches from the superior vertebral level to the adjacent level below. Laterally, fibers of ligamentum attach to the facet articular processes and form part of the joint capsule. Lateral fibers may attach to the pedicle. The lateral fibers of ligamentum contribute to the posterior boundary of the intervertebral foramen. In the lumbar spine, ligamentum flavum may be the strongest and most elastic of spinal ligaments and resists distraction and flexion. In the lumbar spine, ligamentum flavum can be 2–3 mm thick [36]. The elasticity is important to prevent buckling of the ligament, which can compress neural structures. Buckling can occur if there is loss of intervertebral disc height. However, the ligamentum flavum is less robust in the cervical and upper thoracic spine, and its paper thinness and midline absence may affect cervical or thoracic interlaminar injections [60]. The interspinous and supraspinous ligaments are relatively weak and are often the first to be sprained [31, 61]. The iliolumbar ligament is a complex structure that begins as a muscle and becomes a ligament in the third decade and is only present in adults [31, 62].
Table 3.6
The ligaments of the spine
Ligament | Attachments | Function | Comments |
---|---|---|---|
Anterior longitudinal ligament | Cervical to sacral | Counters extension | Lumbar is most developed |
Stabilizes lordosis | |||
Restricts listhesis | |||
Posterior longitudinal ligament | Cranium to sacrum | Resists flexion | Becomes tectorial ligament in cranium |
Reinforces posteromedial disc | Can become ossified and cause stenosis | ||
Ligamentum flavum | Cervical to lumbar | Resists distraction and flexion | See text |
Bifid | |||
Interspinous | Adjacent spinous processes | Resists distraction and flexion | Lumbar is most developed |
Cervical may be absent | |||
Supraspinous | Runs dorsally over spinous processes | Resists distraction and flexion | |
Terminates inferiorly at L4 in 73 %, L3 in 22 %, L5 in 5 %, is rarely at L5–S1 | |||
Iliolumbar | See separate table | ||
False ligaments | See separate table |
Table 3.7
The iliolumbar ligament
Portion | Origin | Insertion |
---|---|---|
Anterior | Anteroinferior border and tip of L5 transverse process | Ilium |
Superior |