11C-Based PET- Radiopharmaceuticals of Clinical Value
Kjell Någren
Christer Halldin
Positron emission tomography (PET) imaging with carbon 11 (11C) radiopharmaceuticals is challenging because of the short half-life of the radionuclide 11C, which is approximately 20 minutes. Usually 300 to 370 MBq (8 to 10 mCi) is injected intravenously into the patients. The synthesis time is 30 to 40 minutes (the time from end of cyclotron irradiation until availability of the radiopharmaceutical for injection), and the imaging time is 40 to 90 minutes. The radioactivity available for the first patient is 4 to 8 times lower than that obtained from the cyclotron, whereas for a potential second patient the radioactivity is 16 to 32 times lower. This quick calculation shows that it is difficult to introduce 11C radiopharmaceuticals into a routine clinical environment, and thus they are more frequently used in research environments partway between clinical practice and research.
Nevertheless, 11C radiopharmaceuticals play an important part in the development of PET tracers, as it is usually simpler to test principle concepts with 11C-labeled substances than with fluorine 18 (18F)–labeled substances. Endogenous molecules do not contain fluorine, and thus it is important to compare the in vivo distribution of the endogenous molecule, traced with 11C, and the 18F labeled analog to confirm that the analog retains important properties of the endogenous molecule. One such substance is [11C]methionine, which several institutions are using for brain tumor imaging. Other tumor-imaging agents clinically used in some institutions are [11C]choline and [11C]thymidine. They have shown promise for the imaging of prostate tumors and for the imaging of cellular proliferation, respectively. Both also have become available as 18F-labeled analogs. In cardiac imaging, the most important clinically useful compound, [11C]hydroxyephedrine, appears to yield relevant information in heart transplant recipients and patients with myocardial hypertrophy. In the brain, the most widely used 11C compounds are [11C]flumazenil for the assessment of epilepsy and [11C]raclopride for the evaluation of diseases of the dopaminergic receptor system. Clinical examinations with such compounds can realistically be done only if an in-house cyclotron and a radiopharmacy unit are available.
Most clinical PET scanning is performed with [18F]fluoro-deoxyglucose (FDG) as the radiotracer. However, an increasing number of clinical studies are performed with 11C tracers in oncology, cardiology, and brain receptor studies (Table 17.1). This chapter focuses on PET 11C radiopharmaceuticals of special clinical value.
Radiochemistry and Radiopharmacy Requirements for Carbon 11 Radiopharmaceuticals
Compounds labeled with the ultrashort-lived isotope carbon 11 (11C) are not commercially available and must be prepared in the vicinity of a cyclotron (1,2,3). In a PET facility, the cyclotron, the radiochemistry laboratory, and the PET scanner(s) constitute the three main operative units. Today, most centers that do PET scanning use low- or medium-energy cyclotrons to produce one or more of the positron-emitting radionuclides: 11C, nitrogen 13 (13N),
oxygen 15 (15O), and fluorine 18 (18F). The ultrashort half-life of 11C implies that it must be produced immediately before use by an adjacent cyclotron. 11C is formed by means of nuclear reactions that occur upon bombardment of target mediums with charged particles. The production of 11CO2 is an example of this hot-atom chemistry. The irradiated material is, in the case of 11C, typically in a gaseous state. Irradiation yields are dependent on such factors as target shape, the design of the target foil, and the efficiency of target cooling. The amount of radioactivity that can be obtained is regulated by the energy of the accelerated particles and the beam current imposed on the target.
oxygen 15 (15O), and fluorine 18 (18F). The ultrashort half-life of 11C implies that it must be produced immediately before use by an adjacent cyclotron. 11C is formed by means of nuclear reactions that occur upon bombardment of target mediums with charged particles. The production of 11CO2 is an example of this hot-atom chemistry. The irradiated material is, in the case of 11C, typically in a gaseous state. Irradiation yields are dependent on such factors as target shape, the design of the target foil, and the efficiency of target cooling. The amount of radioactivity that can be obtained is regulated by the energy of the accelerated particles and the beam current imposed on the target.
Table 17.1 Carbon 11 Radiopharmaceuticals for Clinical Pet Studies | ||||||||||||||||||||||||||||||||||||||||||||||||||||||||||||||||||||||||||||||||||||||||||||||||||||||||||||||||||||||||
---|---|---|---|---|---|---|---|---|---|---|---|---|---|---|---|---|---|---|---|---|---|---|---|---|---|---|---|---|---|---|---|---|---|---|---|---|---|---|---|---|---|---|---|---|---|---|---|---|---|---|---|---|---|---|---|---|---|---|---|---|---|---|---|---|---|---|---|---|---|---|---|---|---|---|---|---|---|---|---|---|---|---|---|---|---|---|---|---|---|---|---|---|---|---|---|---|---|---|---|---|---|---|---|---|---|---|---|---|---|---|---|---|---|---|---|---|---|---|---|---|
|
Labeling of a compound without affecting its biochemical properties is possible by exchange of stable atoms present in the parent molecule. For instance, carbon 12 (12C) is replaced with the corresponding positron-emitting radionuclide 11C. The small kinetic isotope effect due to the different atomic weights of 11C and 12C that may occur is considered negligible for most applications. The ultrashort half-life especially of 11C make it advantageous for sequential investigations with short time intervals in the same individual (animal or human), allowing the subject to be its own control. The use of radionuclides with relatively longer half-lives, such as 18F or bromine 76 (76Br), provides an opportunity to follow the radioactivity from the radiolabeled compound for a longer time but leads to the use of analogs of natural compounds and also to a higher radiation dose to the patient.
In the preparation of compounds labeled with radionuclides such as 11C, a series of requirements related to the ultrashort half-life of the radionuclide must be taken into account. The radioactivity must be introduced by readily accessible radiolabeled precursors. The labeling reactions must occur rapidly and with high yield. The principal strategy is to introduce the labeled precursor as late as possible, followed by none or just a few additional reactions before the final purification. In addition, handling of high levels of radioactivity on a routine basis requires an automated or remote-controlled experimental setup installed in a lead-shielded hot cell to ensure maximal radiation protection.
Online and one-pot reactions shorten the total synthesis time and reduce radioactivity losses.
Online and one-pot reactions shorten the total synthesis time and reduce radioactivity losses.
Commercially available dedicated cyclotrons (10 to 18 MeV) are well suited for the production of 11C for PET radiochemistry purposes. A large number of 11C-labeled precursors can either be produced directly in the target or obtained via rapid online reactions starting from 11CO2 or 11CH4. The most widely used 11C-labeled precursor has been [11C]methyl iodide. Recently a more reactive precursor, [11C]methyl triflate, was demonstrated to give higher yields with shorter reaction times and smaller amounts of precursors for many commonly used PET radiopharmaceuticals. Today, most PET centers have either commercially available or in-house–constructed units for the production of 11C-labeled methyl iodide or methyl triflate. Among the radiopharmaceuticals listed in this chapter, 11C-labeled methionine, MeAIB, choline, metomidate, PK 11195, HED, raclopride, flumazenil, α-methyl-tryptophan, and PIB can all be produced from such a production unit by using, in most cases, commercially available des-methyl precursors (3). [11C]acetate can be prepared by simple reaction and purification conditions, whereas 11C-labeled 5-OH-β-tryptophan, tyrosine, thymidine, CGP 12177, and WAY-100635 production is more complicated, demanding a well-established in-house collaboration between skilled radiochemists and technicians.
Radiopharmacy regulations vary somewhat between countries, but guidelines can be found both in the European and U.S. pharmacopeias, which have entries for 11C-labeled radiopharmaceuticals such as raclopride and methionine. From a radiopharmacy viewpoint, two crucial steps of the production are purification and quality control, with high-performance liquid chromatography (HPLC) the standard method in both steps. The specific radioactivity (SA) of a radiopharmaceutical is defined as the ratio of radioactivity per mole of the labeled compound, with a maximum SA inversely related to the physical half-lives of the radionuclide. The maximum theoretical SA for 11C is 107 mCi/nmol, but because of difficulties in completely eliminating external sources of 12C, this high specific radioactivity is never obtained. An SA higher than 2,000 mCi/μmol (74 GBq/μmol) is sufficient for most of the radiopharmaceuticals used in PET investigations of receptors and transporters. For high-affinity 11C radiopharmaceuticals that require a higher SA, like [11C]FLB 457, the new method of [11C]methyl iodide/triflate production from [11C]methane has opened up the possibility of routinely obtaining a higher SA. [11C]methane can be produced within the target or externally (post-target), with the former method giving a higher SA. When producing endogenous compounds such as amino acids, acetate, and choline, a high SA is not needed. Efficient PET investigations require an unusually high degree of interdisciplinary collaboration and coordination in a very tight time frame, and this is especially evident in the case of ultrashort-lived radionuclides such as 11C. Thus PET centers must optimize all steps in this sequence of events to be able to achieve smooth operation and cost-effectiveness.
Carbon 11 Radiopharmaceuticals in Clinical Use
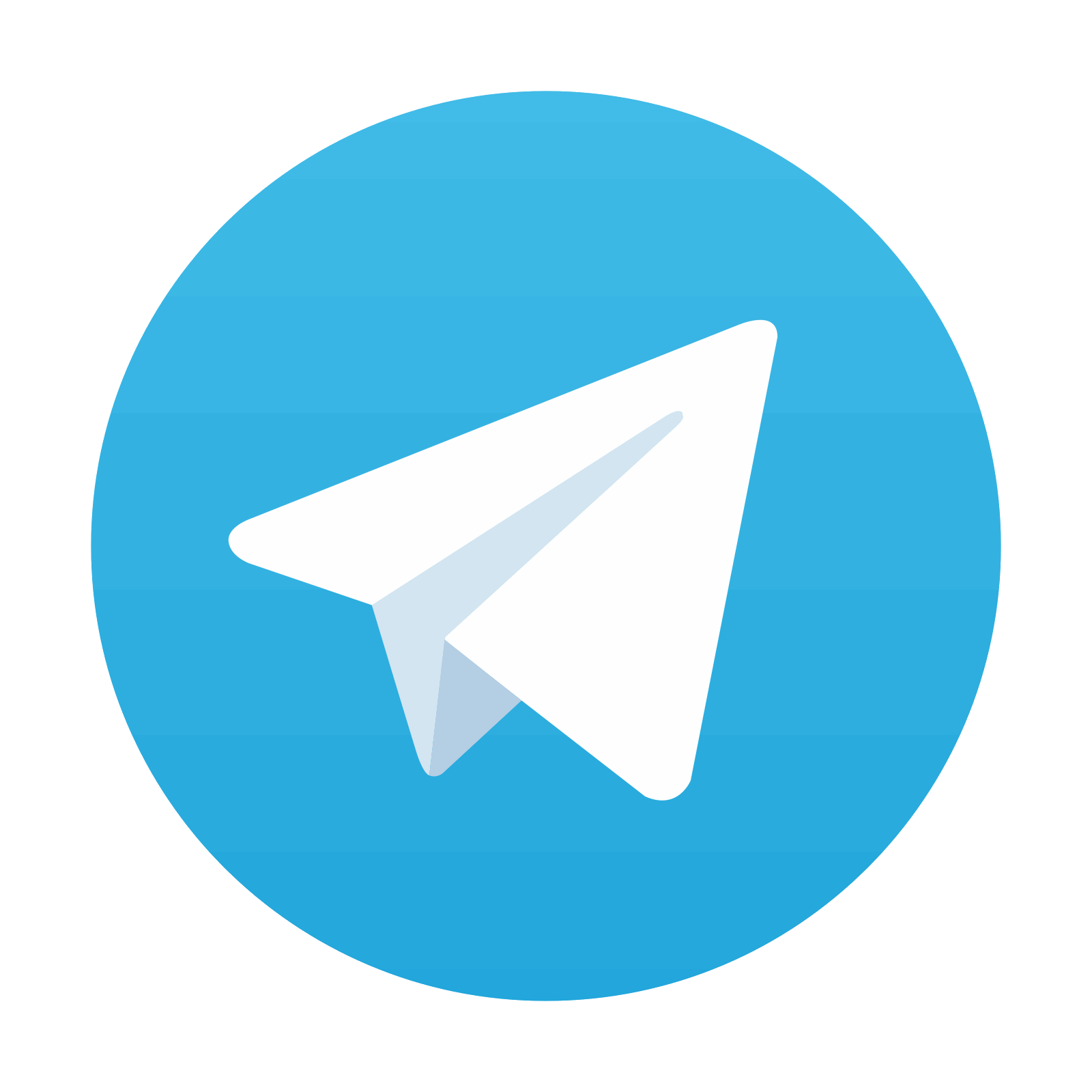
Stay updated, free articles. Join our Telegram channel

Full access? Get Clinical Tree
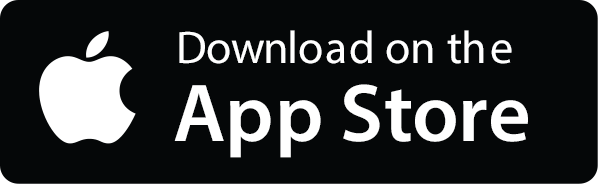
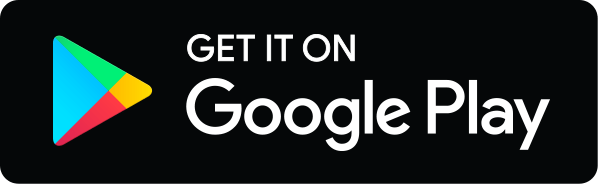