10 Neurofunctional Systems
“Neurofunctional systems” are orders of neuron populations that process and transmit afferent or efferent signals while processing neural information and include, for instance, gustatory, auditory, visual, olfactory, or motor functions. Descriptions in this chapter have been restricted to those systems that may easily be tested clinically and are known to be of diagnostic significance. The science of neuronal pathways, hodology, collates findings from studies in neurohistology, neurophysiology, ontogenetics, neuropathology, animal experiments, neurology, neurosurgery, and neuroradiology. For example, the somatotopic order of the pyramidal tract in the precentral gyrus was first identified in 1870 by neurosurgical interventions performed by Fritsch and Hitzig177 and has been corroborated several times thereafter.170 , 424 , 453 The boundary between the corticospinal and the corticonuclear cortex fields, that is, between the motor innervation of the trunk and that of the head, lies approximately at the boundary between the two-thirds of the precentral gyrus being close to the superior cerebral margin and the third of the precentral gyrus close to the lateral sulcus. There exists a regional overlap of areas perceiving a stimulus from the trunk-extremity region (corticospinal neurons) and from the head (corticonuclear neurons).
Contractions of different muscles were recorded170 , 453 during repeated stimulation of an identical site of the precentral gyrus. Only a gradual reduction in the size of the Betz giant cells may be seen on neurohistology from the superior cerebral margin to the lateral sulcus in area 4. Distinct labeling methods have been used in animal experiments to localize neuronal connections between individual cortical nerve cells and the motor periphery.
In recent years, PET, fMRI, and MEG have been successfully used as imaging techniques for localization of somatosensory and motor areas.137 , 294 , 398 The illustrations in this book therefore represent only current state of knowledge pertaining to probable topography of neurofunctional systems.
Neurofunctional systems are incompletely developed in prenatal life and nerve cells possess great plasticity in embryonal life. The younger the brain, the better it compensates for neuronal lesions. If the cerebellum fails to develop in the initial embryonic period due to genetic or external causes, other neuronal populations can take over its functions almost completely. Cases of congenital hypoplasia of the cerebellum have been described with complete absence of cerebellar symptoms during life. Similar observations have been noted with congenital aplasia of the corpus callosum.593 Even infant brains can compensate for neurofunctional deficiencies much better than adult brains. However, perinatal lesions of certain neuronal structures may cause severe functional loss, such as blindness following bilateral occipital cortical defects. The ability to compensate for neurofunctional damage in childhood is probably due to synaptic connections between existing neurons. Studies on mammals have shown that a larger number of nerve cells are formed and developed perinatally than those that begin functioning later in the postnatal period. Recent findings suggest that the adult brain also has a great potency for cortical reorganization after brain damage.9 , 98 , 205 , 226 , 329 The field of stem-cell research examines the role of stem cells in the reorganization of both the infant and the adult brain.286 , 550 , 570
The localization theory, which assigns specific functions to individual neuronal populations in the central nervous system, was based primarily on neurological findings of school children, adolescents, and adults. With increasing complexity of cognitive, as also emotional–motivational functions, localization in clearly defined neuronal populations becomes increasingly difficult. These complex functions have been described in Section 10.13.
Newer approaches for the depiction of complex neuronal networks may illustrate interactions between different regions of the brain and thus explain why lesions in multimodal areas may result in loss of completely different higher functions. Targeted examination of resting activation of the brain has expanded our understanding of its functions, so that activation in characteristic networks may be observed even in the absence of external stimuli.171
A new interdisciplinary subdivision within neurosciences, so-called connectomics, examines the importance of neuronal networks in intellectual functions under conditions of activation and rest. The term connectome refers to the totality of all connections in the nervous system of a living being. In 1986, the biologist John White and his colleagues succeeded in completely reconstructing the nervous system of the nematode Caenorhabditis elegans using electron microscopy.614
Implementing a similar project in the human brain would be far more complicated because of the much larger number of neurons, but this has been addressed by the Human Connectome Project (National Institutes of Health) since 2010. At the heart of this project is the macroscopic depiction of individual connectomes of test subjects using imaging procedures. Anatomical or functional properties are, for instance, employed to depict connections between brain regions as nodes in network graphs. Influencing factors for the expression of these connections, such as (epi)genetic factors, the influence of learning processes or pathological changes in the brain substance may also be included in the models of these network graphs.
It must be noted that active states do not indicate why a particular region is required for a specific function; the principle of the network elucidates why discrete lesions may result in diffuse damage.493 Currently available data reveals that, despite the presence of discrete neurofunctional systems, the number of anatomically clearly distinguishable subdomains responsible only for a specific function is comparatively low. The brain appears to represent a complex, manifold interconnected macrosystem in which individual brain regions are involved in a variety of physiological, cognitive, and emotional–motivational functions.
Positions of the neurofunctional systems have been depicted in series of slides and illustrated in the atlas in ▶Fig. 3.2, ▶Fig. 3.3, ▶Fig. 3.4, ▶Fig. 3.5, ▶Fig. 3.6, ▶Fig. 3.7, ▶Fig. 3.8, ▶Fig. 3.9, ▶Fig. 3.10, ▶Fig. 3.11, ▶Fig. 3.12, ▶Fig. 3.13, ▶Fig. 3.14, and ▶Fig. 3.15 (coronal series), ▶Fig. 4.2, ▶Fig. 4.3, ▶Fig. 4.4, ▶Fig. 4.5, ▶Fig. 4.6, and ▶Fig. 4.7 (sagittal series), ▶Fig. 5.2, ▶Fig. 5.3, ▶Fig. 5.4, ▶Fig. 5.5, ▶Fig. 5.6, ▶Fig. 5.7, ▶Fig. 5.8, ▶Fig. 5.9, ▶Fig. 5.10, ▶Fig. 5.11, ▶Fig. 5.12, ▶Fig. 5.13, ▶Fig. 5.14, and ▶Fig. 5.15 (bicommissural series), and ▶Fig. 6.4, ▶Fig. 6.5, ▶Fig. 6.6, ▶Fig. 6.7, ▶Fig. 6.8, ▶Fig. 6.9, ▶Fig. 6.10, ▶Fig. 6.11, ▶Fig. 6.12, and ▶Fig. 6.13 (brainstem series).
Neuronal networks presented in Section 10.13 have been illustrated in ▶Fig. 10.44, ▶Fig. 10.45, ▶Fig. 10.46, ▶Fig. 10.47, ▶Fig. 10.48, ▶Fig. 10.49, ▶Fig. 10.50, ▶Fig. 10.51, ▶Fig. 10.52 in lateral and/or medial views, respectively, in a highly simplified manner based on conventionally depicted fMRI data. The difficulties inherent in transposing cadaveric neuroanatomical findings to in vivo studies have been critically reviewed in Section 1.3 and must be taken into consideration in the analysis of neurofunctional systems. Only a few studies about individual variability of neurofunctional systems have been published to date.84 , 191 , 319 , 527 , 608 Despite these limitations, hemianopia, ataxia, aphasia, and many other neurologic syndromes are diagnosed daily in clinical practice and are correlated with the probable site of the lesion. The principal neurofunctional pathways depicted in this book in parallel coronal, sagittal, and bicommissural planes should serve as an aid to diagnosis. Current hodological knowledge is being constantly upgraded by scientific correlation of clinical findings with results of modern imaging and functional diagnostic procedures.
10.1 Sensory Systems
10.1.1 Anterolateral System
The anterolateral system (see ▶Fig. 10.1 and ▶Fig. 10.2) receives its signals from nociceptors (pain), thermoreceptors (heat, cold), and mechanoreceptors located in the legs, trunk, arms, and neck. The cell bodies of the first-order neurons together with their pseudounipolar nerve cells lie in the spinal ganglia and their central axons terminate in the sensory neurons of the posterior horns of the spinal cord. Second-order neurons arising here extend in a cranial direction as the anterior and lateral spinothalamic and the spinoreticular tracts. The spinothalamic tracts cross in the anterior white commissure of the spinal cord and then ascend contralaterally in the anterolateral tract. The spinoreticular tract extends as a polysynaptic pathway to the medial reticular formation of the hindbrain and further upward to the intralaminar nuclei of the thalamus. These thalamic neurons project widely throughout the cerebral cortex, notably into certain cortical areas of the cingulate gyrus and the prefrontal cortex. Psychological pain is probably perceived in the cingulate gyrus.651
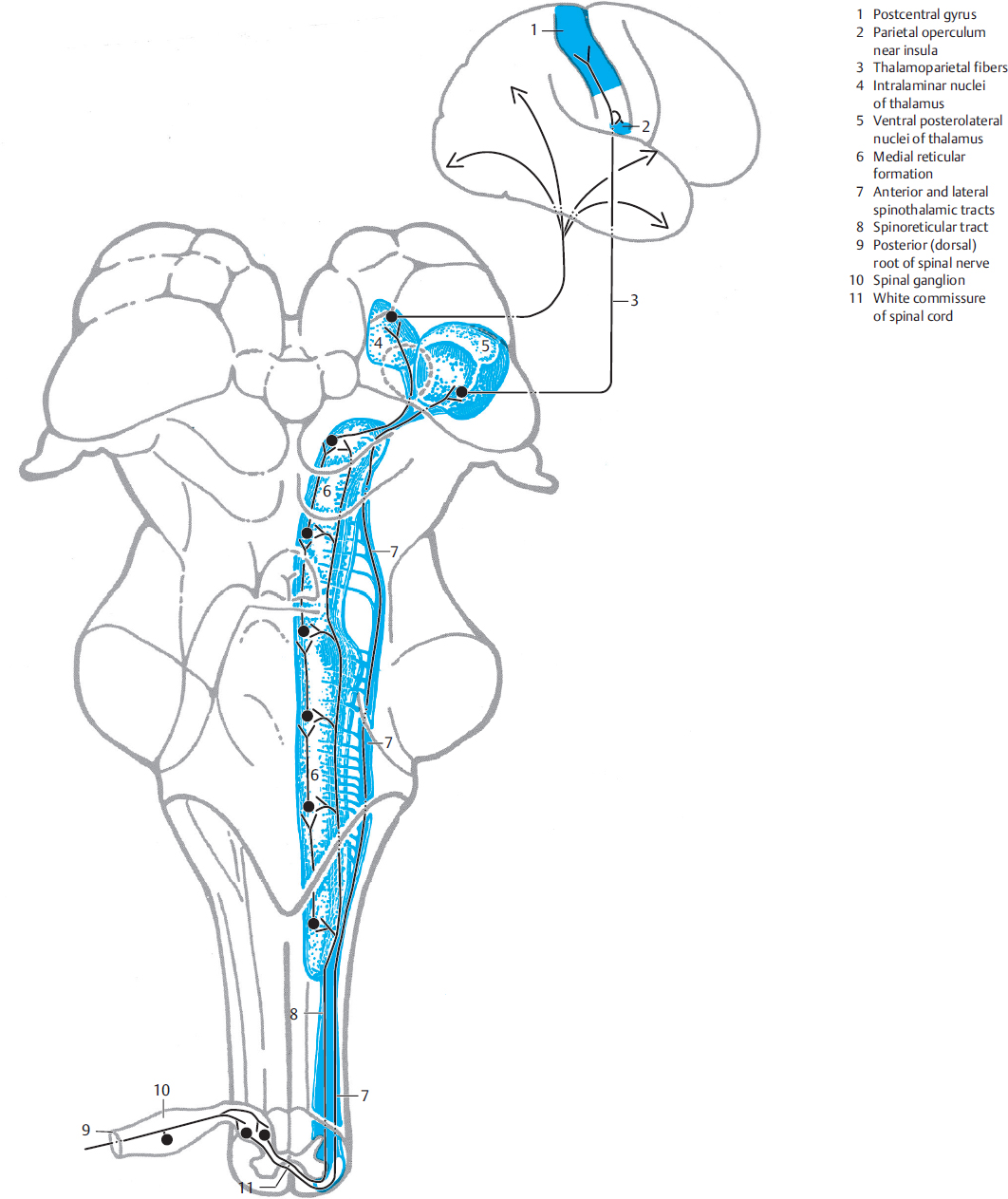
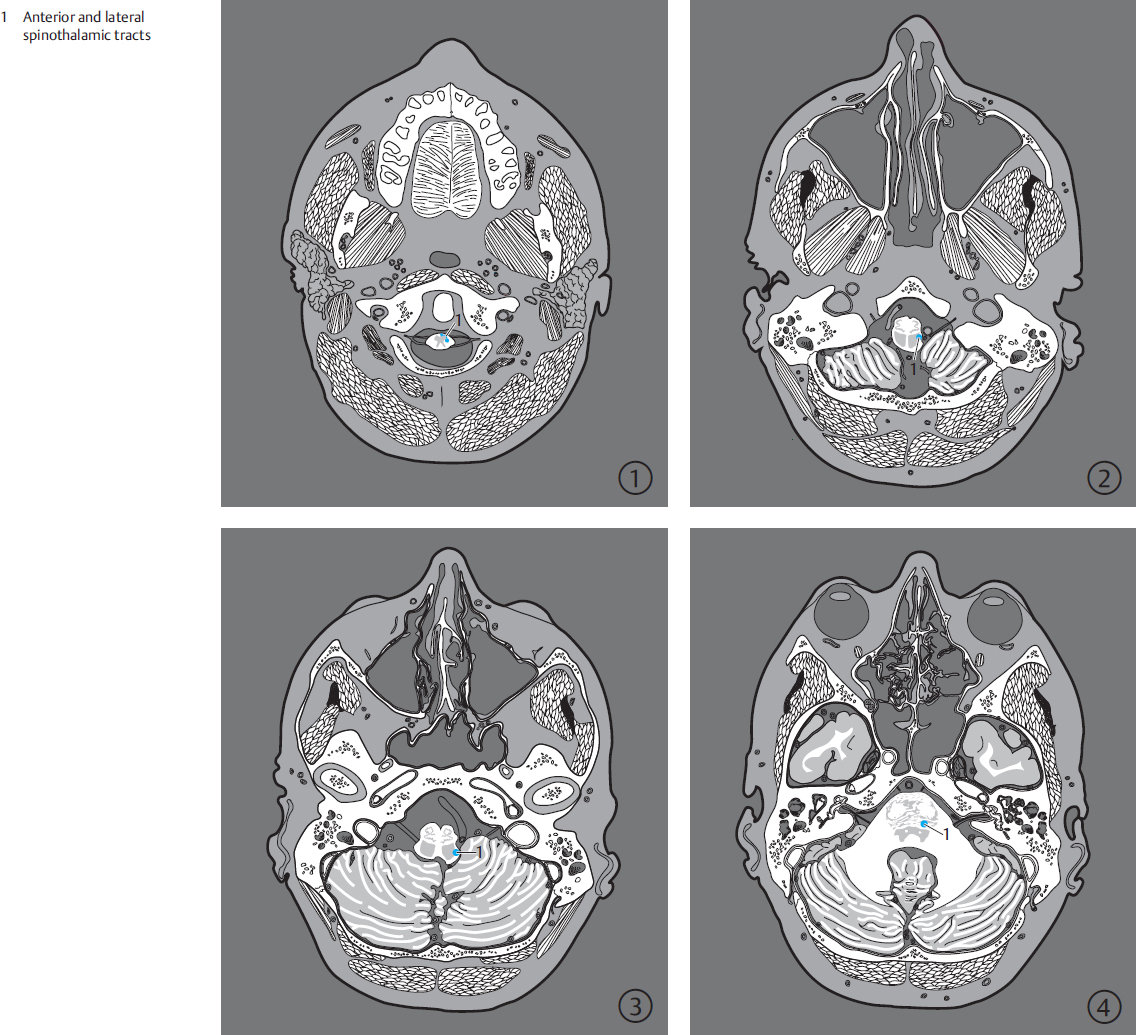
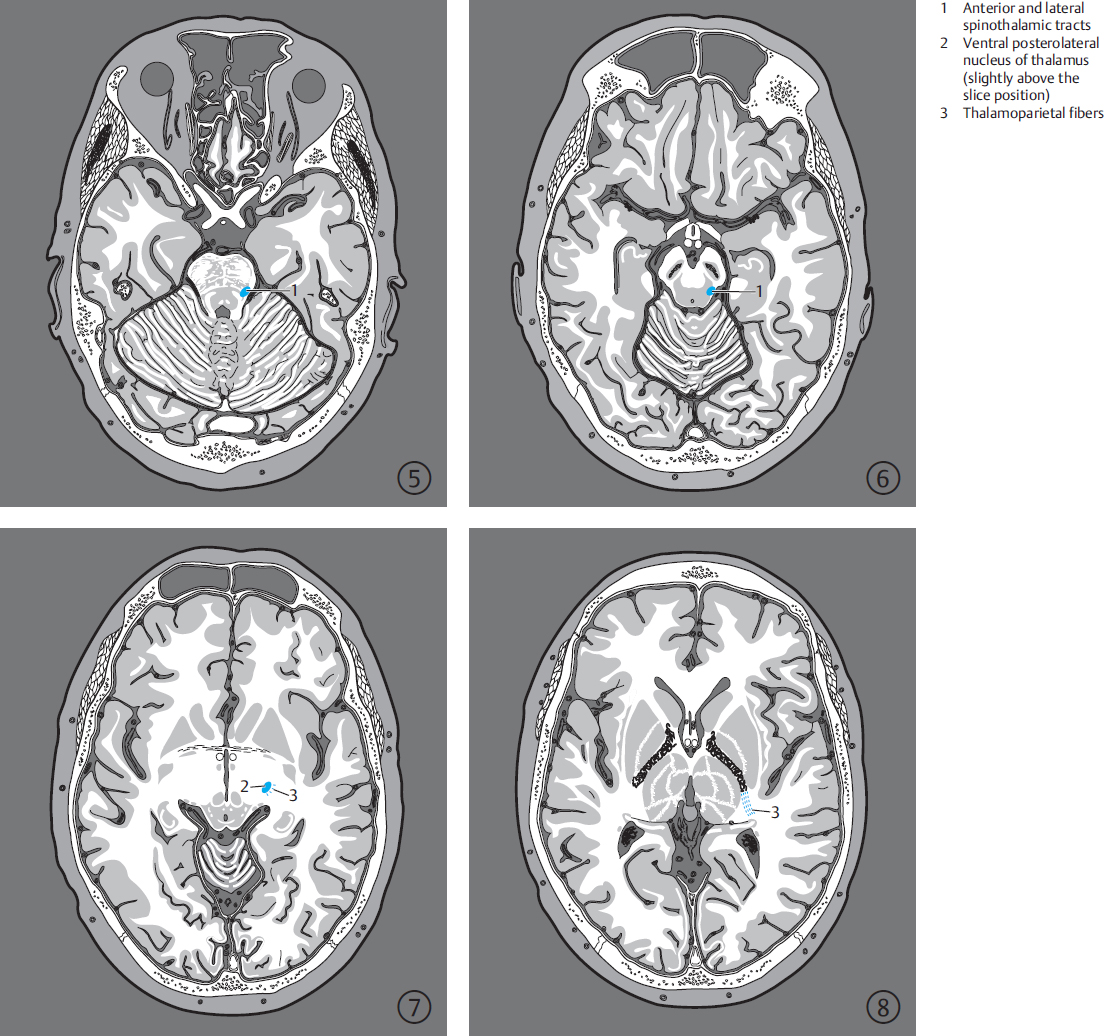
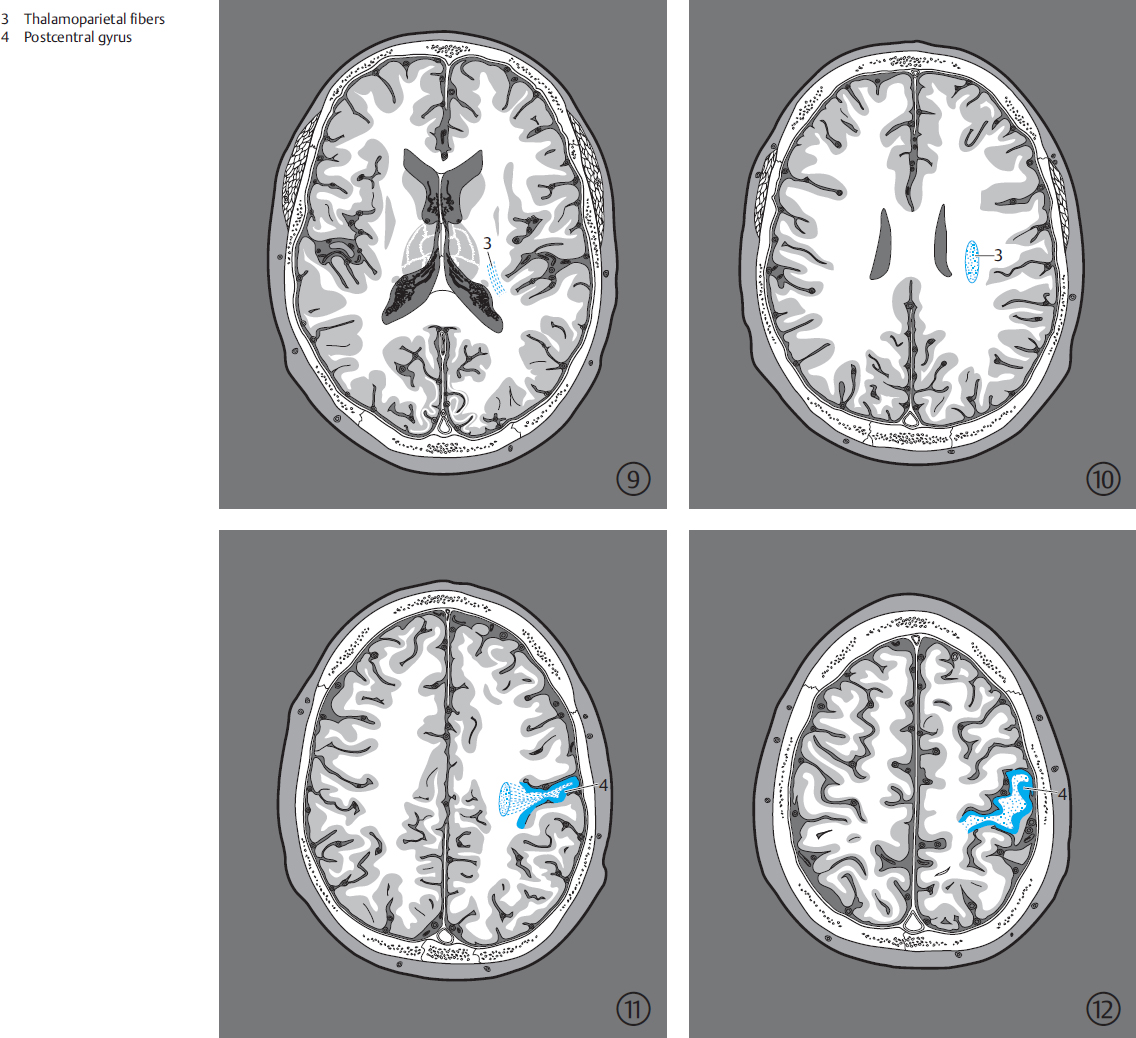
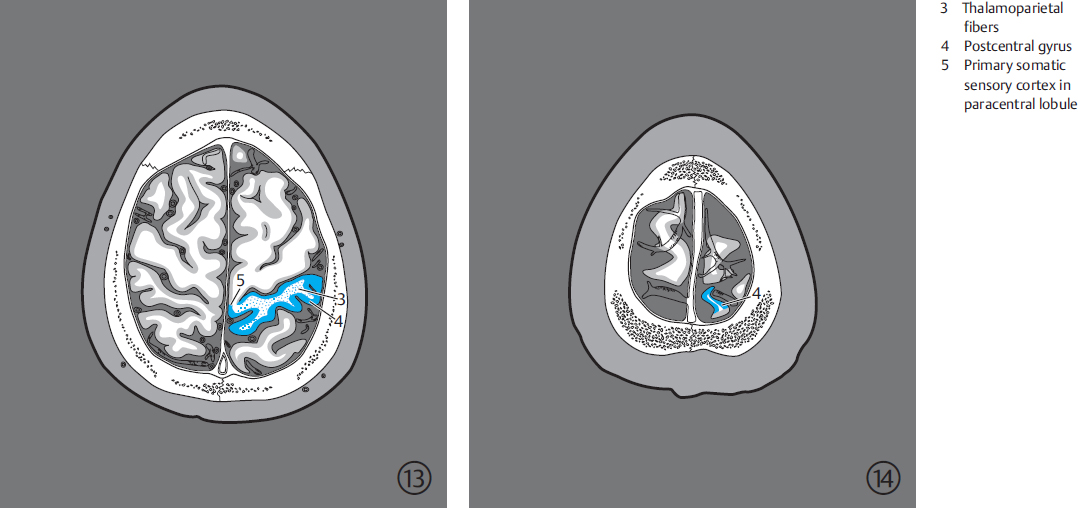
The united spinothalamic tract passes lateral to the reticular formation trough the medulla oblongata and pons. It joins the medial lemniscus in the pons–midbrain region, where an isolated lesion involving only one pathway is unlikely. The spinothalamic tracts terminate in the ventral posterolateral nuclei of the thalamus; axons of the third-order neurons pass from here as thalamoparietal fibers through the posterior limb of the internal capsule to the postcentral gyrus. Located in the postcentral gyrus is the sensory projection field with cytoarchitectonic fields 3, 1, and 2 as described by Brodmann (see ▶Fig. 7.53). These somatosensory areas are arranged in a somatotopic fashion in this small cortical region: projection fields for the contralateral leg are localized at the superior margin of the cerebral hemisphere. The areas for the trunk, arm, and neck of the opposite side lie in the upper two-thirds of the postcentral gyrus between the superior margin and the lateral sulcus (see ▶Fig. 10.1). A secondary pain area is additionally present in the parietal operculum (see ▶Fig. 10.1). Pain is consciously localized in both primary and secondary areas. Interruption of the spinothalamic tract results in disorders of pain and temperature perception.625
10.1.2 Medial Lemniscus System
The sensory receptors of the medial lemniscus system (dorsal column pathway; see ▶Fig. 10.3, ▶Fig. 10.4, and ▶Fig. 10.5) are mechanoreceptors in the skin, muscle spindles, tendon organs and other proprioceptive stimulus converters in the legs, trunk, arms, and neck. Cell bodies of first-order neurons lie in the spinal ganglia. The axons of these unipolar nerve cells are arranged in a somatotopic fashion in the dorsal column of the spinal cord, that is, the pathways from each dermatome are organized in layers. Axons from the caudal half of the body run in the gracile fasciculus (medial spinobulbar tract) while those from the cranial half form the cuneate fasciculus (lateral spinobulbar tract).
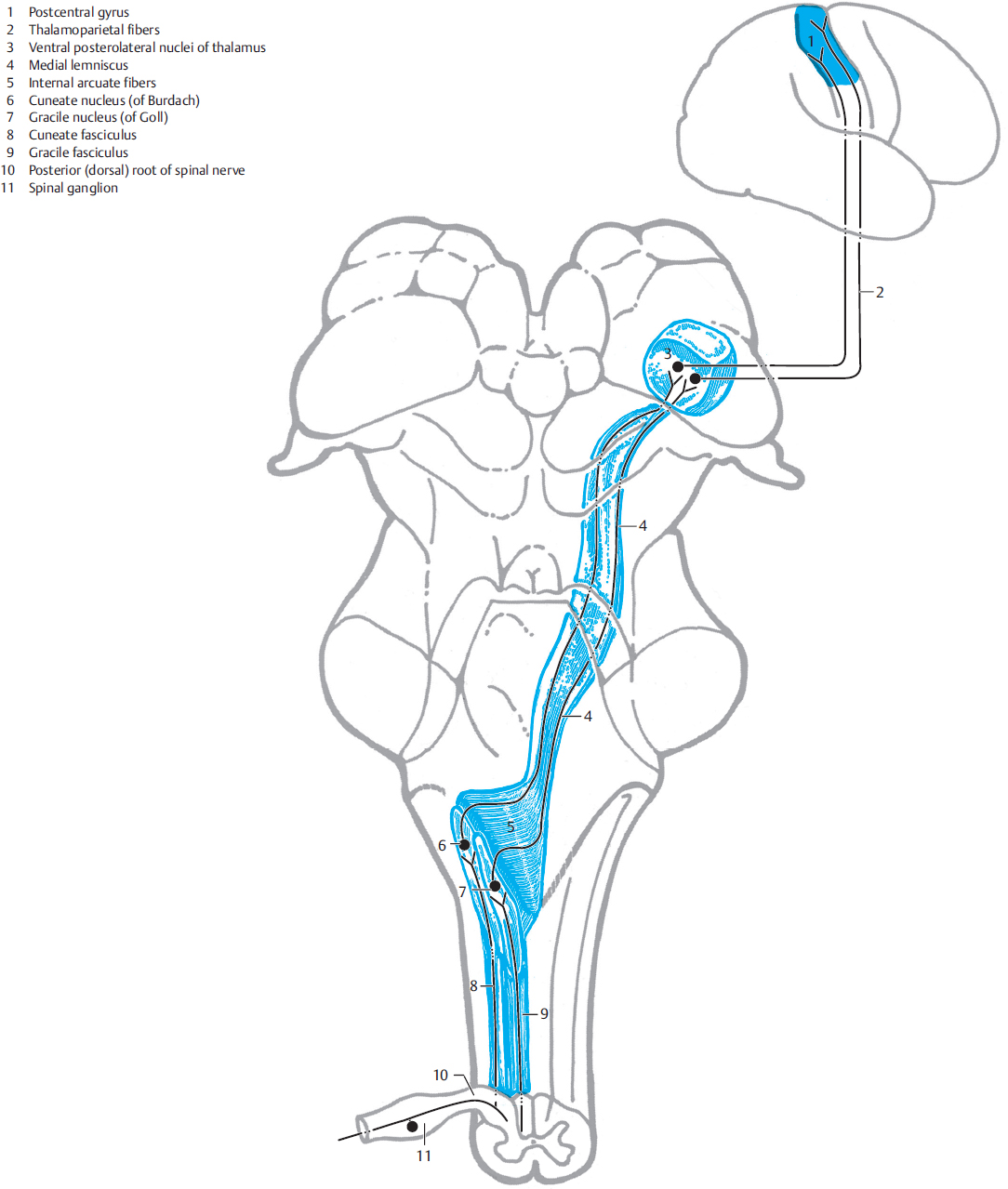
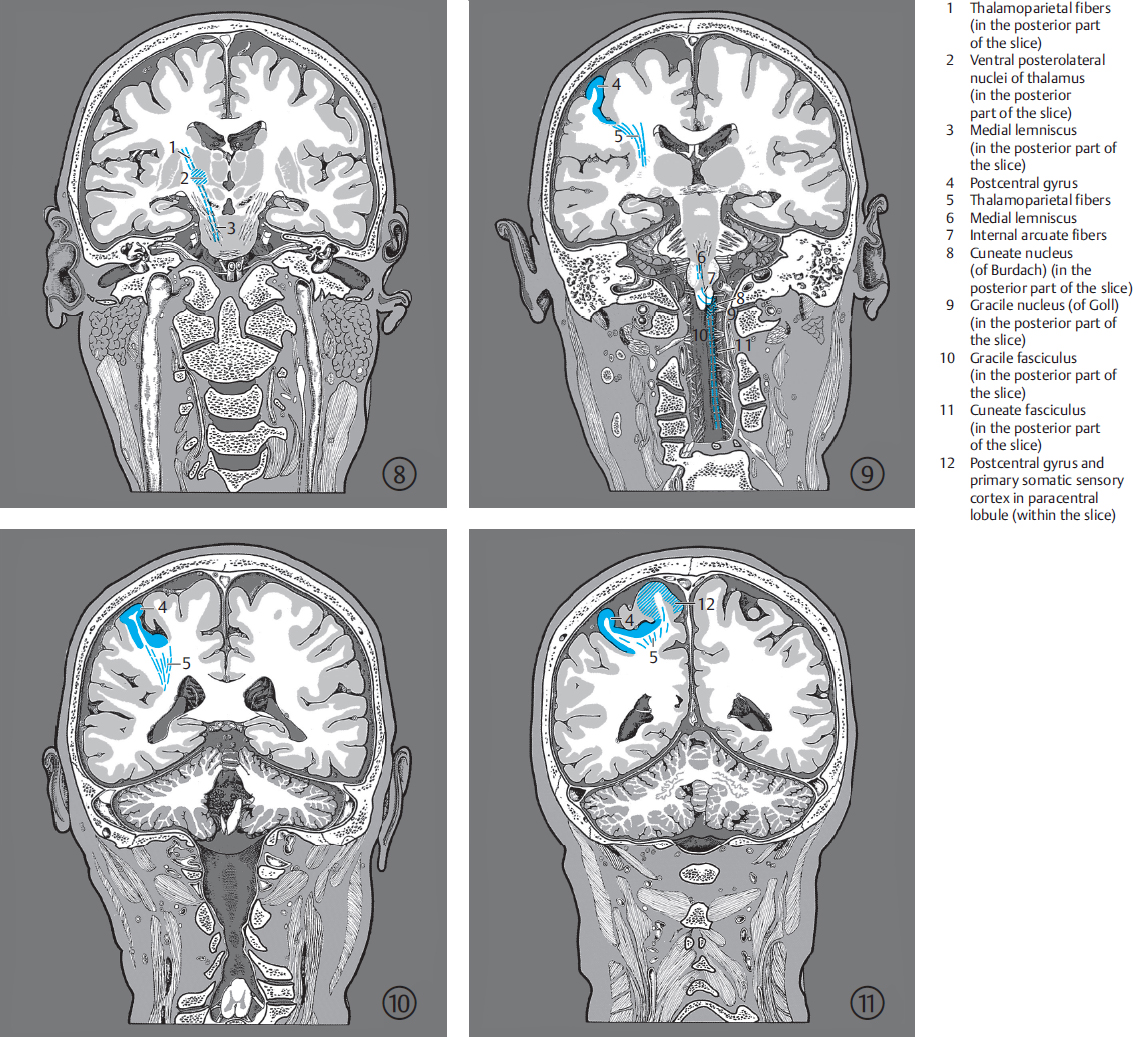
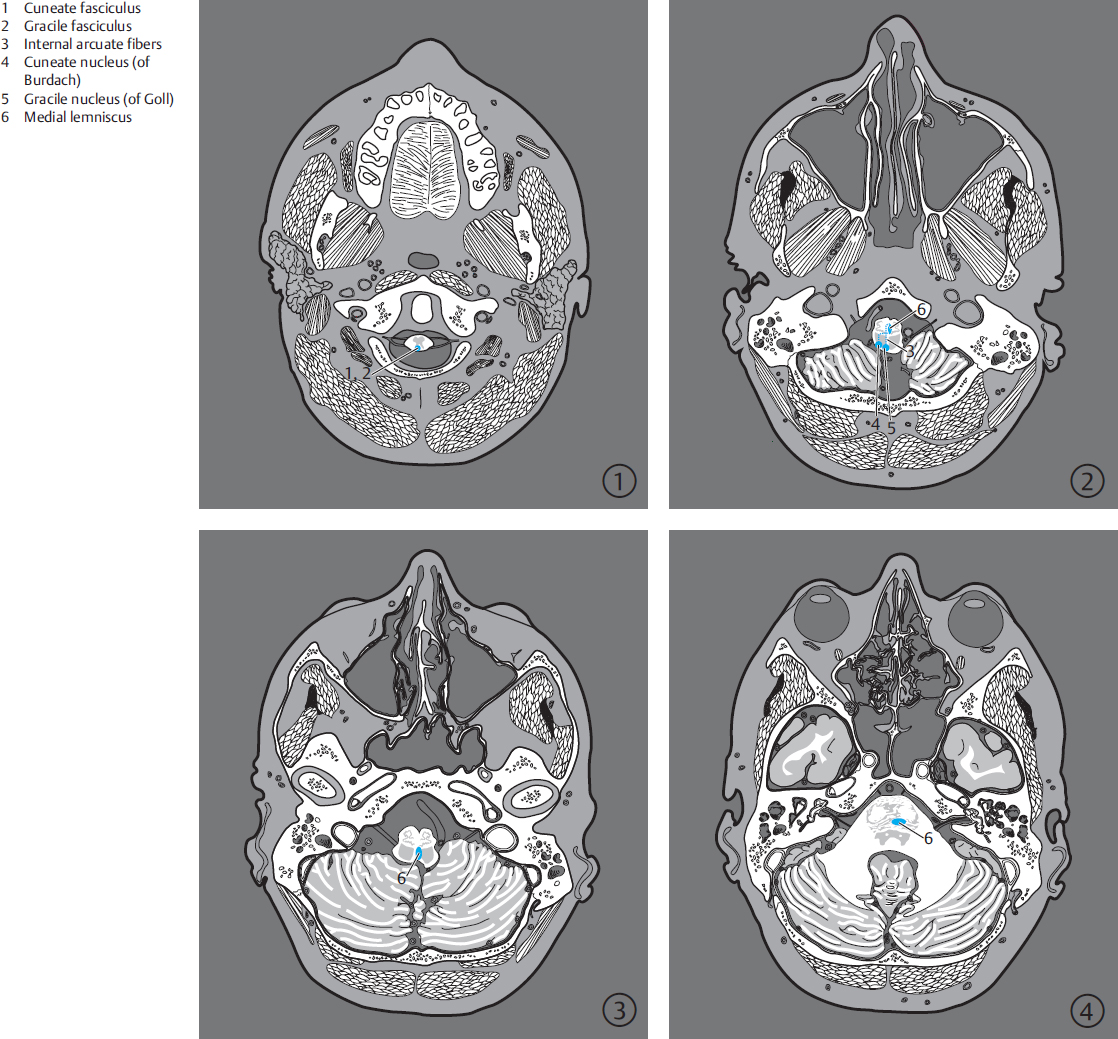
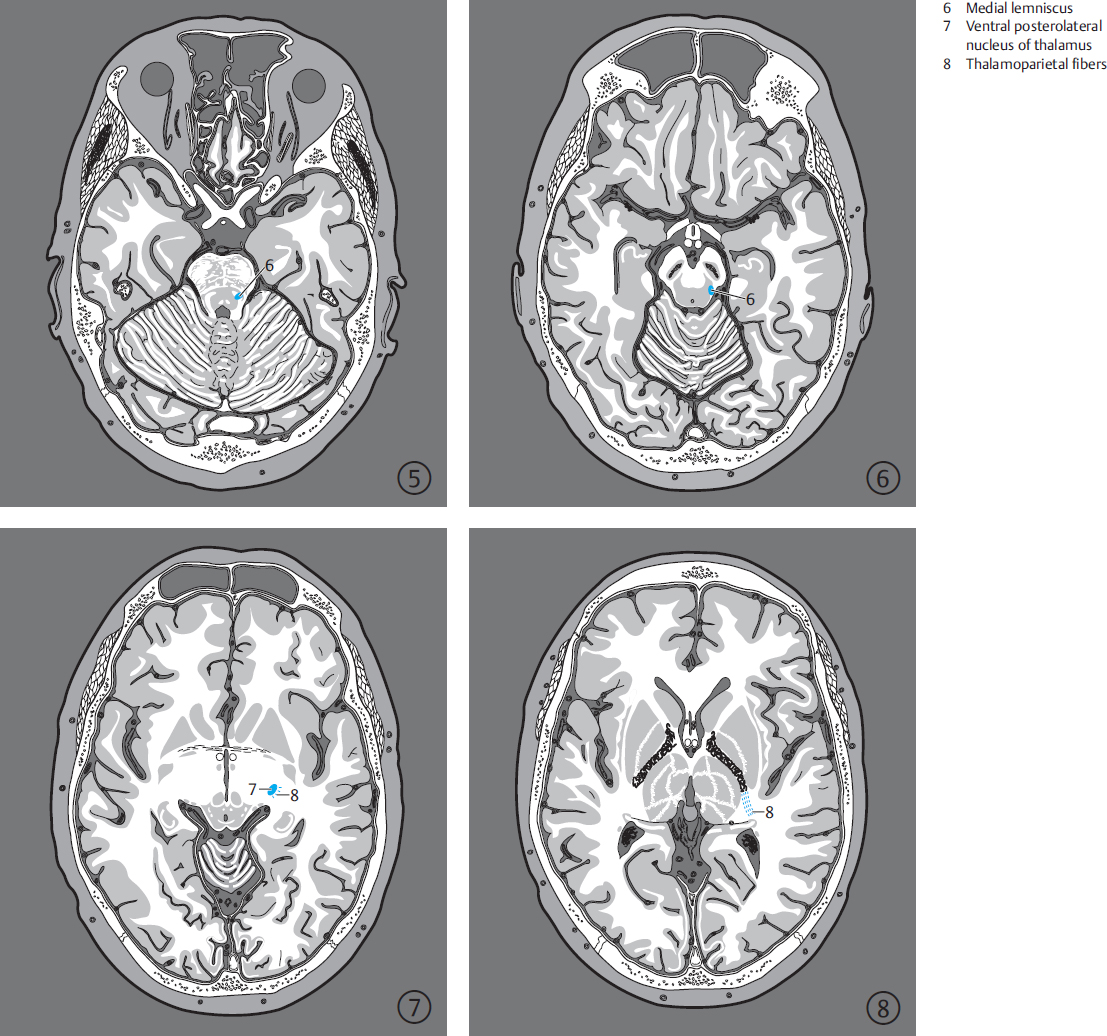
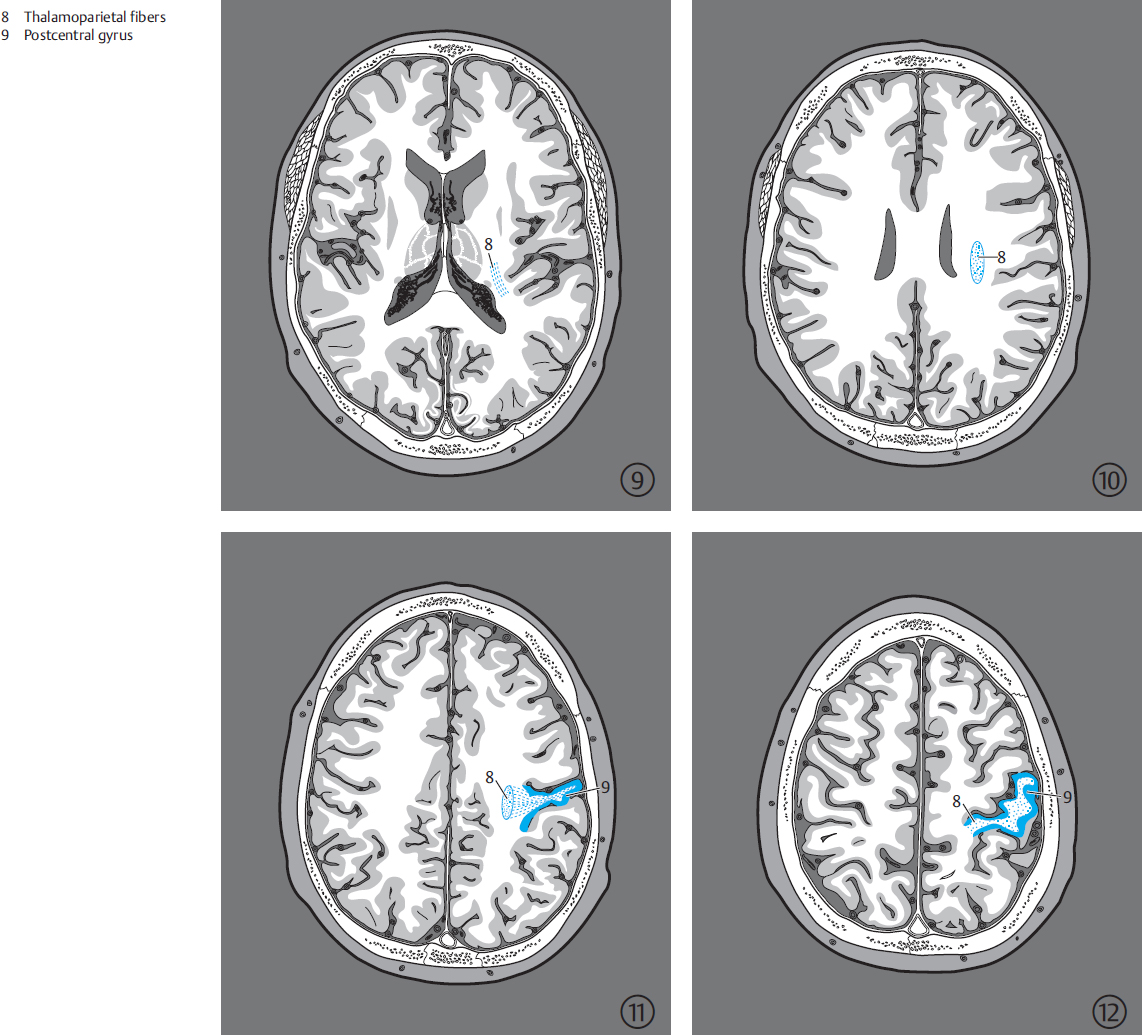
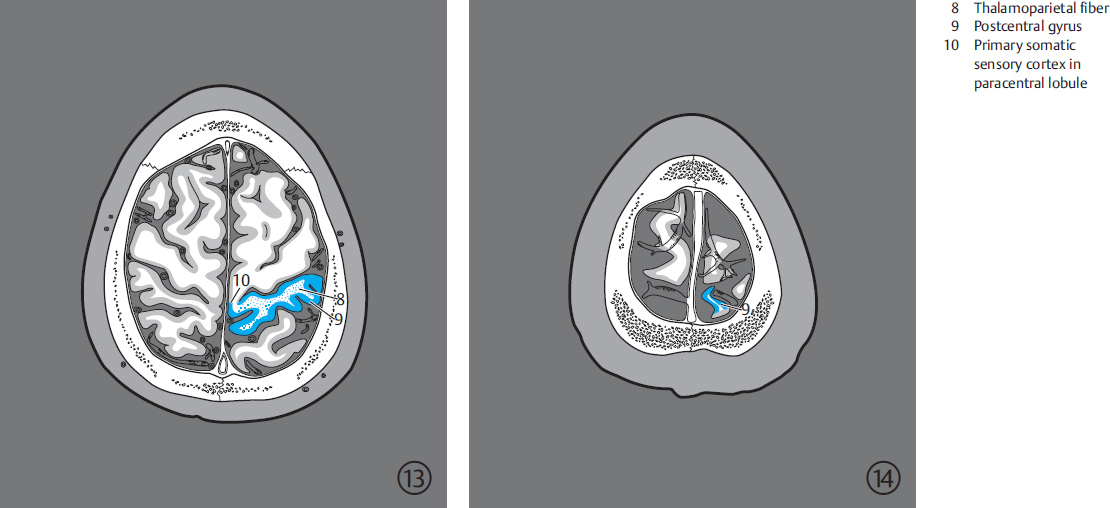
The gracile fasciculus terminates in the gracile nucleus in the medulla oblongata (Goll; see ▶Fig. 6.4b and ▶Fig. 6.4c) while the cuneate fasciculus ends in the cuneate nucleus (Burdach; see ▶Fig. 6.4b and ▶Fig. 6.4c). The gracile and cuneate nuclei are grouped together as “dorsal column nuclei” in accordance with their position at the upper end of the dorsal column of the spinal cord. They lie in the inferior region of the medulla oblongata at its junction with the spinal cord and their inferior ends lie at the level of the crossing-over of pyramidal tracts.
These nuclei form two macroscopically visible protrusions together with their fascicles on the posterior surface of the medulla oblongata, medially the gracile tubercle (see ▶Fig. 6.4c) and laterally, the cuneate tubercle (see ▶Fig. 6.4c). Axons of these second-order neurons extend in an arcuate direction to the opposite side from nerve cells of the gracile and cuneate nuclei, and then run upward as the medial lemniscus close to the median plane of the medulla oblongata (see ▶Fig. 6.5c). The medial lemnisci appear as two bands abutting each other in the median plane in a cross-section of the medulla oblongata. In the pons, the medial lemniscus lies close to the anterior margin of the tegmentum of the pons (see ▶Fig. 6.9c) while it lies at the lateral aspect of the tegmentum in the midbrain (see ▶Fig. 6.12c). The medial lemniscus moves from medial to lateral at the junction of the midbrain and diencephalon and terminates in the ventral posterolateral nuclei of the thalamus. It may be identified through its shape and its contrast to surrounding structures on axial T2-weighted MR images.70 , 130
The posterior border of the ventral posterolateral nucleus where it lies adjacent to the pulvinar is demarcated by a nearly triangular, fiber-containing area (of Wernicke).229 , 514 It forms a groove inferiorly at the lateral aspect of the thalamus. The ventral posterolateral nucleus may be identified on axial MR images with the posterior limb of the internal capsule, the pulvinar, and to some extent the triangular area (of Wernicke)404 serving as landmarks.511 , 608 Third-order neurons project from the ventral posterolateral nucleus as the thalamoparietal tract to the postcentral gyrus and paracentral lobule.
Thalamoparietal fibers cannot be definitively identified either macro- or microscopically in specimens of adult human brains. They have therefore been demarcated in comparison with histological sections of infant brains.463 , 608 These fibers course diagonally upward in a lateral direction through the posterior limb of the internal capsule, posterior to the corticospinal tract. Superior to the optic radiation, they run at the lateral edge of the corona radiata to the postcentral gyrus and the paracentral lobule. The anterior border of the primary somatosensory cortex in the postcentral gyrus is formed by the floor of the central sulcus, even though the somatosensory and motor regions seldom meet exactly at the floor of the sulcus.463 The floor of the postcentral sulcus has therefore been defined as its posterior boundary. Inferiorly, in the region of the postcentral gyrus, the primary somatosensory cortex of the medial lemniscus system cannot be morphologically demarcated from the primary sensory cortex of the trigeminal system. This boundary has thus been demarcated based on physiological–experimental descriptions.630 The primary so mato senso ry cortex of neurosurgical patients was stimulated under local anesthesia by means of electrodes applied directly to the cerebral surface. Stimulation produced parasthesiae in specific parts of the body as described by the patients. In accordance with these investigations,630 the distance from the superior cerebral margin to the lateral sulcus in the region of the postcentral gyrus was calculated in a similar fashion to a measurement using a tape measure.608 The boundary between representative areas of the medial lemniscus system and the trigeminal system was determined by selecting a section of the brain lying just below the upper 70% of the determined measurement. The central sulcus may be used as an orienting point to identify the postcentral gyrus on MR images since it is visualized on both sagittal as well as on axial planes (see ▶Fig. 7.52a).231 , 511 , 602
The primary somatosensory cortex on the medial side of the hemisphere in the paracentral lobule is a triangular structure, similar to that depicted in Brodmann’s illustrations76 (see ▶Fig. 7.53). The terminal end of the central sulcus perpendicular to inferior sections in the direction of the cingulate sulcus is transposed as the anterior border; the inferior end of this triangular cortical area is assumed to be represented by the boundary between the upper two-thirds and the inferior thirds of this connecting line. The marginal branch of the cingulate sulcus helps identify the paracentral lobule in axial MR images, which is usually seen on the medial aspect as a furrow immediately posterior to the central sulcus.231 , 511
Somatotopic organization of the medial lemniscus system corresponds to that of the spinothalamic system (see Section 10.1.1). An interruption of the medial lemniscus system leads to an impaired perception of deep sensations (vibration and position) as well as some superficial sensations (disordered two-point discrimination).
10.1.3 Trigeminal System
Pain, cold, and heat receptors of skin of the face, as well as those of the mucous membranes of the nose and paranasal sinuses, the oral cavity and the teeth transmit their signals via branches of the trigeminal nerve (see ▶Fig. 10.6, ▶Fig. 10.7, and ▶Fig. 10.8) to the pseudounipolar nerve cells of the trigeminal (Gasserian) ganglion. The central axons of the trigeminal ganglion run to the pons, passing through it as the spinal tract of the trigeminal nerve to reach the caudal part of the spinal nucleus of the trigeminal nerve. This part of the nucleus lies at the lateral aspect of the medulla oblongata and extends from the obex down to the cervical segment of the spinal cord at C2. It corresponds to sensory neurons in the dorsal gray horn of the spinal cord, which also transmit pain and thermal signals. The lateral trigeminothalamic tract arises from second-order neurons at the caudal aspect of the spinal nucleus of the trigeminal nerve, and passing upward, it crosses in the medulla oblongata. It then passes together with the spinothalamic tract to the thalamus and thereafter to the postcentral gyrus. The primary somatosensory fields for these pathways lie at the base of the postcentral gyrus in the vicinity of the lateral sulcus. The suboral nucleus (approximate length 14 mm) of the spinal nucleus of the trigeminal nerve lies in the lower part of the pons while its interpolar part (approximate length 11 mm) lies in the medulla oblongata6 and transmits pain sensations from the teeth.199 , 613
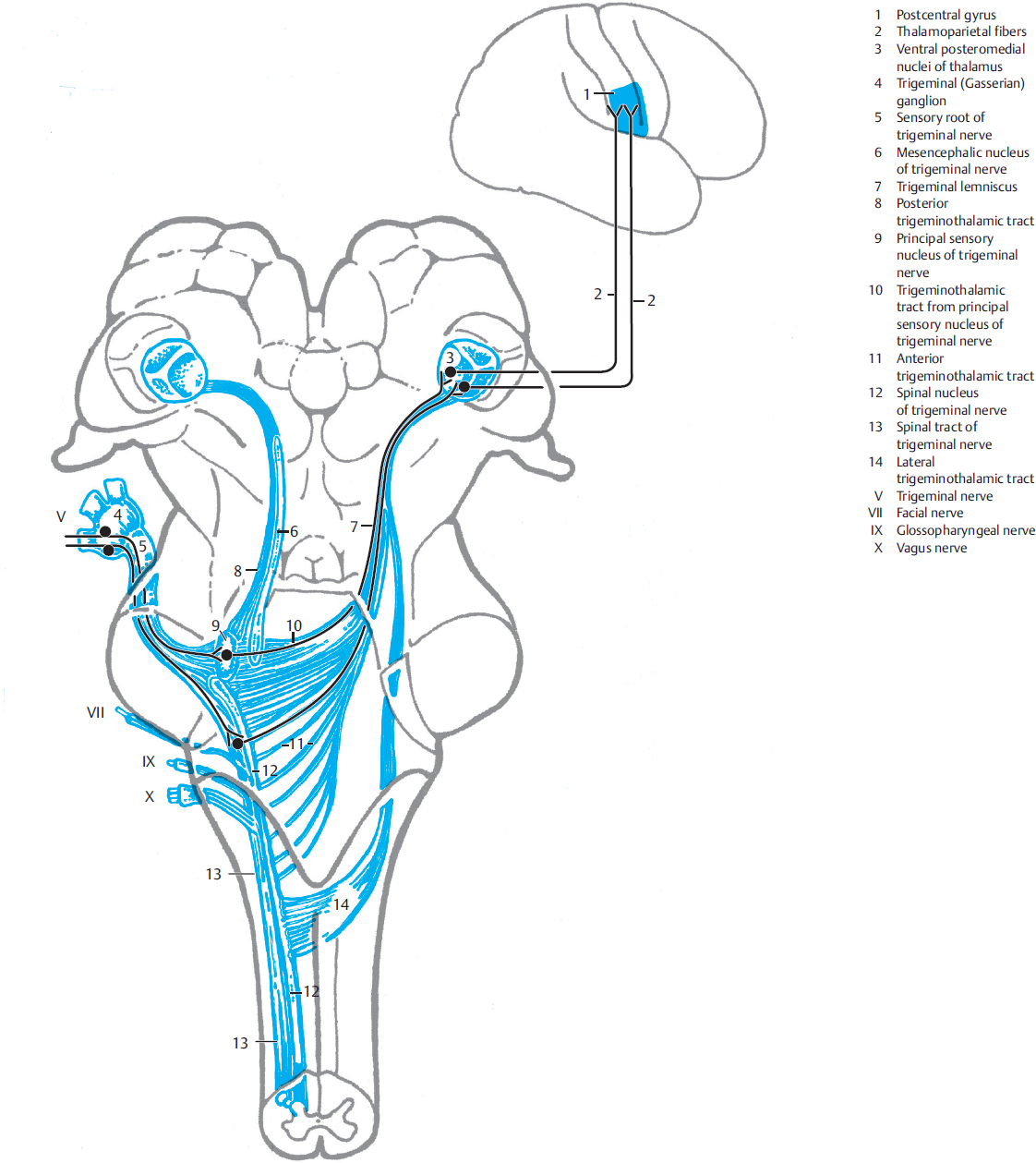
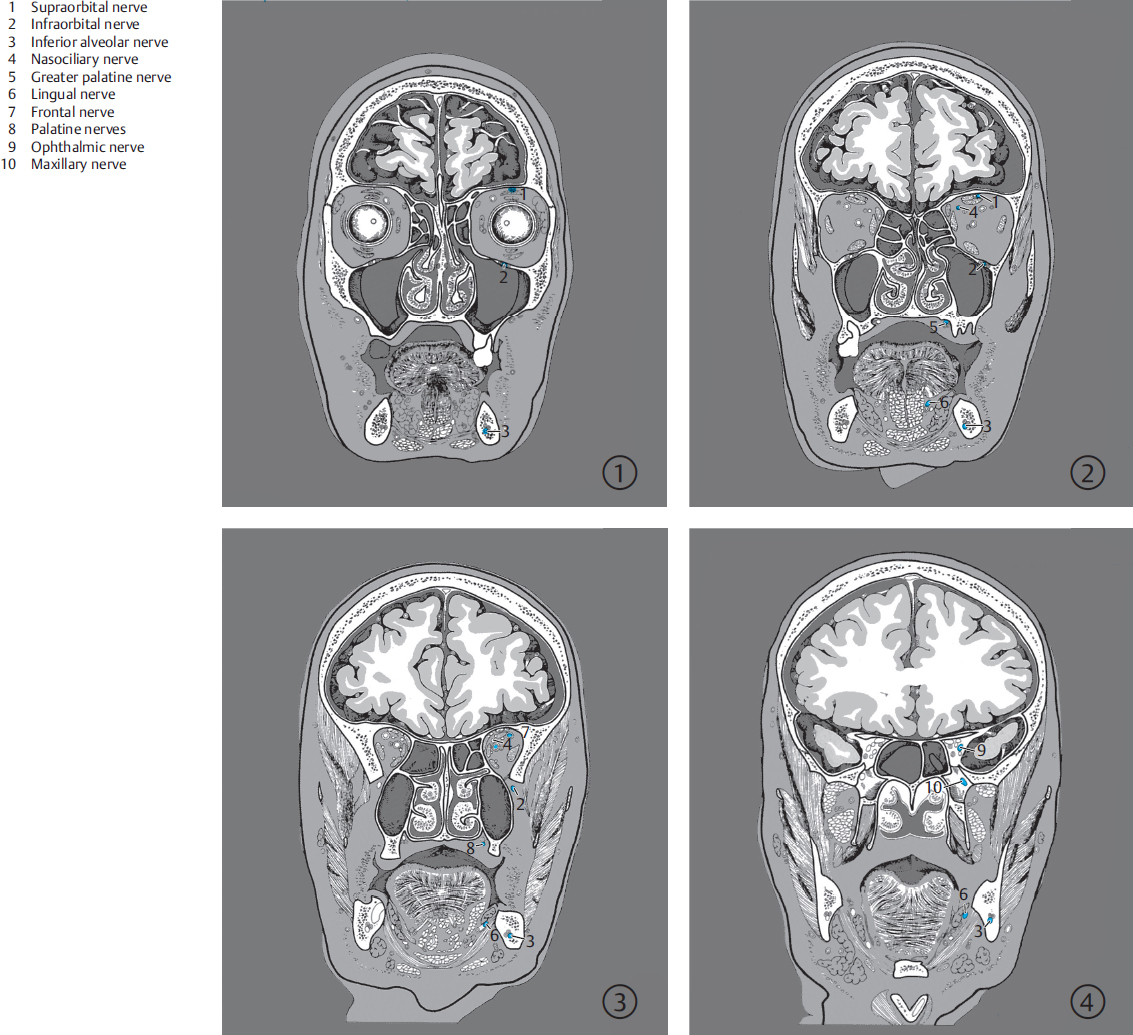
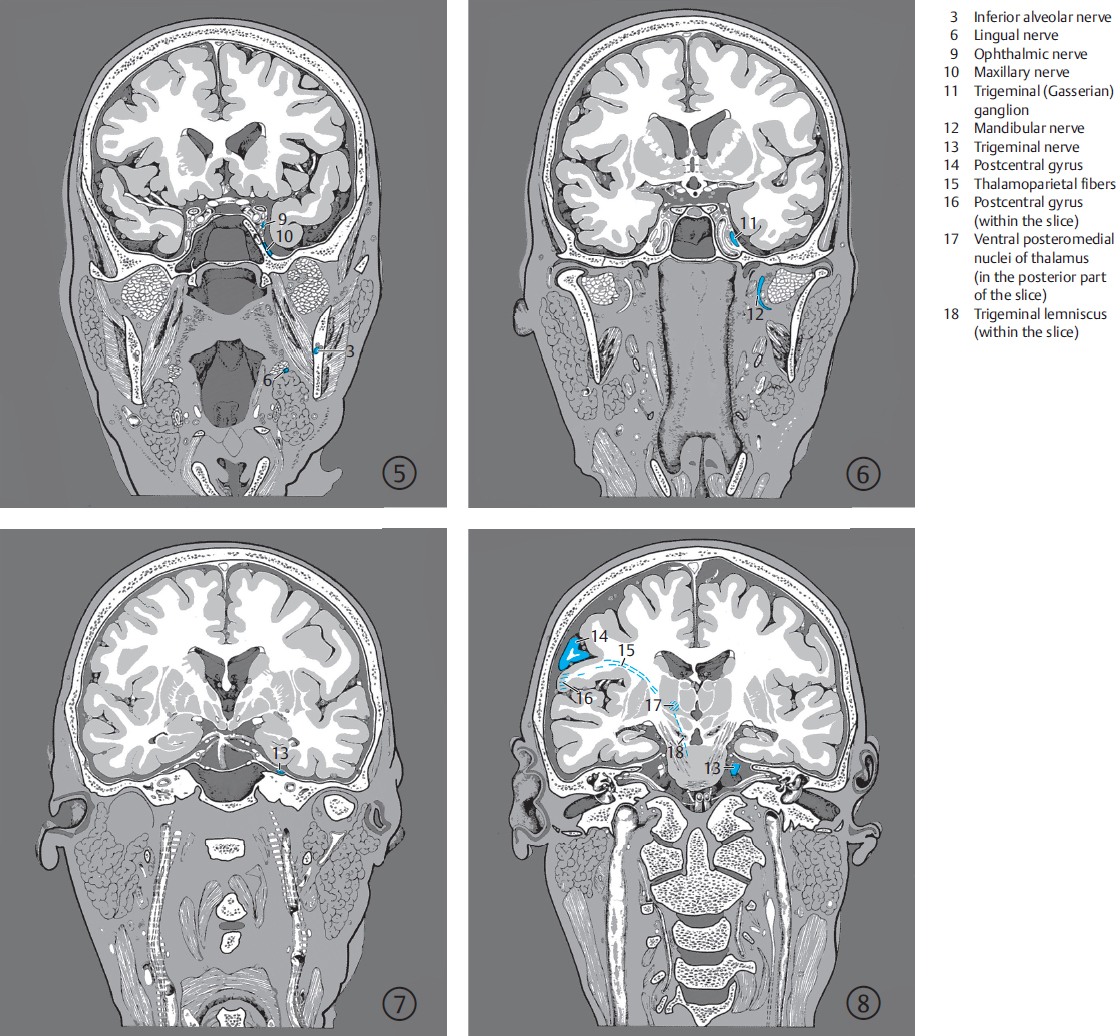
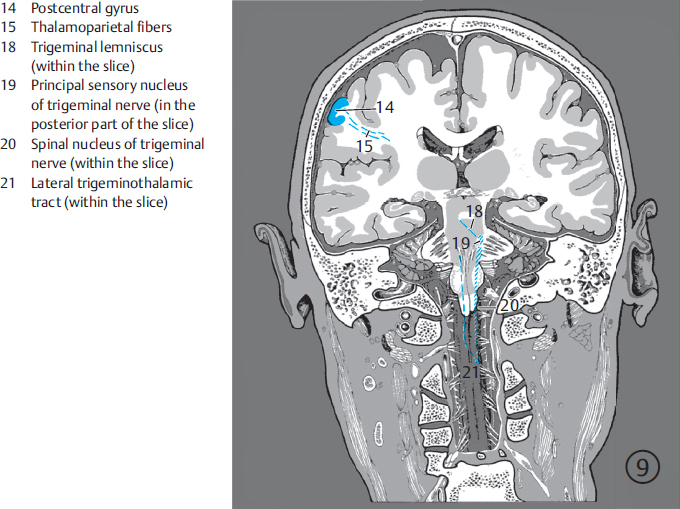
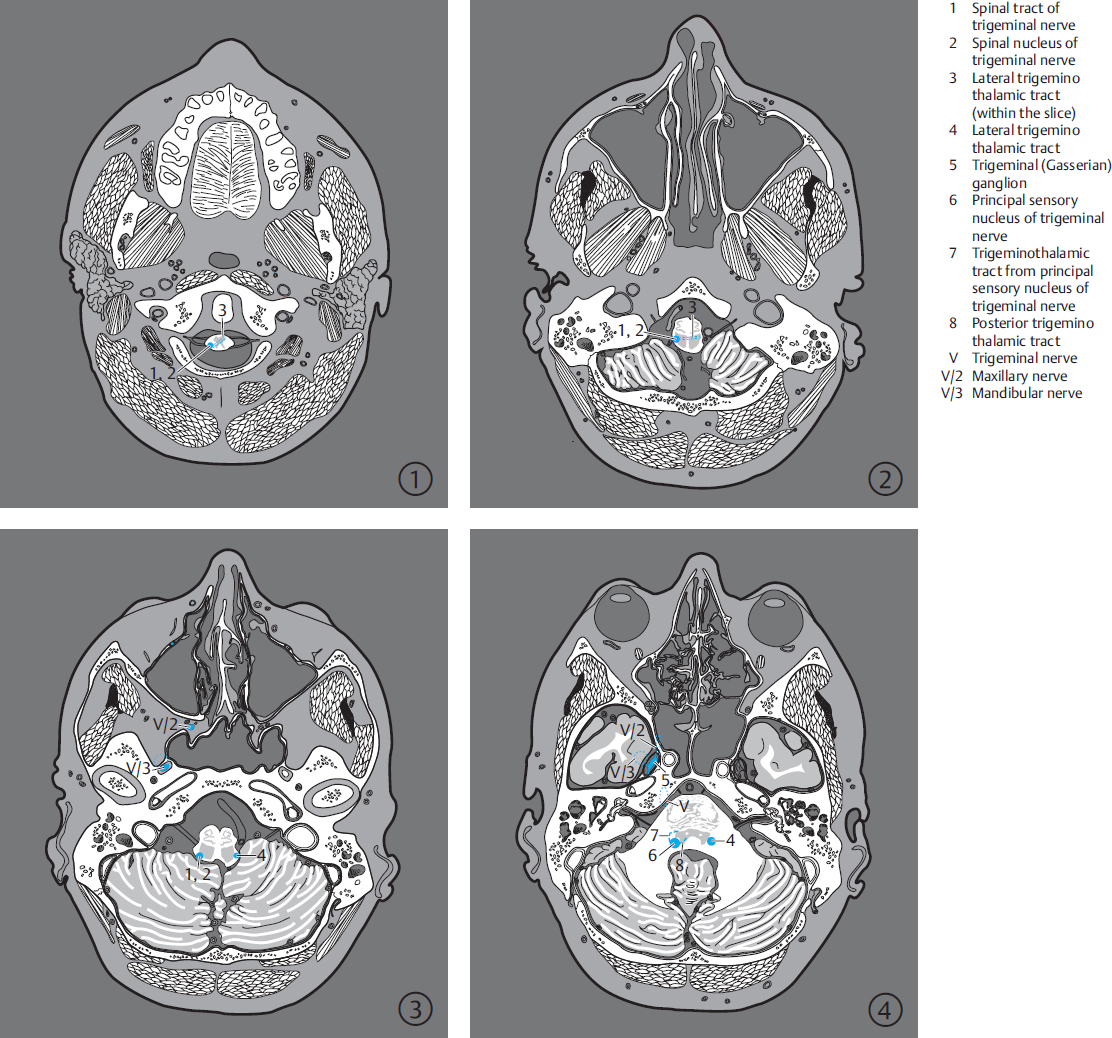
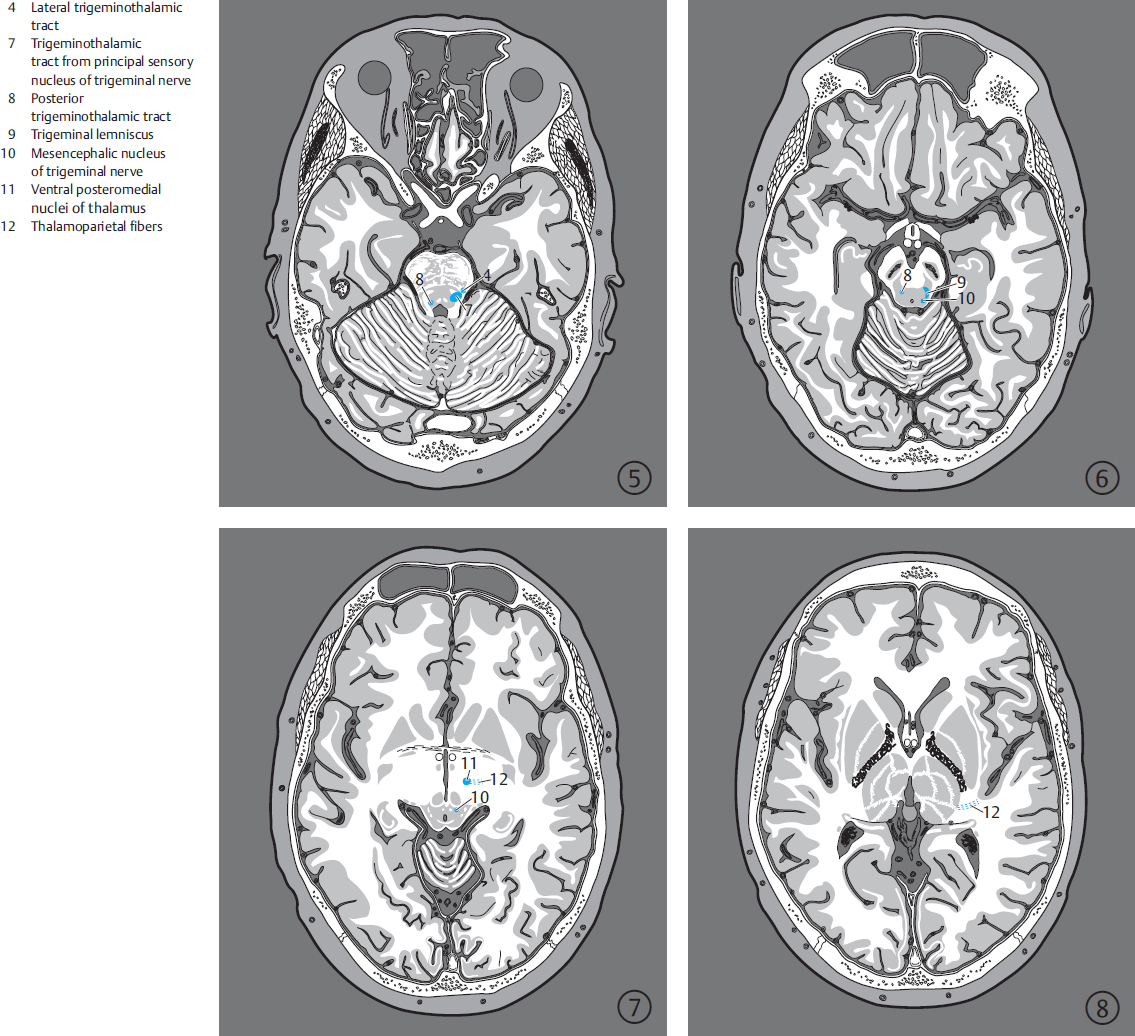
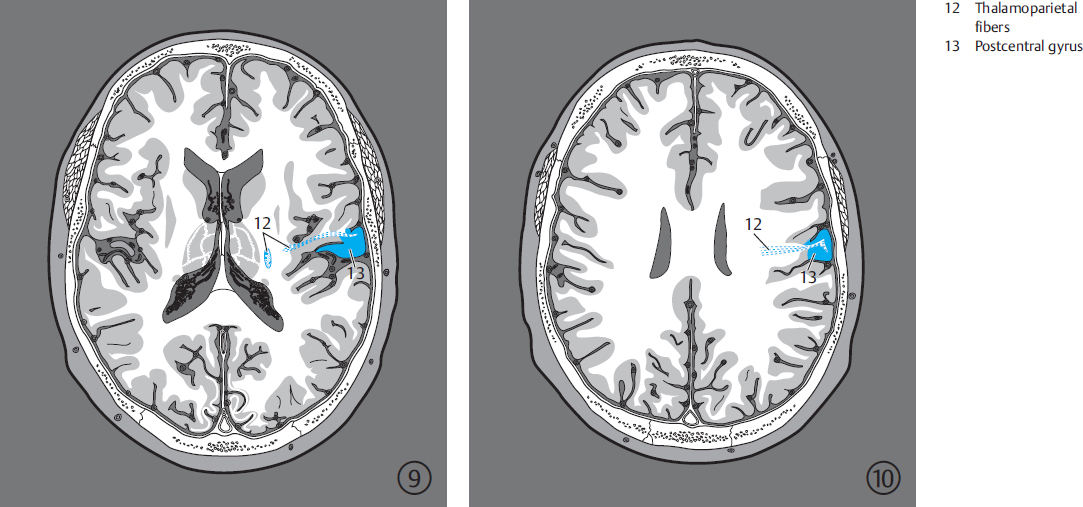
The mechanoreceptors of the skin of the face, eyes and the nasal and oral cavities transmit their signals via branches of the trigeminal nerve to pseudounipolar nerve cells of the trigeminal ganglion. The central axons of this ganglion extend as the sensory root (portio major) of the trigeminal nerve, mainly to the principal sensory nucleus of the trigeminal nerve. This nucleus was earlier known as the “pontine nucleus of the trigeminal nerve” and was renamed, since the oral part of the spinal nucleus of the trigeminal nucleus also lies in the pons. The principal sensory nucleus of the trigeminal nerve lies in the lateral part of the tegmentum of the pons at the same level as the entrance of the trigeminal nerve. Axons of the second-order neurons from the principal sensory nucleus cross over to the opposite side and continue as trigeminothalamic fibers to the ventral posteromedial nuclei of the thalamus. Third-order neurons arise as thalamoparietal fibers from this nucleus and pass to the lower third of the postcentral gyrus. These pathways carry superficial and depth sensations, except for pain and temperature. Uncrossed fibers coursing from the principal sensory nucleus to the ventral posteromedial nucleus are known as the Wallenberg tract or the posterior trigeminothalamic tract,527 which courses further posteriorly in the tegmentum of the pons and the midbrain than the main pathway from the principal sensory nucleus. The ipsilateral and contralateral tracts lie directly next to one another just short of the ventral posteromedial nucleus.
▶Fig. 10.6 depicts the lateral trigeminothalamic tract (pain and temperature signals), the anterior trigeminothalamic tract (pain and temperature signals), and the trigeminothalamic tract from the principal sensory nucleus (mechanosensibility) running close to each other at the level of the pontomesencephalic junction before they simultaneously reach the ventral posteromedial nucleus. This trigeminal lemniscus runs so close to the medial lemniscus in the upper part of the pons and the midbrain such that these two fiber pathways may only be differentiated histologically after experimental marking in mammalian brains. Histological findings reveal that the fibers of the trigeminal lemniscus lie posterior to those of the medial lemniscus within this common fiber bundle.
Muscle spindle afferents of masticatory muscles pass through pseudounipolar nerve cells that lie not in the trigeminal ganglion but in the mesencephalic nucleus of the trigeminal nerve, which extends from the lateral aspect of the floor of the rhomboid fossa of the pons to the central periaqueductal gray matter of the midbrain. Central axons of pseudounipolar nerve cells connect with the motor nucleus of the trigeminal nerve, thus providing a monosynaptic pathway for the masseter reflex.
10.1.4 Clinical Significance
The effects of lesions on individual sensory systems have been summarized as follows:
Clinical Notes
The separate course of the anterolateral and medial lemniscus systems in the medulla oblongata explains the appearance of a dissociated sensory disorder due to an isolated lesion of the anterolateral system in the medulla oblongata. These are often an outcome of vascular insults, which cause a contralateral disturbance of pain and sensation. Additional lesions of first-order sensory neurons and/or of the initial part of second-order trigeminal neurons may cause ipsilateral sensory impairment involving the face. This gives rise to crossed sensory impairment, such as that of Wallenberg’s syndrome, caused by lesions in the lateral medulla oblongata. usually cause sensory disorders involving the entire contralateral side of the body due to the compact bundling of all sensory systems in this region. Increasing somatotopic fanning out of thalamocortical pathways in the centrum semiovale up to the sensory cortex results in isolated sensory disorders of individual (contralateral) parts of the body, which then include the entire range of sensations. Records of somatosensory evoked potentials (SEP) enable objective measurements of functional disturbances of both peripheral and central sensory systems. Repeated electrical stimulation of a nerve allows topical localization if typical SEP patterns are obtained using surface electrodes over the spinal cord, brainstem, and the primary cortical somatosensory field.366 , 521 , 564
A lesion of the medial lemniscus impairs tactile discrimination, that is, sensations of touch, position, and vibration sense. Lesions close to the midline of the medulla oblongata may result in uni or bilateral impairment of this sensory system. Dissociated sensory disorders above the pons are rare due to the proximity of both sensory systems. The same is true for the trigeminal system. Isolated sensory loss, such as those of pain and temperature sensations, appears only as an outcome of injuries of the upper cervical spinal cord and/or the medulla oblongata.
Lesions in the posterior region of the internal capsule
10.2 Gustatory System
The facial, glossopharyngeal, and vagus nerves carry gustatory signals from taste buds and free nerve endings to the medulla oblongata (see ▶Fig. 10.9 and ▶Fig. 10.10). The perikarya or cell bodies of the first-order neurons lie within the geniculate ganglion and the superior and inferior ganglia of the IXth and Xth cranial nerves. Their central axons terminate in the gustatory part of the solitary nucleus and in its superior extension, the nucleus ovalis. The pathway then ascends, coursing in a similar fashion as the trigeminal system close to the medial lemniscus and reaches a thalamic subnucleus. Third-order neurons project from here to the parietal operculum and to an area at the edge of the insula.48 , 88
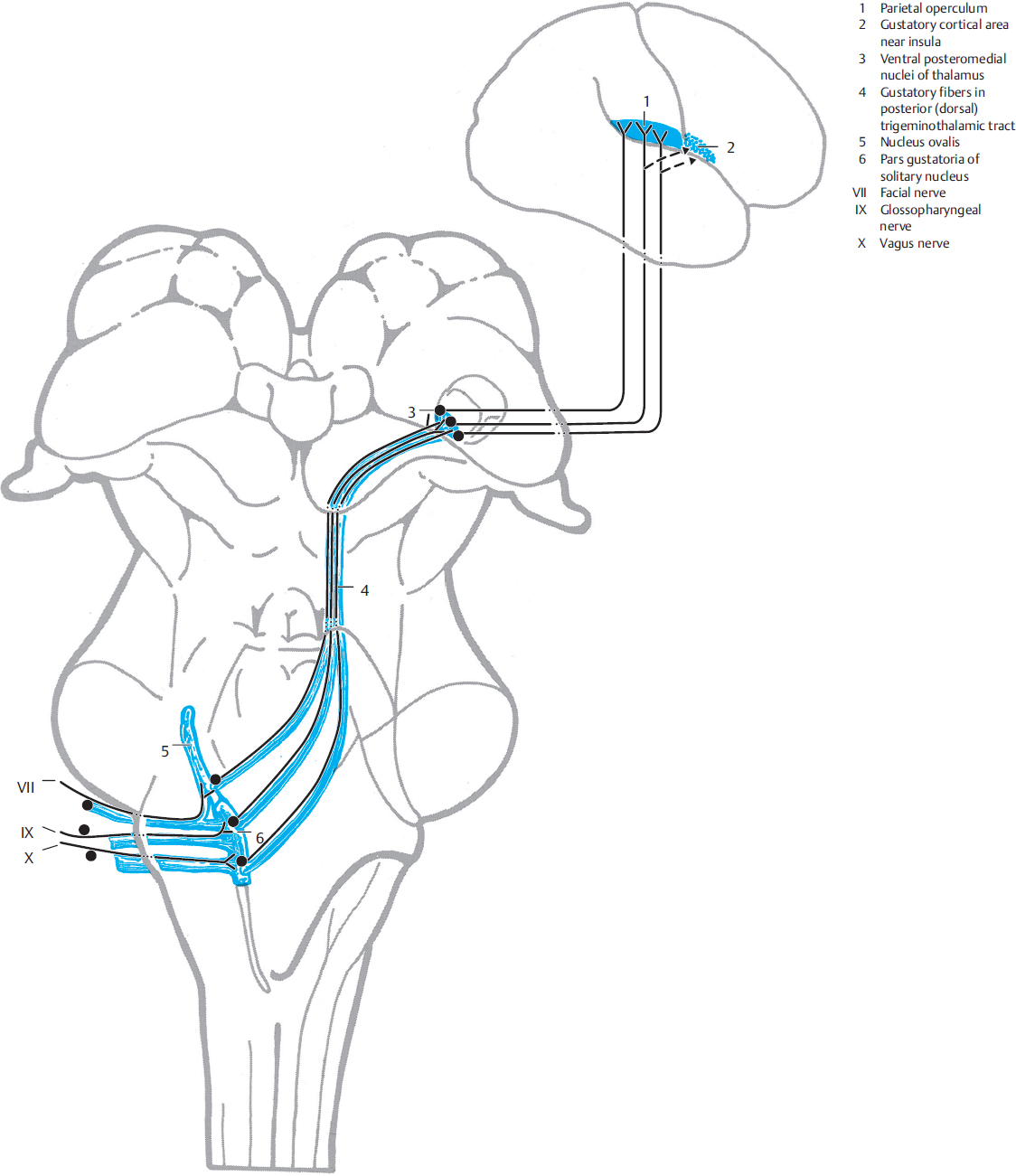
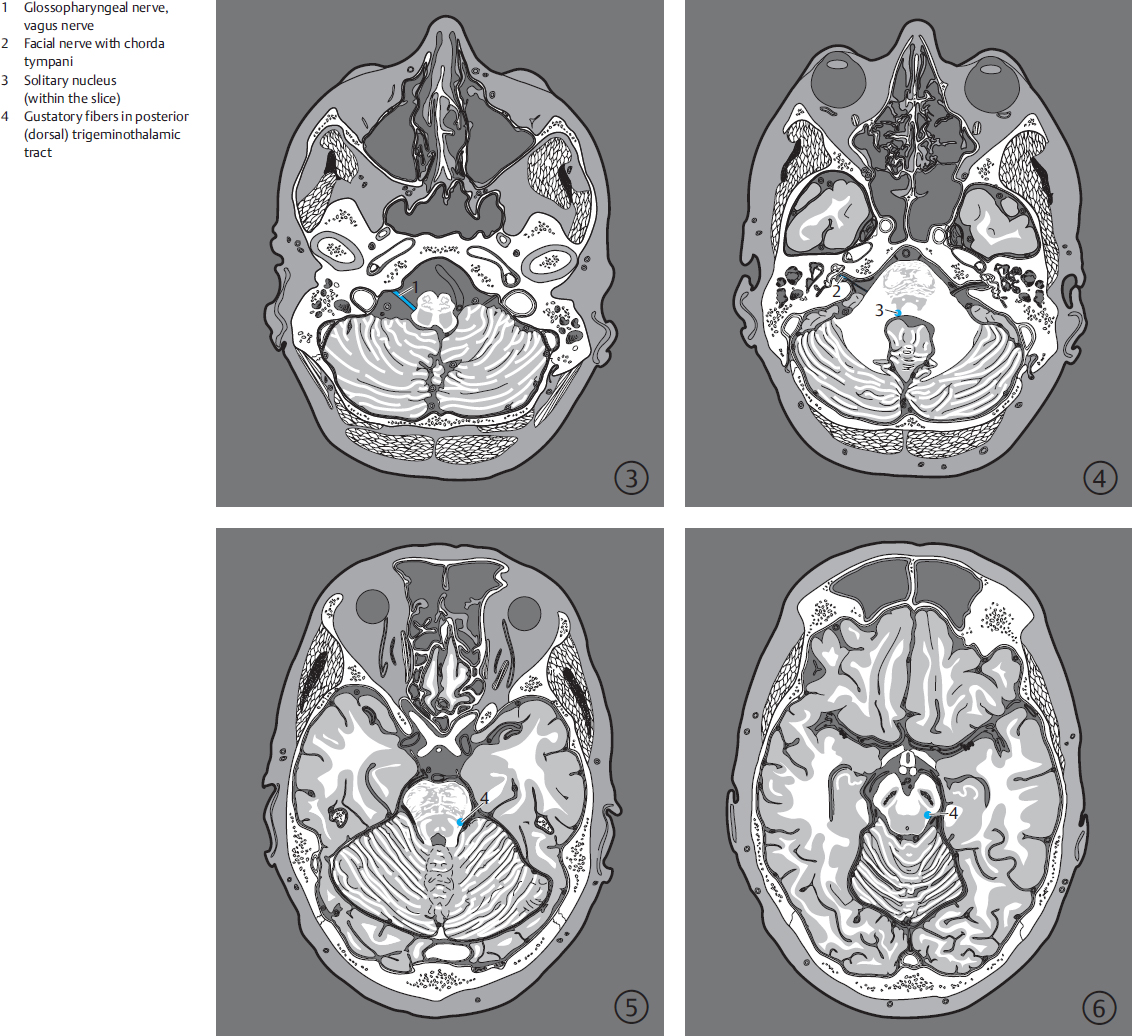
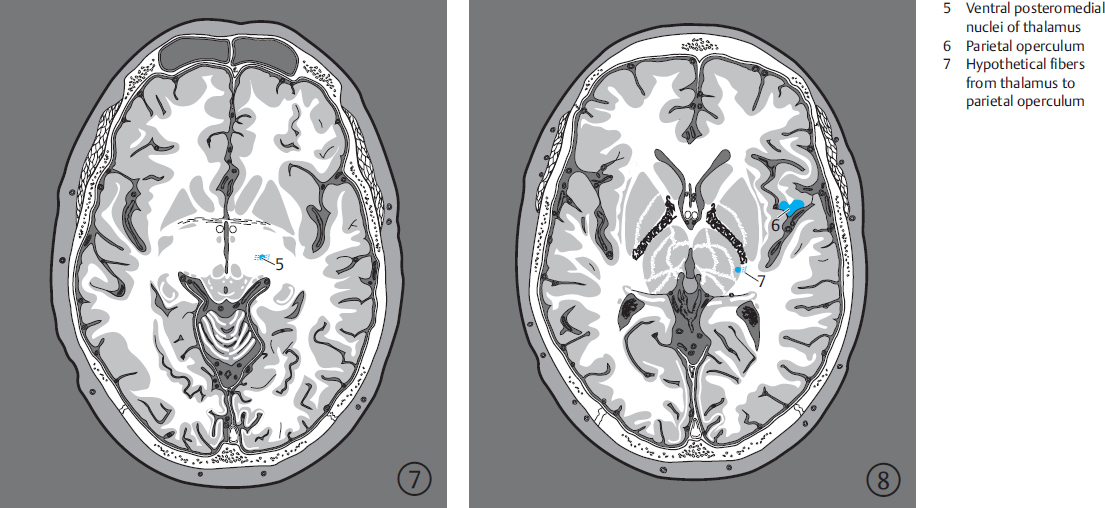
Clinical Notes
Impaired taste sensations are predominantly accounted for by peripheral lesions of the taste buds or lesions of the VIIth, IXth, and/or Xth cranial nerves and not by lesions in the gustatory nuclei and cortical areas.
10.3 Ascending Reticular System
The reticular formation (see ▶Fig. 6.12b and ▶Fig. 10.1) consists of a network of organized nerve cells in the central zone of the tegmentum of the medulla oblongata, pons and midbrain and is surrounded by cranial nerve nuclei, several relay nuclei, and descending fiber tracts.
The medial lemniscus system passes through the reticular formation469; the latter receives afferent signals from the spinal cord and from all sensory cranial nerves which are then relayed through intralaminar nuclei of the thalamus via widely dispersed projections to the cerebral cortex. The reticular formation forms a nonspecific system of neurons between receptors and cortical nerve cells due to polysynaptic impulse propagation and a well-developed network. Specific processing systems, in contrast, exhibit a point-to-point relay between the signal-producing receptors and nerve cells in the primary regions of the cerebrum. Examples of such systems include the medial lemniscus system and the visual pathway. The ascending reticular system also projects into numerous subcortical centers, including the striatum, preoptic region, septal nuclei, and hypothalamic regions65 , 424 and is closely related to the descending reticular system.75
Clinical Notes
The complicated interconnections of the reticular formation with its numerous connections with motor, limbic, and several other systems explains the difficulty of analyzing isolated functional disorders of this complex system. Ascending fibers emerging from the reticular formation have an activating effect on the forebrain. Damage to the reticular system may result in altered attentiveness, impaired consciousness as well as unconsciousness.255 , 651
10.4 Vestibular System
Sensory receptors of the vestibular system (see ▶Fig. 10.11, ▶Fig. 10.12, and ▶Fig. 10.13) lie in the semicircular canals as well as within the saccule and utricle. Sensory cells of the semicircular canals monitor angular acceleration of the head while sensory cells within the saccule and utricle monitor the effect of linear acceleration via small calcium carbonate crystals embedded in a gelatinous matrix. Information pertaining to linear acceleration in the gravitational field of the earth is thus relayed to the central nervous system.93
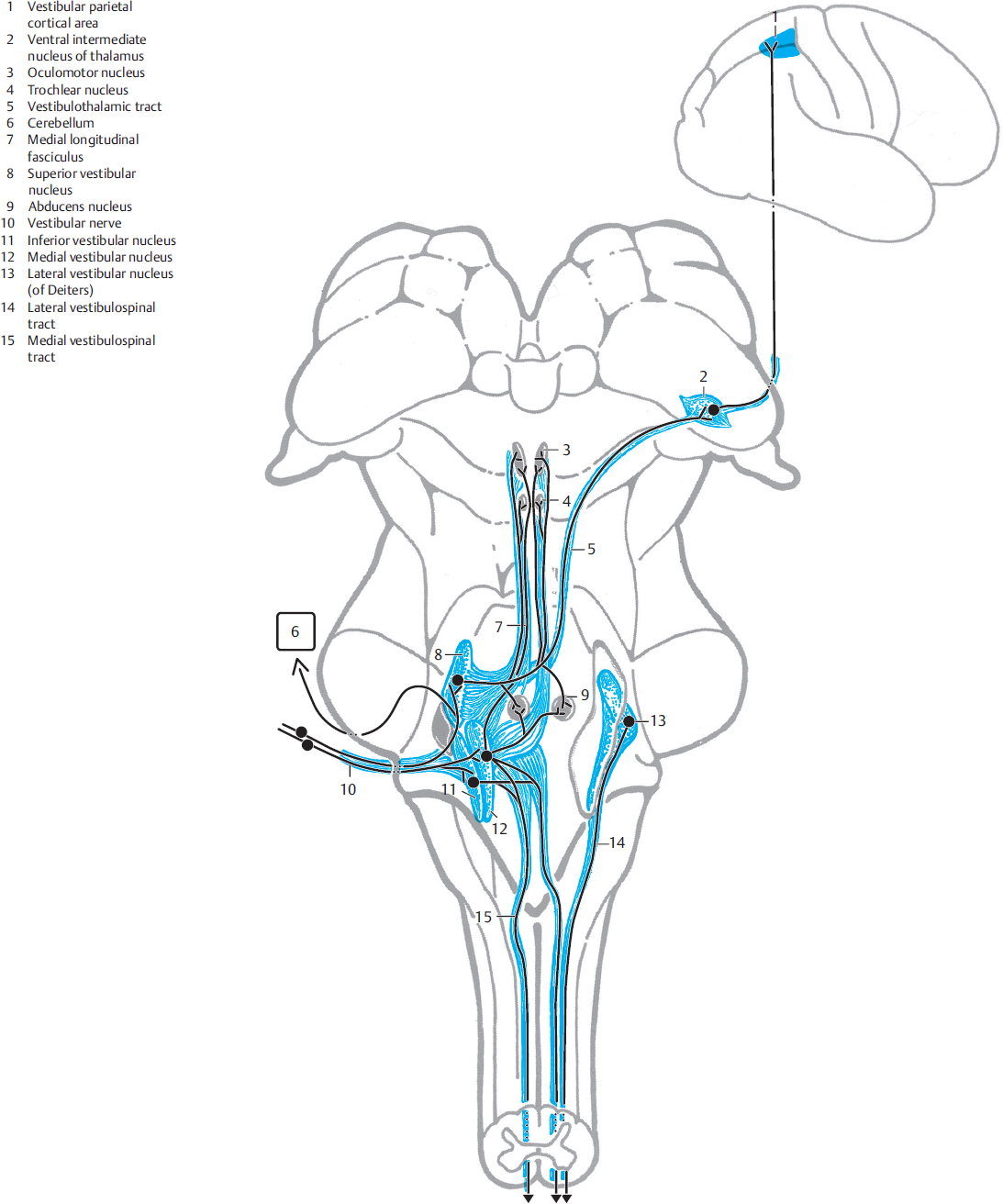
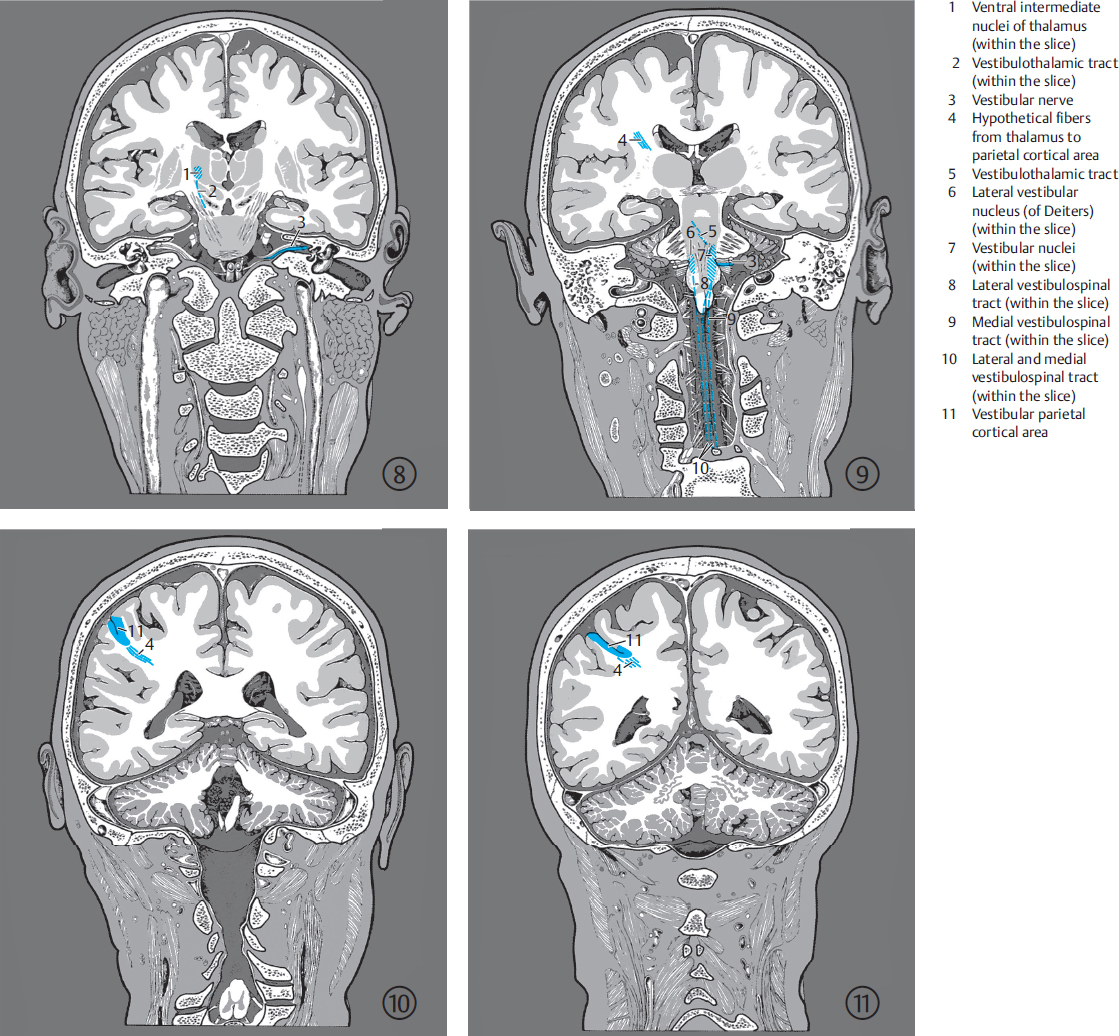
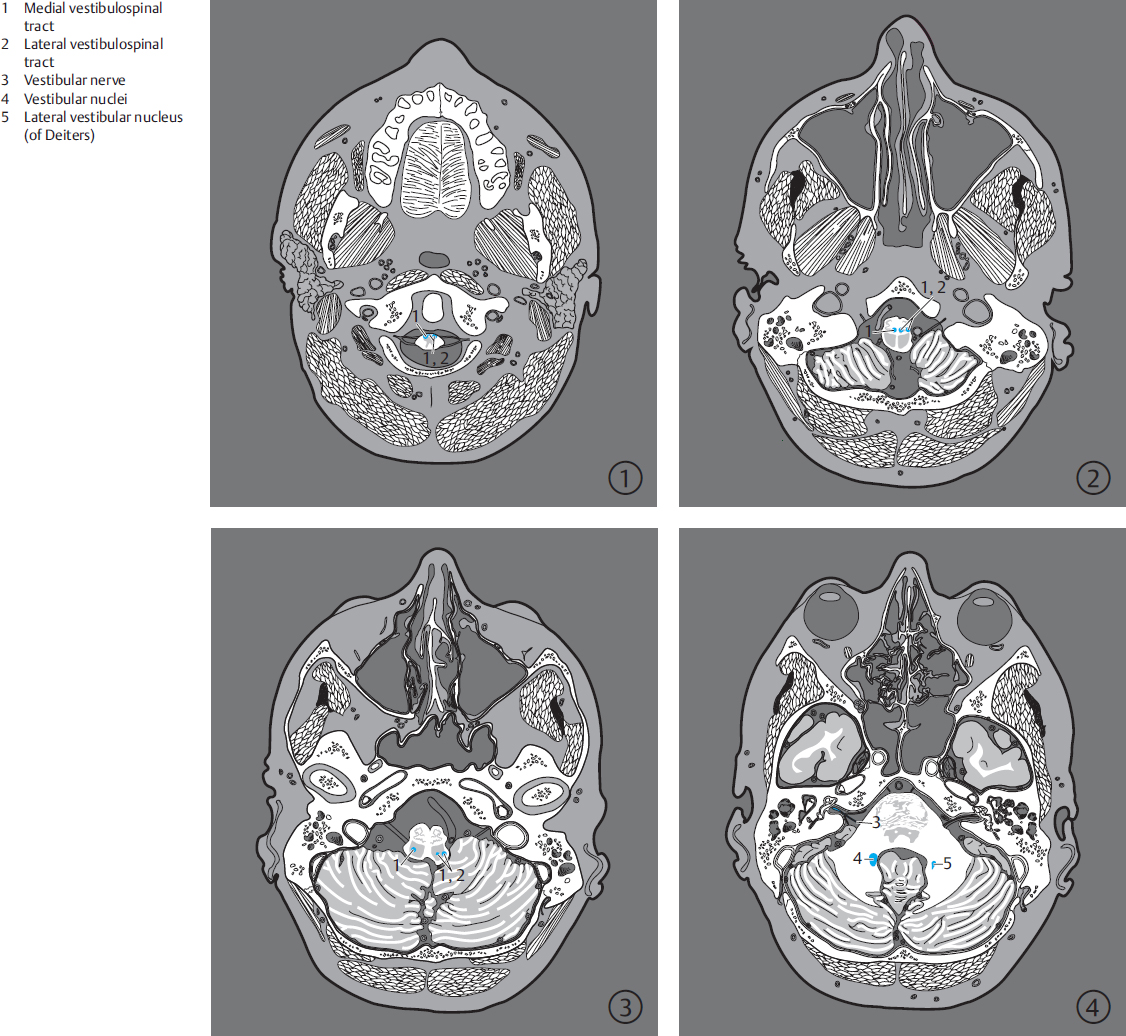
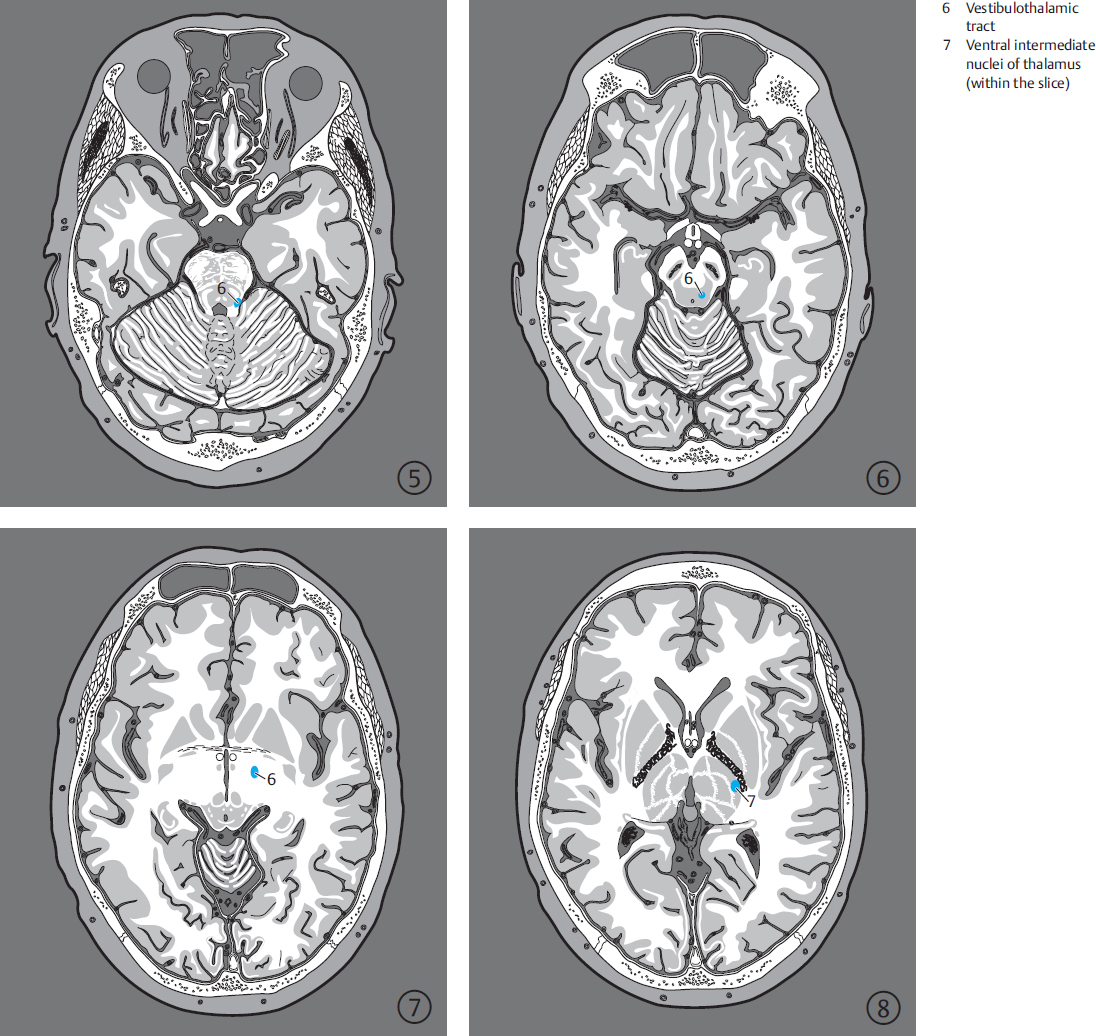
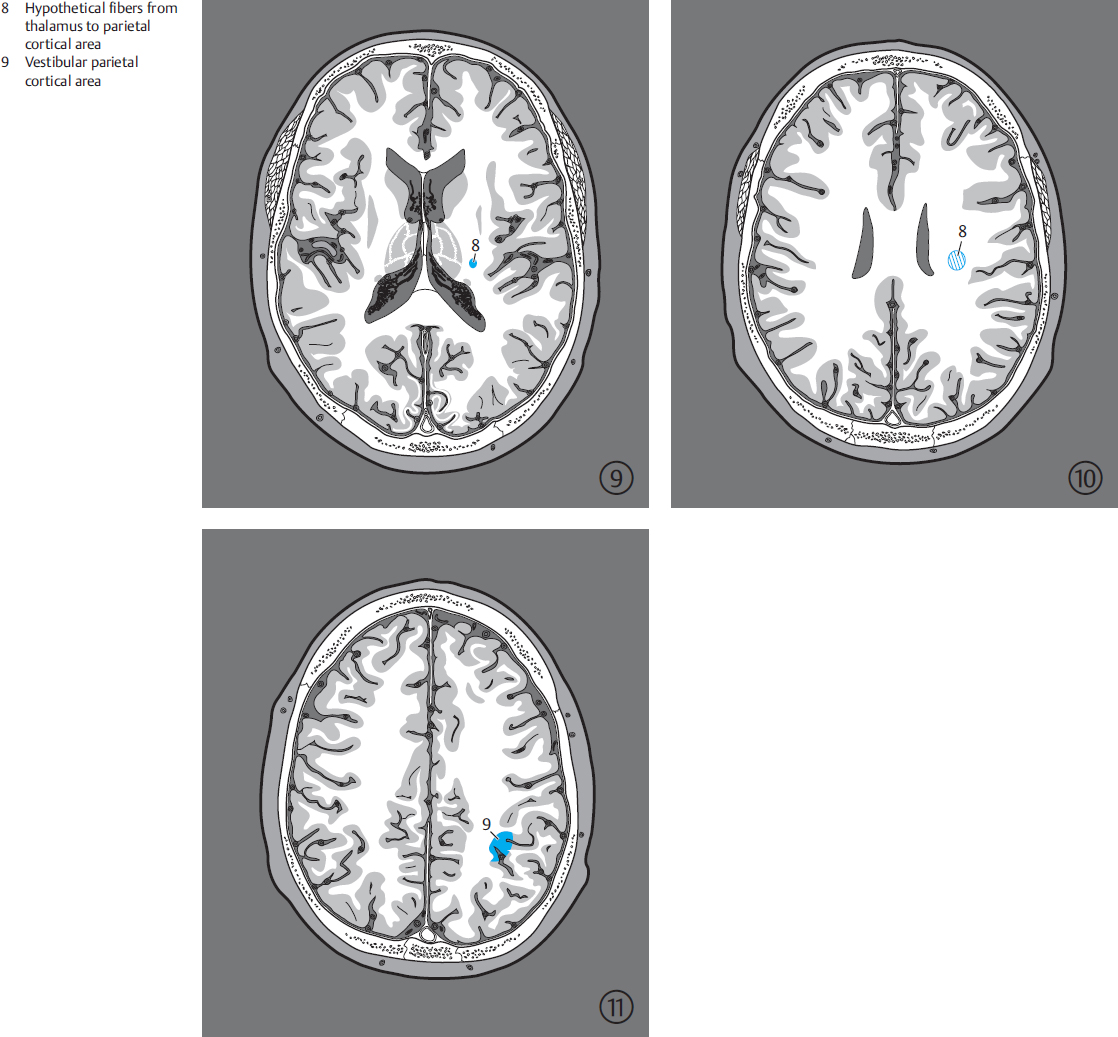
The signals of angular and linear acceleration are transmitted by the neurons of the vestibular system. Cell bodies of the first-order vestibular neurons lie in the vestibular ganglion in the internal acoustic canal. The peripheral axons of these bipolar cells ramify in the sensory cells of the semicircular canals and those of the saccule and utricle. The central axons form the vestibular component of the VIIIth cranial nerve which enters the brainstem at the cerebellopontine angle. Fibers from sensory cells of the semicircular canals terminate primarily in the superior and medial vestibular nuclei while some extend directly to the flocculonodular lobe of the cerebellum. These fibers, with synaptic connections with sensory cells in the saccule and the utricle then preferentially run to the inferior vestibular nucleus. Only a few of the primary vestibular afferent fibers terminate in the large-celled lateral vestibular nucleus (of Deiters).
Vestibular nuclei receive afferent signals from the spinal cord, reticular formation and cerebellum. Efferent connections from the lateral vestibular nucleus descend in the lateral vestibulospinal tract to the spinal cord. Fibers from the remaining vestibular nuclei run in the medial longitudinal fasciculus to motor neurons in the brainstem for the extraocular and neck muscles and via the vestibulospinal tract to the spinal cord. These pathways form a compensatory system which stabilizes the position of the eyes and posture of the head in the presence of disturbing influences. Each movement of the head is followed by reflex movement of the eyes such that the viewed object forms a constant image on the retina, thereby ensuring visual orientation in space.
The vestibular system has numerous connections with the motor neurons of the eye, neck, trunk, arm, and leg muscles (vestibular reflexes). There are fewer pathways between the vestibular system and the cerebral cortex and these are probably relayed in the small contralateral ventral posterior nuclei of the thalamus424 and then in a parietal cortical area around the intraparietal sulcus. Another pathway runs via the ventral posterolateral nuclei of the thalamus to area 3 in the postcentral gyrus but has not been demonstrated in ▶Fig. 10.11, ▶Fig. 10.12, and ▶Fig. 10.13. Other small vestibular cortical areas (area 7, parieto-insular and insular cortical areas) have been demonstrated in monkeys.94
Clinical Notes
Vestibular system lesions result in a disturbance of equilibrium. Acute vestibular lesions initially cause vertigo. Unilateral lesions of the vestibular apparatus are compensated for after several weeks while unsteadiness of gait persists with bilateral lesions.149 , 387 , 407 , 408
“Nystagmus” implies a sequence of involuntary or reflex triggering of movements of both eyeballs with slow and rapid components, triggered by saccadic generators via brainstem pathways. Spontaneous nystagmus is always pathological and is indicative of a peripheral or central lesion. Peripheral lesions affect receptors in the semicircular canals and/or the first vestibular neuron while central disturbances affect subsequent neurons of the vestibular system. Gaze-dependent nystagmus is seen with lesions of the cerebellum, medulla oblongata as well as mesencephalic and diencephalic structures serving visual motor function.351 , 387 Fixation nystagmus occurs as pendulous movements during fixation of the globe and is a central congenital disorder of the oculomotor system. Spontaneous nystagmus is observed after elimination of fixation by means of illuminated glasses and is seen both with peripheral and central vestibular disorders. Since different lesions may result in similar forms of nystagmus, the presence of nystagmus alone is inadequate for topical diagnosis. Dissociated nystagmus, whereby the abducting eye exhibits larger excursions of the globe, indicates a lesion near the midline in the region of nuclei of extraocular muscles in the brainstem. Rotatory and vertical nystagmus also indicates the presence of a central lesion.
10.5 Auditory System
Sound waves reach the tympanic membrane through the external acoustic canal; its vibration is mechanically amplified by auditory ossicles in the middle ear which are then transmitted to the oval window (see ▶Fig. 7.5 and ▶Fig. 7.6). The resulting movements of the endolymph are perceived by hair cells of the organ of Corti in the cochlea and are transmitted to the chain of neurons of the auditory system (see ▶Fig. 10.14, ▶Fig. 10.15, ▶Fig. 10.16, and, ▶Fig. 10.17). Anatomically speaking, retrocochlear hearing loss results from a lesion of this chain of neurons. The first-order neurons of this chain are formed in the cochlea by the bipolar nerve cells of the cochlear (spiral) ganglion. Their peripheral processes innervate the bases of the hair cells. The central axons of bipolar ganglion cells form the cochlear nerve (cochlear division of the VIIIth cranial nerve), which emerges from the petrous part of the temporal bone through the internal acoustic opening and enters the medulla oblongata at the cerebellopontine angle. The central axons then divide into two branches: one extending toward the posterior cochlear nucleus and the other in the direction of the anterior cochlear nucleus. The second-order auditory neurons originate in these two nuclei:
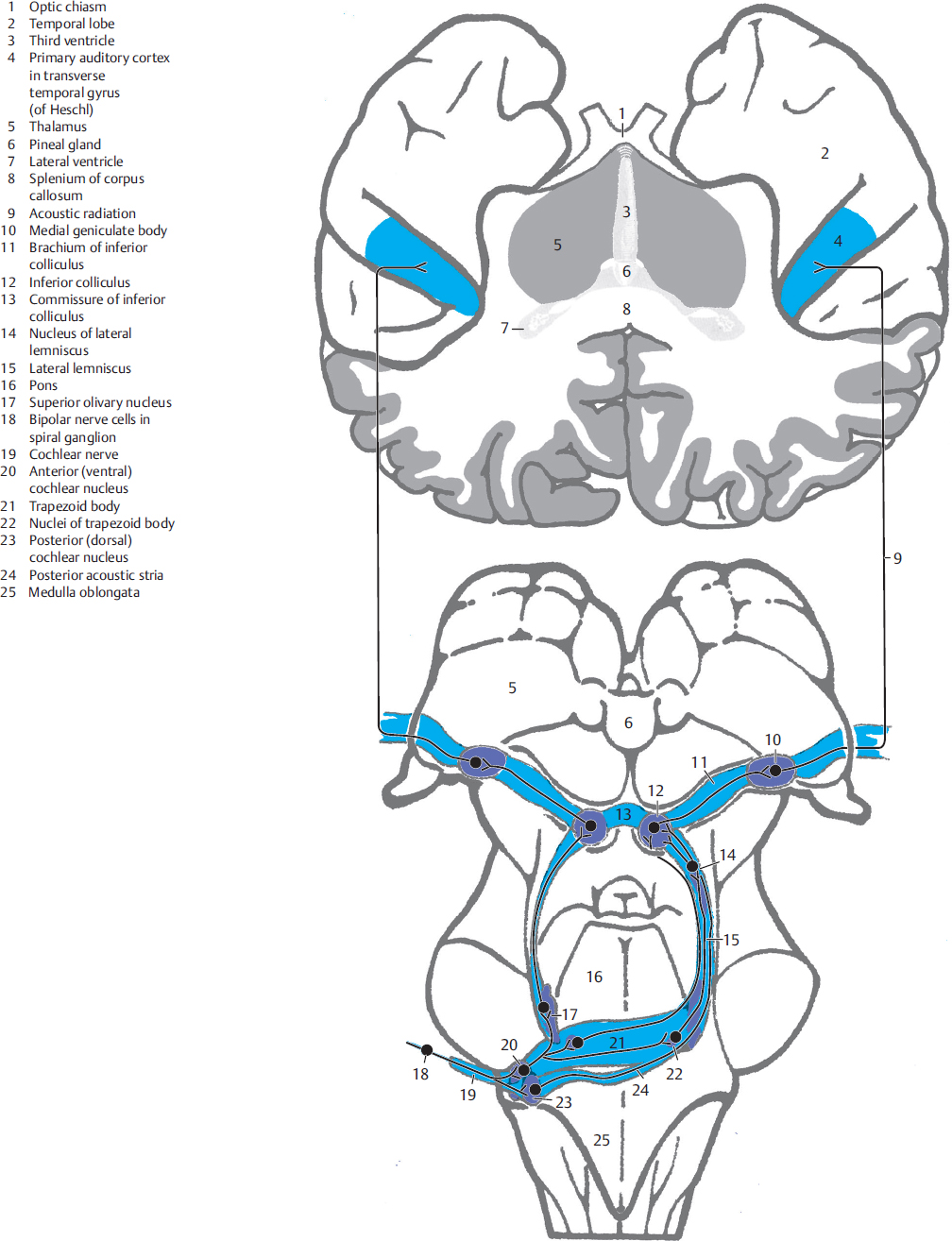
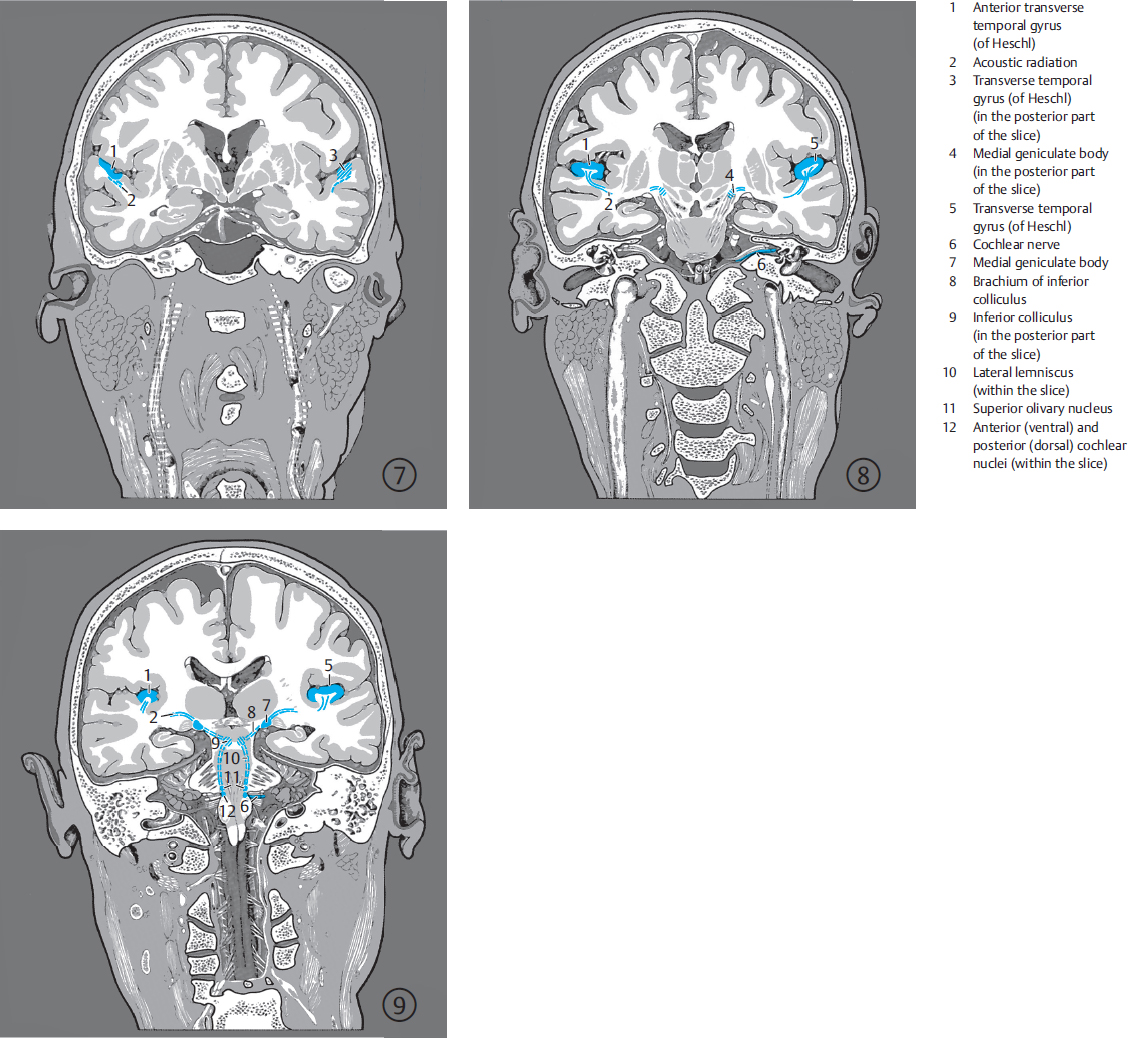
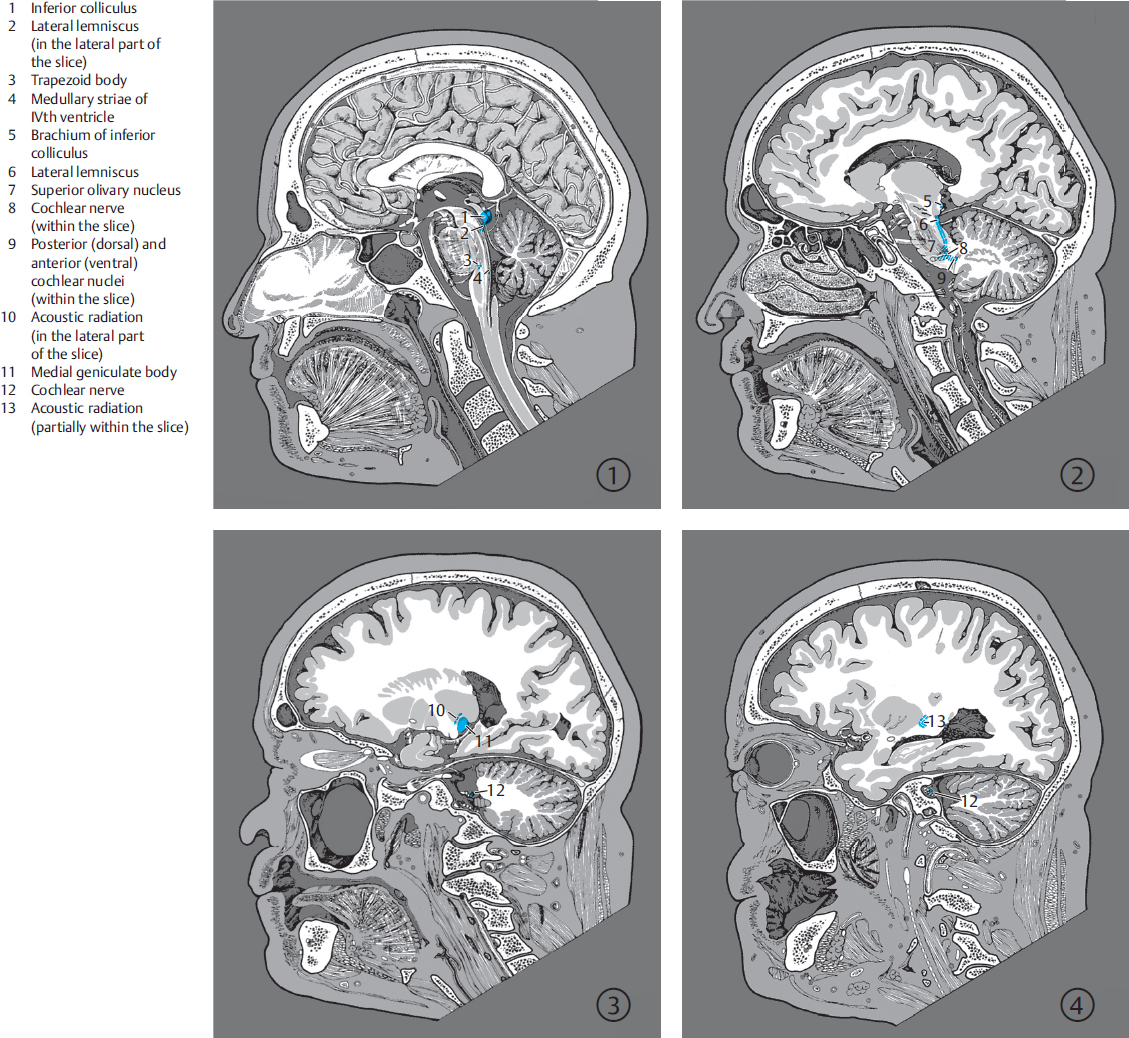
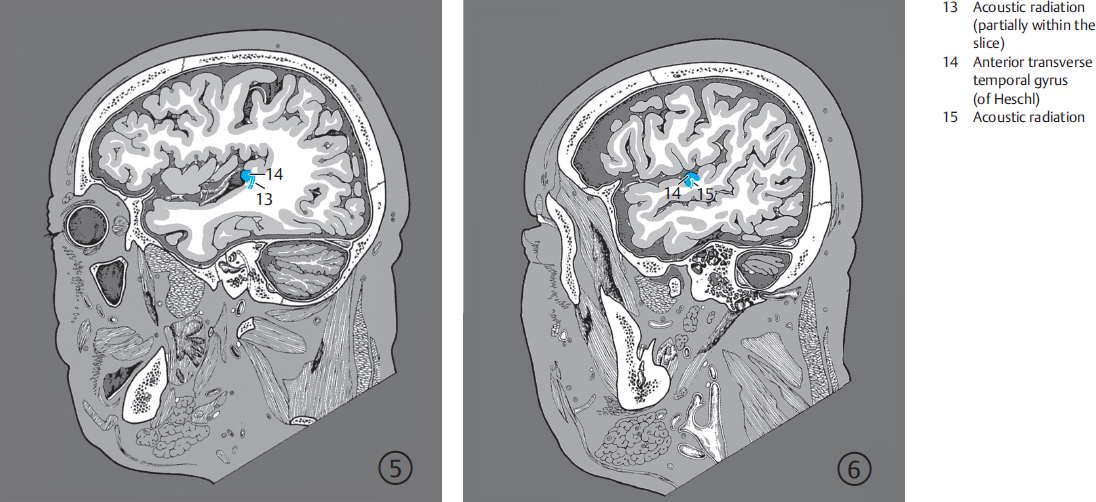
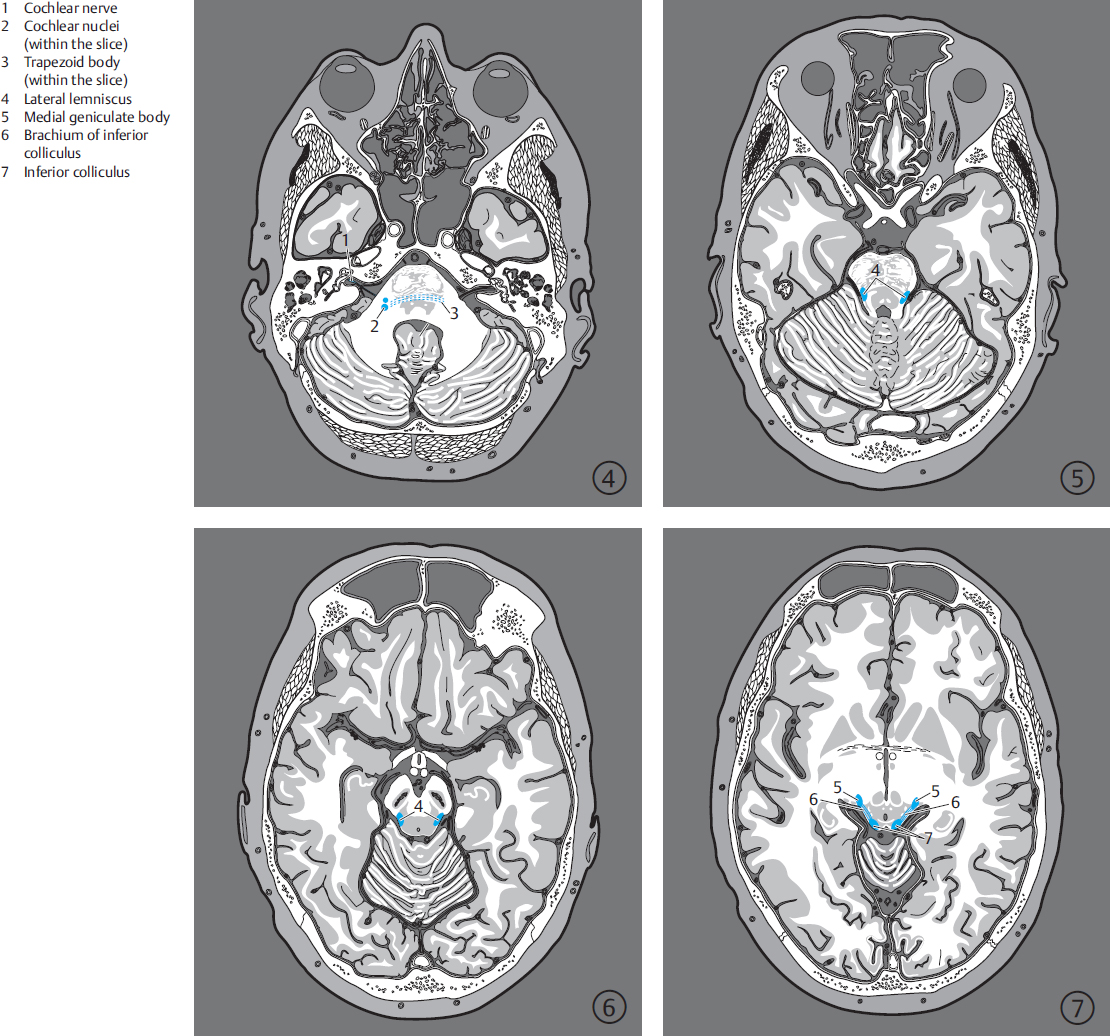
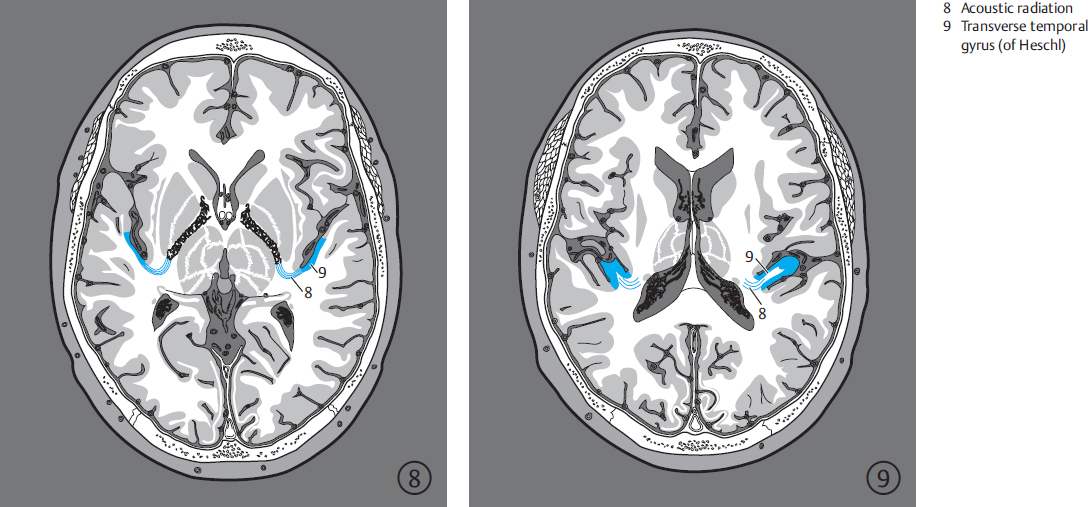
The axons of the posterior cochlear nucleus belong to the posterior part of the auditory pathway and pass along the floor of the rhomboid fossa immediately beneath the medullary striae of the IVth ventricle. Crossing over to the opposite side, they pass anteriorly and ascend in the lateral lemniscus to reach the inferior colliculus. Additional neurons may be connected along the way. Auditory signals are carried from the axons of nerve cells of the inferior colliculus via the brachium of the inferior colliculus to the medial geniculate body; the last neurons of this auditory chain pass from here through the auditory radiation to the primary auditory cortex (area 41, see ▶Fig. 7.53). The latter is located approximately in the anterior transverse temporal gyrus (of Heschl) in the depths of the lateral sulcus.225 Heschl’s gyrus is only visible on the cerebral convexity by splaying the lateral sulcus.
The anterior auditory pathway runs in the trapezoid body from the anterior cochlear nucleus via the superior olivary nucleus and via the nuclei of the trapezoid body, crossing to the opposite side to join the lateral lemniscus. It then follows an identical course as the posterior part of the auditory pathway already described. Another part of the anterior auditory pathway remains on the same side, ascending ipsilaterally through the aforementioned subcortical centers to the primary auditory cortex of the cerebrum (area 41).
This ipsilateral and contralateral course of the auditory pathway allows highly specialized neurons to determine travel time between two sources of sound when one is more distant from one ear than from the other, thereby enabling directional localization of a source of sound by hearing alone. The second-order and higher auditory neurons are highly specialized in the interpretation of specific sound patterns, and may filter out useful sounds, for example, speech from background noise.
The posterior and anterior cochlear nuclei (see ▶Fig. 6.7b) are situated in the medulla oblongata immediately anterior to the point of entry of the vestibulocochlear nerve at the pontomedullary junction.579 The cochlear nuclei lie on the lateral surface of the medulla oblongata at the level of the lateral recess of the IVth ventricle (see ▶Fig. 6.7b and ▶Fig. 10.15). The inferior cerebellar peduncle borders the cochlear nuclei at their anteromedial aspect, which helps in indirect localization of cochlear nuclei in MR images.19
Only the larger, anterior part of the auditory pathway is represented in ▶Fig. 10.15, ▶Fig. 10.16, and ▶Fig. 10.17 and courses through the trapezoid body. Fibers of the trapezoid body exit the cochlear nuclei at their anterior border and run in a gradual upward direction anterior to the inferior cerebellar peduncle and continue to the ipsilateral and contralateral auditory complex consisting of the superior olivary nucleus and the nuclei of the trapezoid body. The fibers traverse the anterior part of the medial lemniscus and are situated posterior to pontine nuclei in the inferior region of the pons. The trapezoid body may be identified directly on MR images as well as by virtue of its topographic relationship to the medial lemniscus.19
The lateral lemniscus (see ▶Fig. 6.9b, ▶Fig. 6.10b, ▶Fig. 6.11b, and ▶Fig. 10.14) originates in the nuclear complex of the superior olivary nucleus and in the nuclei of the trapezoid body and terminates in the inferior colliculus (see ▶Fig. 6.12b). It measures approximately 25 mm in length. Auditory signals may be relayed through synapses in one or more nuclei of the lateral lemniscus. These have not been described in greater detail here and have not been depicted in ▶Fig. 10.14, ▶Fig. 10.15, ▶Fig. 10.16, ▶Fig. 10.17. The lateral lemniscus runs upward in the lateral part of the tegmentum of the pons and lies immediately beneath the posterolateral surface of the pontine tegmentum at the junction between midbrain and pons. It borders the lateral surface of the superior cerebellar peduncle near the inferior colliculus, radiating into the latter from its anterolateral aspect.
The inferior colliculus is visualized in the first sagittal section as an inferior bulge of the quadrigeminal plate lying posterior to the aqueduct of the midbrain (see ▶Fig. 4.2a and ▶Fig. 4.2b). The lateral lemniscus may be identified on axial MR images by virtue of its contrast with neighboring structures.130 The inferior colliculus is clearly visualized on sagittal, axial, and coronal MR images due to its exposed position.19 , 511
Perikaryal axons of the inferior colliculus form the brachium of inferior colliculus, a narrow band of fibers on the lateral aspect of the tegmentum of the midbrain. At the junction of the midbrain and the diencephalon, the brachium of the inferior colliculus curves laterally over about 5 mm to enter the medial geniculate body at its posteromedial aspect. The medial geniculate body lies laterally to the midbrain inferiorly within the diencephalon. The anterior surface of the medial geniculate body (see ▶Fig. 3.10a, ▶Fig. 3.10b, ▶Fig. 4.4a, ▶Fig. 4.4b, and ▶Fig. 5.8) borders the internal capsule. The medial geniculate body measures approximately 8 to 9 mm in its largest diameter and 5 to 6 mm in its vertical span. It is composed of three parts:
Secondary medial nucleus: This small, secondary nucleus lies posteromedial to the principal nucleus and contains large cells.399 Electrophysiological examinations indicate that it obtains somatosensory, vestibular and auditory afferents and is thus multimodal.10 , 399
Secondary dorsal nucleus: This is a small triangular nuclear area which receives visual and auditory signals.
Secondary ventral nucleus: This is the largest nuclear area, and contains small cells. Also termed the “principal division”, it receives purely auditory signals.279
The fibers of the brachium of the inferior colliculus reach the posteromedial aspect of the medial geniculate body; emerging fibers exit as the acoustic radiation anterolaterally.312 , 314
The acoustic radiation interconnects the medial geniculate body with the primary auditory cortex. It was described for the first time in humans on myelogenetic microscopic specimens of infant brains461 and further details were revealed by subsequent studies.73 , 315
The acoustic radiation begins at the anterolateral border of the medial geniculate body and its initial fibers ascend while curving laterally, lying above the optic tract and below thalamoparietal fibers.
It is bordered by the lateral geniculate body postero-inferiorly, posterosuperiorly by the pulvinar and antero-superiorly by the rest of the thalamus. Further laterally, the optic radiation borders the acoustic radiation at its posterior aspect. The acoustic radiation passes through the posterior end of the posterior limb of the internal capsule and then posterior to the lateral part of the globus pallidus and along the putamen and claustrum. It finally curves anteriorly around the posterior border of the insular cortex and ascends in the white matter of the transverse temporal gyrus to reach the primary auditory cortex.
The primary auditory cortex is known as “area 41” in cytoarchitectonic terms.650 Brodmann76 localized area 41 roughly in the transverse temporal gyrus region (see ▶Fig. 7.53). Area 41 continues in an anterior direction over the transverse temporal gyrus. Area 42 forms an arc encompassing field 41. The area corresponding to field 41 is described as “area supratemporalis granulosa” on the anterior transverse temporal gyrus (of Heschl).155 The primary auditory cortex was localized in the medial aspect of the transverse temporal gyrus and the adjoining part of the planum temporale using pigmentarchitectonic methods.68 , 69 Auditory evoked potentials from cortical auditory fields were recorded intraoperatively in over 20 patients102 , 307 as part of electrophysiological studies. Potentials were relatively constant and had short latencies over the medial two-thirds of the anterior and posterior transverse temporal gyri while the area surrounding the planum temporale and the superior temporal gyrus showed potentials with smaller amplitudes and long latencies. As a rule, two transverse temporal (Heschl) gyri (anterior and posterior) are seen on the right while one is visualized on the left.182 , 183 The transverse temporal gyrus lies above the superior temporal gyrus in the depths of the lateral sulcus. It runs from the posterior edge of the insula separated from it by its circular sulcus, descends obliquely from posteromedial to anterolateral and is limited posteriorly by the transverse temporal sulcus. The entire transverse temporal gyrus and the primary auditory cortex are covered by the parietal operculum and are therefore not directly visible with an intact hemisphere. The transverse temporal gyrus may be easily identified on transversal, coronal and sagittal MR images.19 , 639 , 642
The auditory system (see Section 10.5) is the shortest neurofunctional system as compared with the medial lemniscus, visual, and corticospinal systems. Its primary cortical area lies in the depth of the lateral sulcus.312 , 314
Clinical Notes
Some of the mentioned brainstem nuclei are not merely relay nuclei but also reflex centers, connecting nuclei of the trapezoid body with motor nuclei of the VIIth cranial nerve, thereby forming a reflex arc from the spiral organ of Corti to the stapedius. This muscle contracts reflexively in response to high intensity sound and consequently dampens the transmission of sound waves from the tympanic membrane to the stapes (stapedius reflex). Loss of this reflex results in hyperacusis.
Additional reflex pathways extend from the inferior to the superior colliculi and modulate reflexes associated with eye and head movements in response to auditory stimuli. In addition, neurons of the reticular formation are interconnected in parallel with ascending sections of the auditory pathway.
A pathway from the auditory cortex and the inferior colliculi descends to the periolivary nuclei in the inferior part of the pons. An efferent pathway (olivocochlear tract of Rasmussen) originates in these periolivary nuclei and extends into the cochlea, terminating in the hair cells of the organ of Corti. This tract contains cholinergic (see Section 11.4) and enkephalinergic fibers (see Section 11.7.4). Experimentally, impulses arising in the auditory nerve can be suppressed by stimulation of this olivocochlear tract.
Clinical Notes
Clinically, middle ear, cochlear, and retrocochlear hearing loss may be differentiated. Identification of middle ear deafness involves a simple examination using a tuning fork. In addition to audiometry, neurophysiological tests such AEP or BERA are employed to distinguish a cochlear or retrocochlear hearing defect. Early acoustic evoked brainstem potentials were thus named in inconsistent international nomenclature.387 , 564 Recording AEP using surface electrodes following repeated acoustic stimuli enables localization of lesions both at the periphery and centrally at various levels in the brainstem.366
MRI or alternatively thin-section CT with IV contrast are diagnostic modalities of choice in suspected cases of acoustic schwannoma (commonly known as “acoustic neurinoma”) or other space-occupying lesions of the cerebellopontine angle as well as in patients with central hearing loss.
10.6 Visual System
The photoreceptors of the visual system lie in the retina (see ▶Fig. 10.18, ▶Fig. 10.19, ▶Fig. 10.20, ▶Fig. 10.21). Optical signals emitted from the rods and cones are transmitted through bipolar nerve cells to large multipolar nerve cells. The neurites of these multipolar neurons extend along the inner layer of the retina and converge at the optic disc, they pass through the lamina cribrosa of the sclera to form the optic nerve. Within the orbit, the optic nerve measures approximately 3 cm in length and pursues a slightly curved course to allow for free movements of the globe. This nerve crosses the bicommissural plane at an acute angle. The optic nerve passes through the optic canal, a bony channel approximately 5 mm in length, to reach the optic chiasm (see ▶Fig. 10.21a). The nerve is repositioned medially in the orbit with lateral movements of the eyeball.504 The optic nerve is ensheathed by the pia, arachnoid, and dura mater within the orbit with a narrow subarachnoid space; while these meningeal layers are firmly fused with the bony wall of the optic canal.
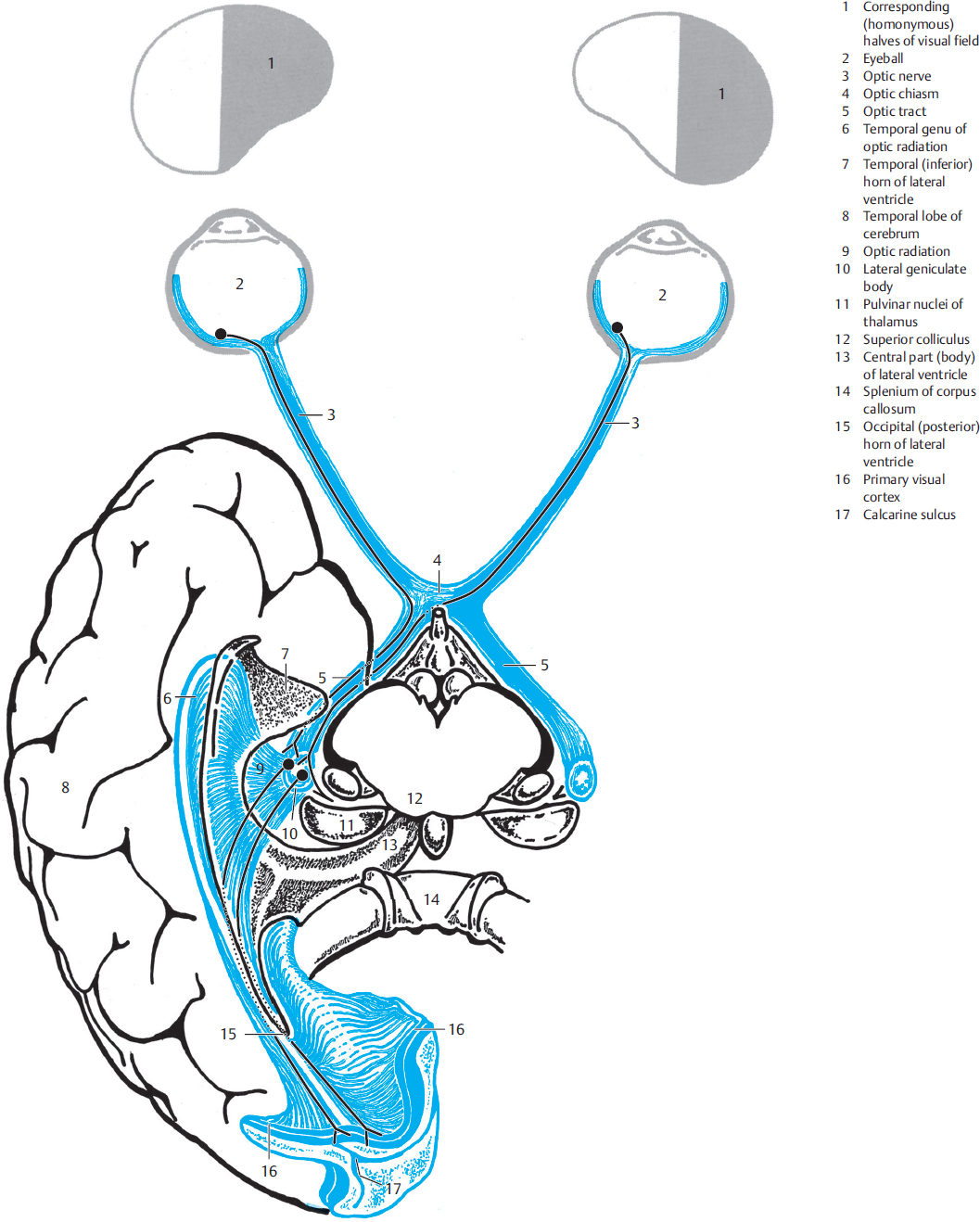
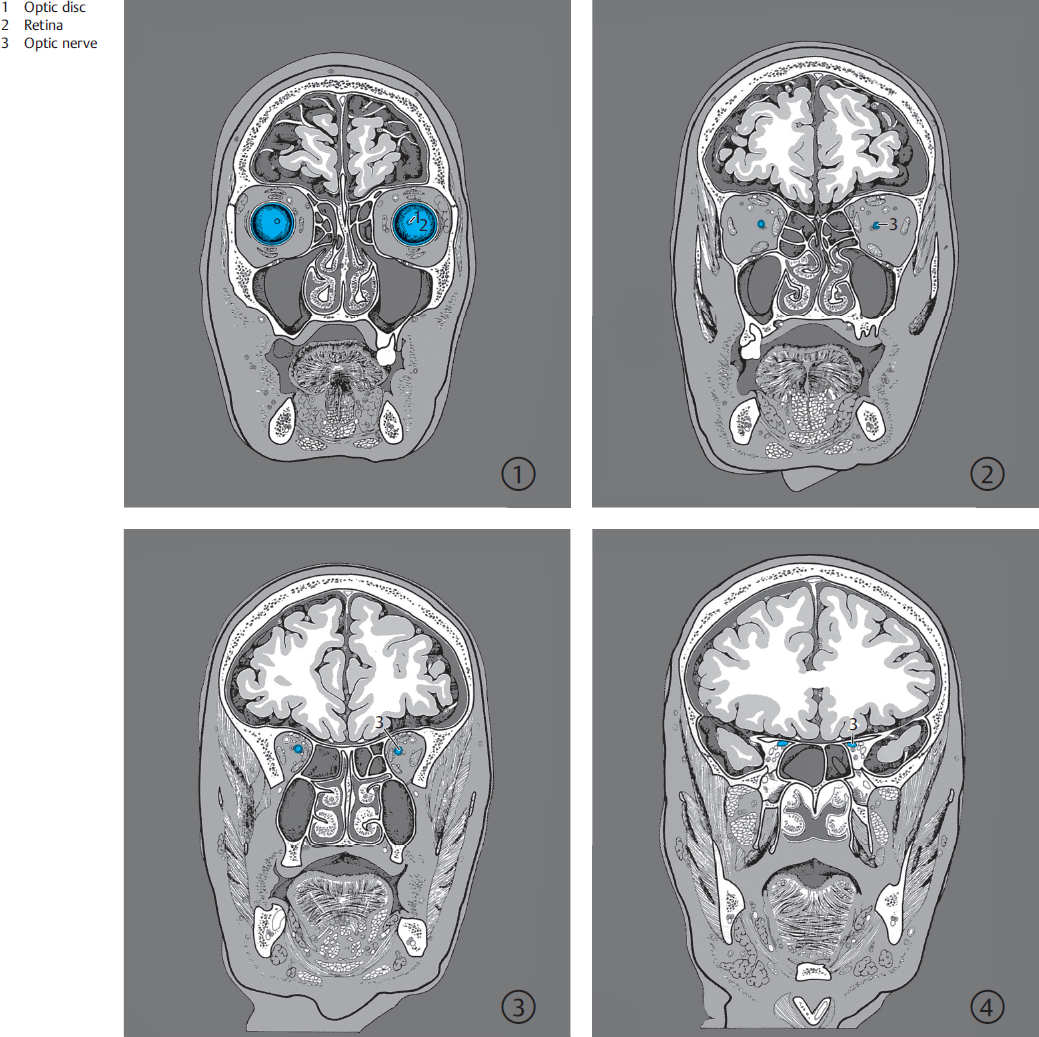
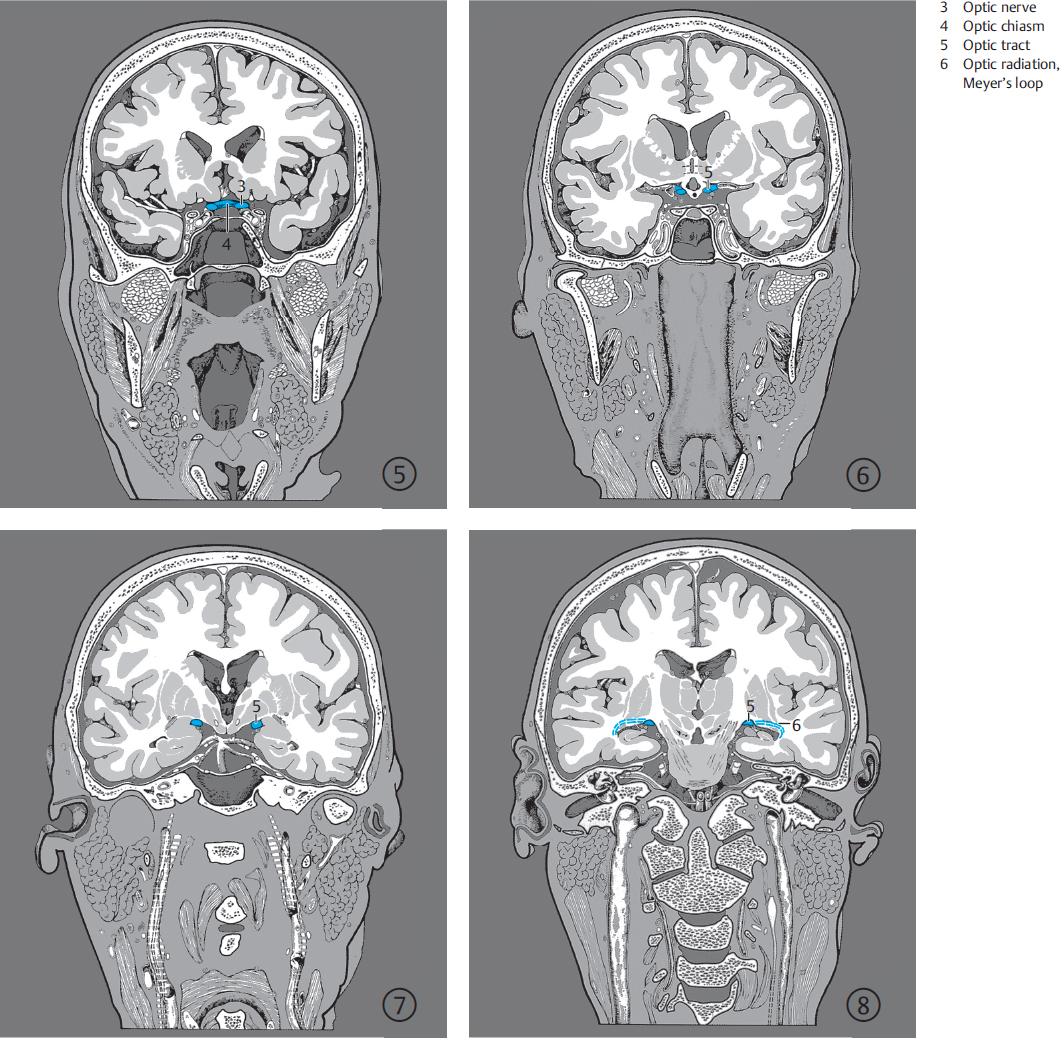
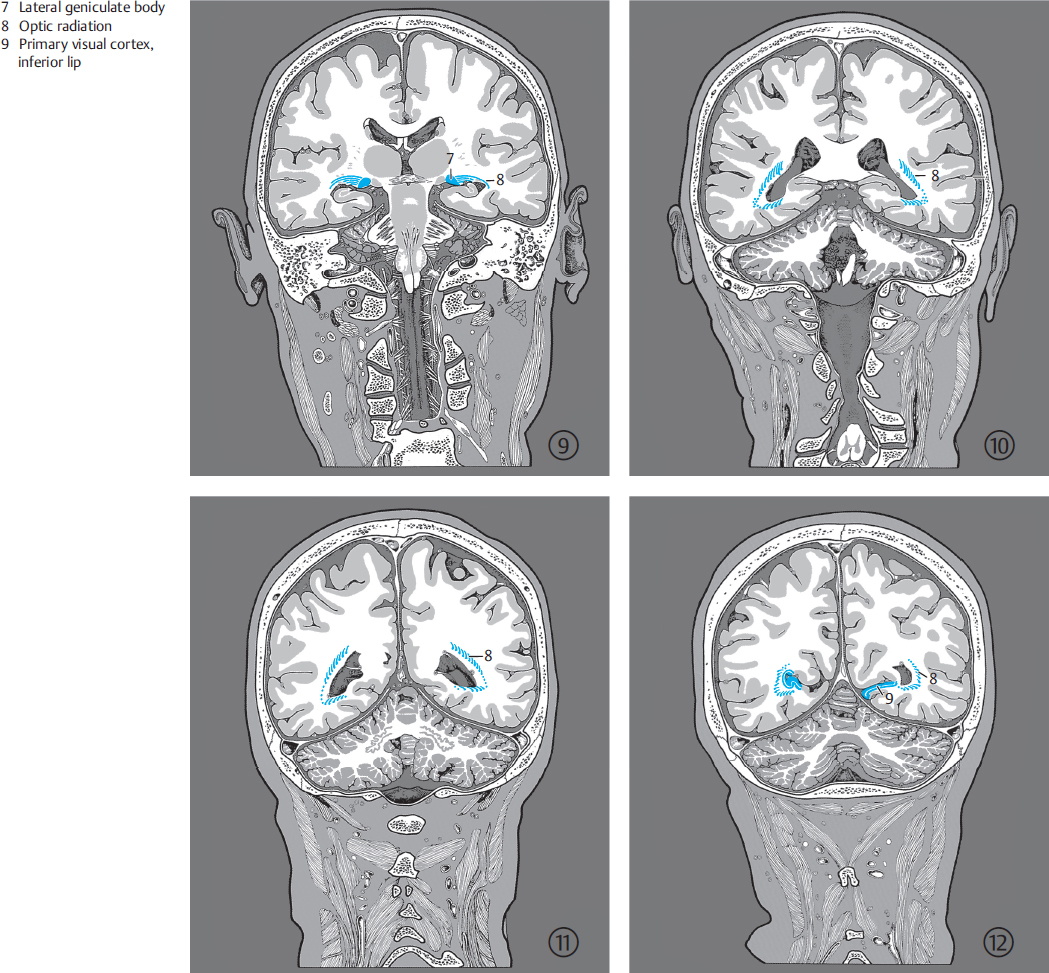
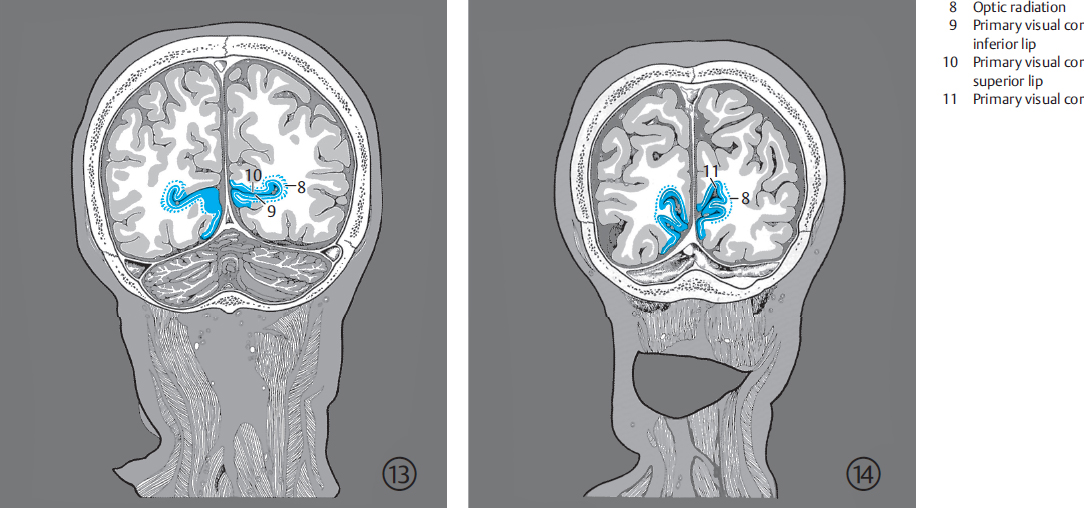
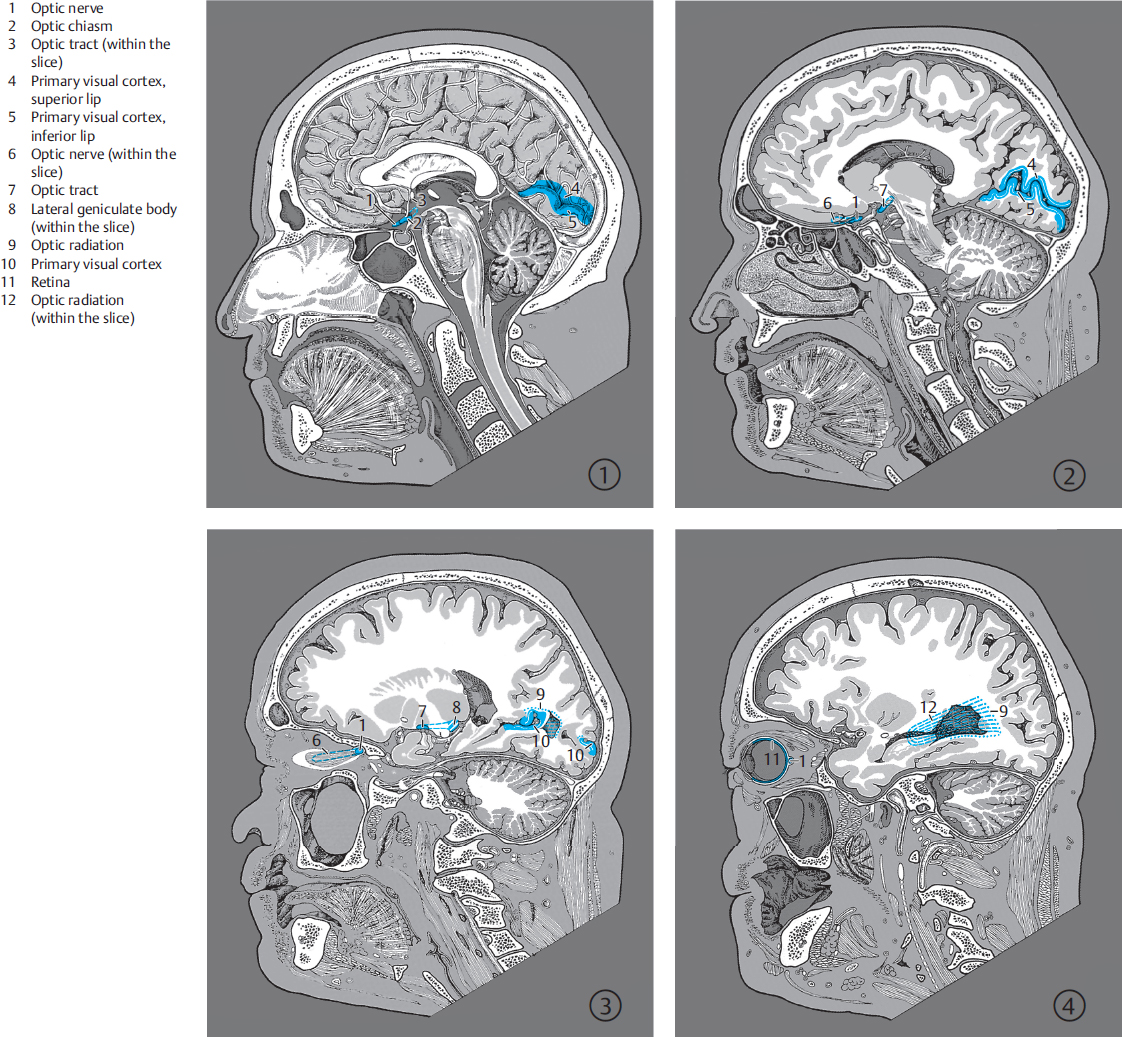
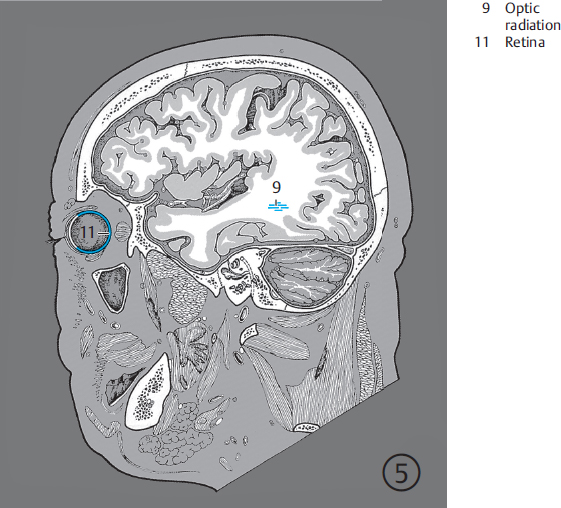
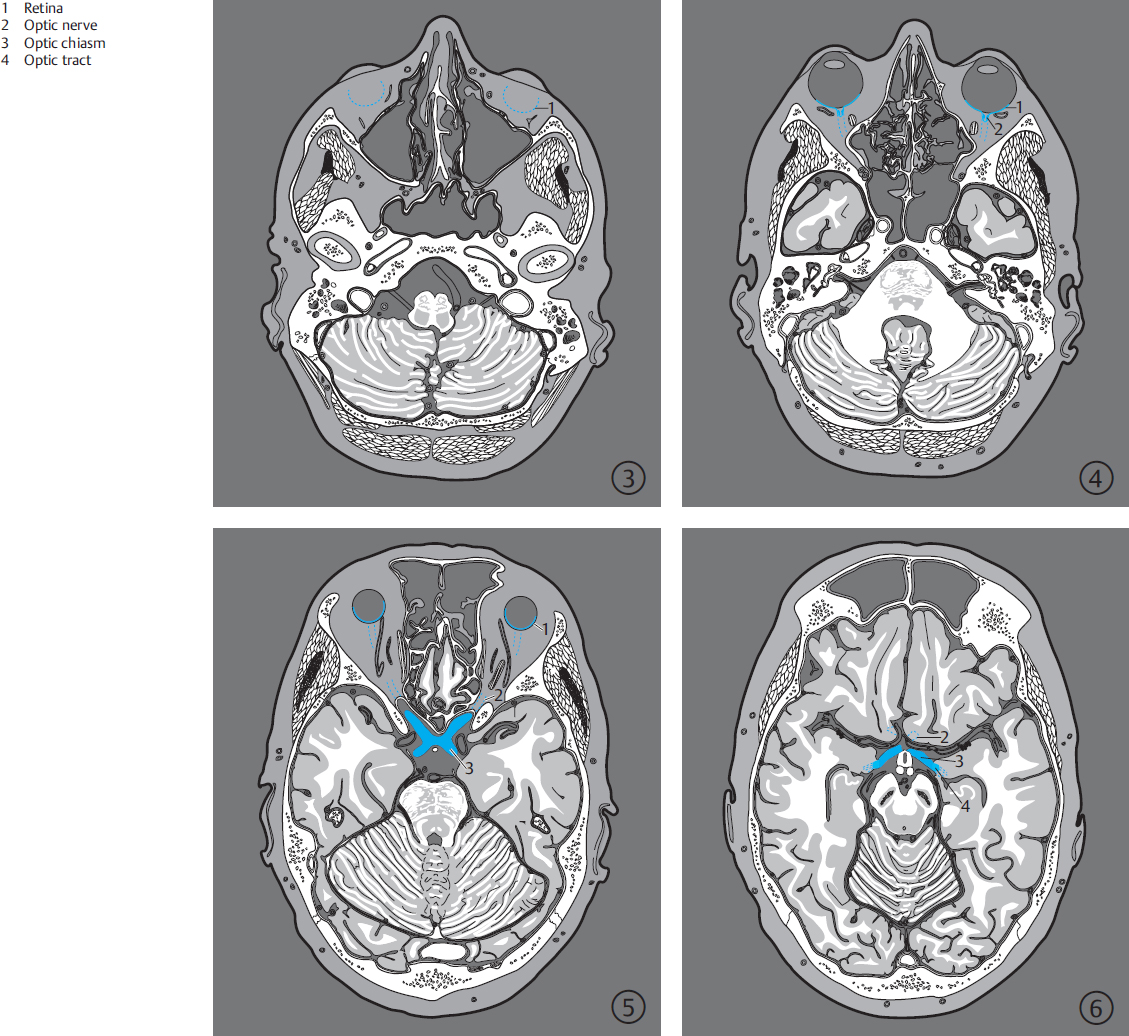
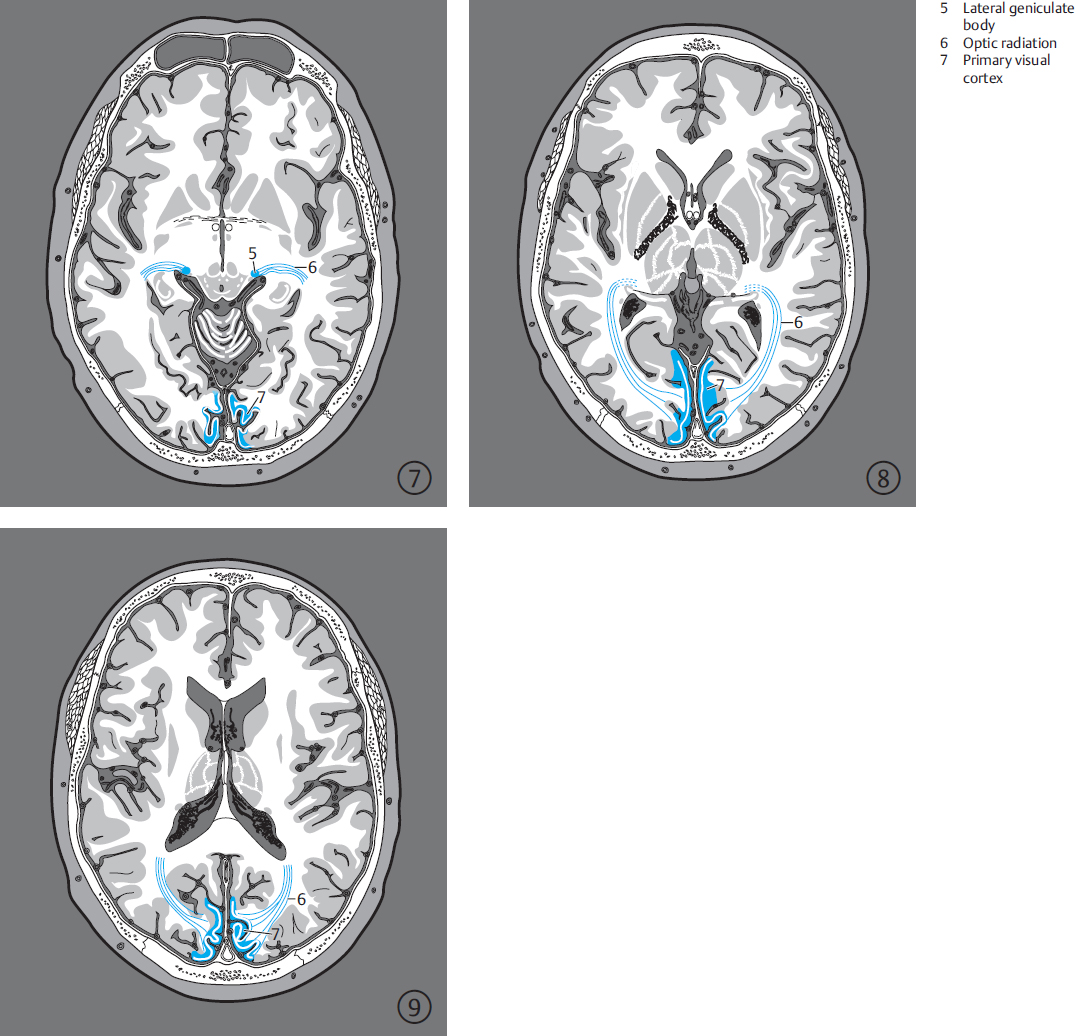
Inferiorly to the optic chiasm lie the sphenoid sinus and the sella turcica containing the pituitary gland (hypophysis). The hypothalamus is related posterosuperiorly while the internal carotid arteries lie laterally to the optic chiasm. Fibers from the nasal half of the retina (temporal visual field) cross in the optic chiasm, while fibers from the temporal half of the retina (nasal visual field) remain on the same side.
Both crossed and uncrossed fibers form the approximately 4 cm long optic tract. This tract arches along the border between the midbrain and the diencephalon to the lateral geniculate body where most of the fibers terminate (see ▶Fig. 3.10a, ▶Fig. 3.10b, ▶Fig. 5.8, ▶Fig. 10.19c, ▶Fig. 10.20a, and ▶Fig. 10.21b).
The lateral geniculate body lies inferiorly to the pulvinar nuclei of the thalamus and immediately laterally to the medial geniculate body. The largest axial cross section of the lateral geniculate body resembles the shape of a hat, with a maximum diameter of approximately 8 to 9 mm. Microscopically, the lateral geniculate body is composed of six cell layers separated by thin white zones (fibers of the optic tract). Most fibers of the optic tract terminate here in fourth-order neurons. Crossed optic nerve fibers terminate in layers 1, 4, and 6 and uncrossed fibers in layers 2, 3, and 5. Layers 1 and 2 are composed of magnocellular nerve cells while layers 3 to 6 consist of parvocellular nerve cells. A small section of optic tract fibers extends to the superior colliculi and pretectal areas which modulate reflexes of intrinsic and extraocular muscles. A third group of fibers forms the extrageniculate pathway leading to the cerebral cortex (see ▶Fig 10.19d).
The optic radiation arises from the lateral geniculate body arching at first over the temporal horn of the lateral ventricle before turning medially toward the visual cortex.106 , 107 , 263 , 603 Nerve cells from the medial half of the lateral geniculate body project mainly into the superior lip of the primary visual cortex and those from its lateral half to its inferior lip. The optic radiation may be identified on MR images and in histologic sections of infant brains aged 3 to 12 months.384 It lies lateral to the temporal and occipital horns of the lateral ventricle in an approximately sagittal plane (see ▶Fig. 10.19c) and appears hook-shaped in coronal sections, with the open part of the hook directed medially. These fibers of the optic radiation arise in the posterior half of the lateral aspect of the lateral geniculate body. The upper layer of fibers in the optic radiation contains extramacular fibers which run to the anterior part of the upper lip of the primary visual cortex, while its middle part contains fibers which carry signals from the macula lutea of the retina to the occipital region of the primary visual cortex. The lower layer of fibers of the optic radiation runs through the loop at the anterior edge of the temporal horn of the lateral ventricle, and passing underneath it, continues to the anterior part of the inferior lip of the primary visual cortex. Selective damage to this region impairs perception in the peripheral (extramacular) part of the upper quadrant of the visual field.236
The arc-like proximal part of the optic radiation, its genu or Meyer’s loop, is not clearly demarcated in histological specimens of juvenile and adult brains since it is combined with other fibers and appears “matted” in histological sections. These may be stained in isolation and their course identified only in infant brains in whom large projection tracts, such as the optic radiation, develop first. It must be remembered that the volume of infant brains more than doubles as growth occurs while transposing these findings to adult brains.
Therefore, only an approximate reconstruction of the genu of the optic radiation is possible in adult life. The further course of the optic radiation in the stratum sagittale externum has been identified histologically and macroscopically with a magnifying glass and lies in the regions of the temporal and occipital horns of the lateral ventricle.
The primary visual cortex [striate area or area 17 according to Brodmann (see Fig. 7.53)], lies mainly in the medial part of the occipital lobe surrounding the calcarine sulcus; see also neuronal networks for higher-order visual fields. More than half of the primary visual cortex lies on the inner surface of the calcarine sulcus, which extends deep into the occipital lobe up to the lateral ventricle. The white matter of the primary visual cortex borders the occipital horn of the lateral ventricle in the region of the calcarine spur. The visual cortex extends anteriorly inferior to the calcarine sulcus before it joins the parieto-occipital sulcus. The shape of the primary visual cortex of the right and left hemispheres is asymmetrical, exhibits interindividual variability,560 and may extend up to the occipital pole of the cerebrum. It largely follows a course akin to that of the bikommissural plane.574 Variable arches and depressions of the cortex are present within the calcarine sulcus.508 The primary visual cortex is histologically classified as being of a granular, sensory type. A macroscopically and microscopically visible fiber layer, known as the occipital stripe of Gennari (see ▶Fig. 5.10c), is present in the fourth layer and is composed of myelinated axons extending from the lateral geniculate body to the primary visual cortex.
Clinical Notes
Clinical experience with temporal corticectomies showed no impairment of the visual field if excisions lay more than 1 cm anterior to the verticofrontal plane of the posterior commissure.573 This plane runs through the posterior commissure and is perpendicular to the median plane and the bicommissural plane of Talairach. These findings suggest that the optic radiation lies at a variable distance from the frontal pole of the temporal horn of the lateral ventricle, and does not extend as far anteriorly as has been described under Meyer’s loop.263 Furthermore, partial temporal lobectomy may result in small, large, or no visual field defects.23 These results suggest a variability of Meyer’s loop.
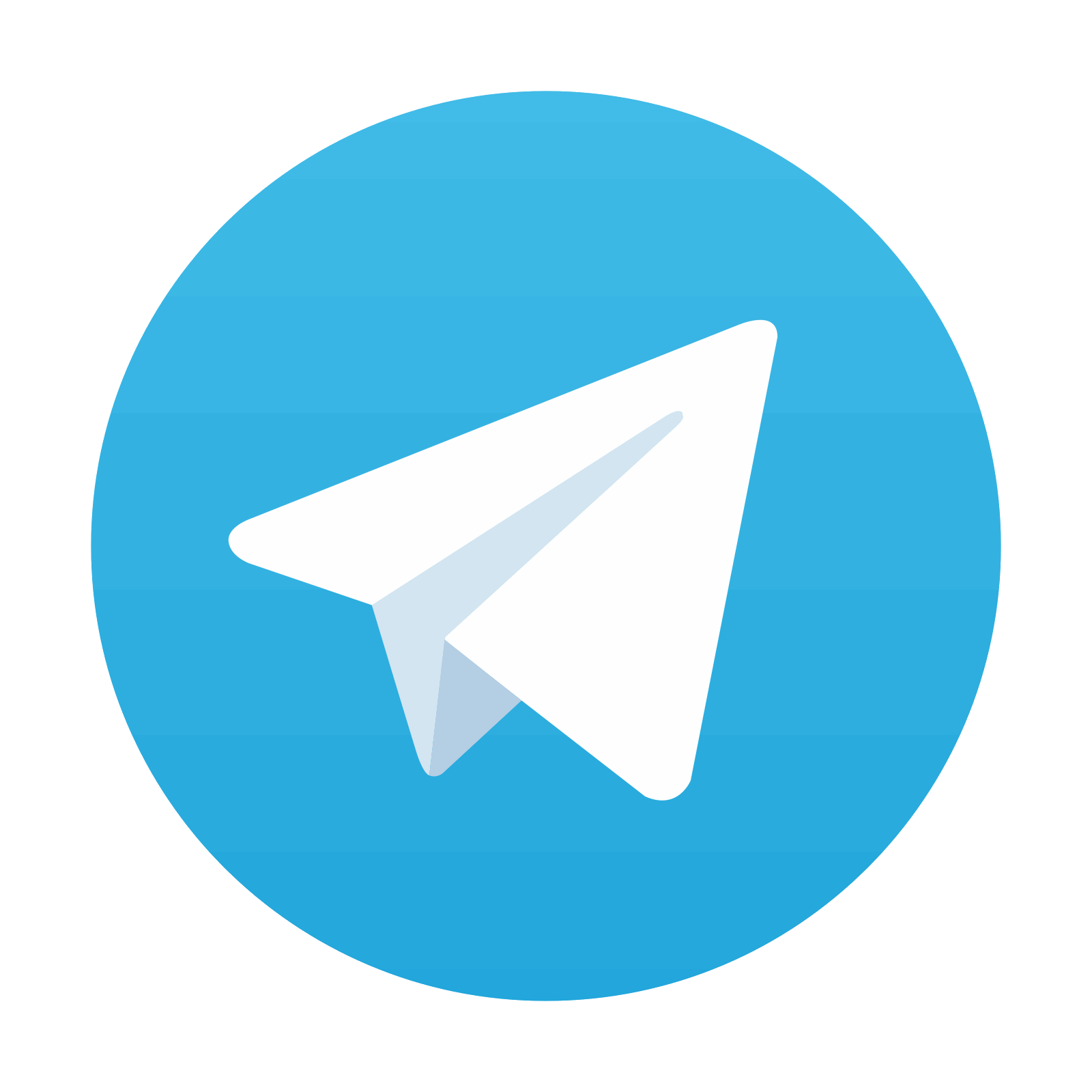
Stay updated, free articles. Join our Telegram channel

Full access? Get Clinical Tree
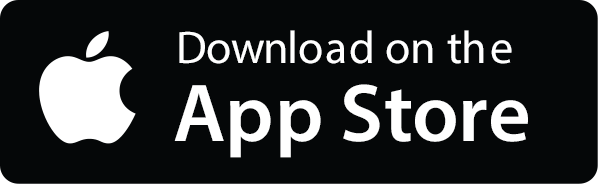
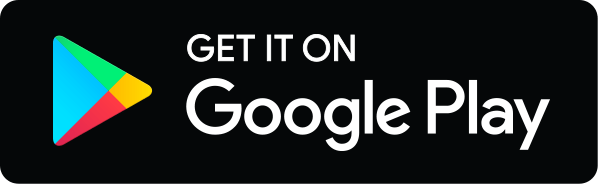
