15 Image-Guided Neurosurgery: Intraoperative MRI
15.1 Introduction
Since the development and introduction of intraoperative magnetic resonance imaging (iMRI) in the late 1990s, applications for neurosurgical interventions have become increasingly widespread as more and more centers across the world adopt this technology. Following is a brief outline of the historical development of iMRI and a description of current applications.
15.2 Historical Background
The historical development and use of iMRI applications evolved out of the adoption of neuronavigational systems. With the development of frame-based and frameless applications, neurosurgeons were able to target deep intracranial lesions with previously unattainable accuracy. 1 , 2 , 3 , 4 , 5 , 6 , 7 , 8 , 9 , 10 The availability of neuronavigation greatly aided in localization of lesions as well as surgical planning, allowing for a minimally invasive approach toward intracranial surgery. However, the accuracy of neuronavigation was dependent on a preoperative scan. Once the cranial vault was accessed, and as an operation progressed, numerous factors (egress of cerebrospinal fluid, resection of tissue, local tissue swelling) could result in a shift of up to 5 mm, potentially compromising the accuracy of any navigation system. 6
The next step in attempting to improve accuracy during an operation was to develop real-time intraoperative imaging to provide updated information with which to guide a procedure. Intraoperative ultrasonography was one such development; however, use was limited because it was unable to interface with other simultaneously available navigational modalities. 11 Computed tomography was also explored in the 1980s, but was never widely adopted due largely to the perception of an unfavorable cost-to-benefit ratio. 12 , 13 , 14 , 15 With the multiplanar capability and image quality of MRI, development of an iMRI was the next logical progression.
The earliest experience of development and application was described by Peter Black and his colleagues at Brigham and Women’s Hospital in conjunction with General Electric Medical Systems. 16 , 17 The first of its kind, the Magnetic Resonance Therapy Unit at Brigham and Women’s Hospital represented a major collaboration and nearly a decade of work through the 1980s. This initial application used an open low-field scanner built within the operative suite, a so-called double doughnut configuration. The 0.15 T magnet configuration provided a spherical imaging volume of 30 cm in diameter and allowed a 56-cm space between two magnets where a surgeon and assistant could stand or sit to operate. The surgical field would remain within the scanner, which would allow for image acquisition during the procedure. A requisite for this configuration would be MRI-compatible instruments, which had to be developed independently. In addition, an integrated navigational system was also developed and could be updated with each scan that was done during the procedure. Although use of this early iMRI added increased operating time due to the additional time required to position a patient properly, there were no adverse events reported in this initial experience. Interventions that were described included stereotactic biopsies and cyst drainages, as well as craniotomies for tumor resection.
The experience published at Brigham and Women’s Hospital illustrated that iMRI could prove a powerful tool in the hands of a neurosurgeon. However, such a system would pose great costs in terms of both installation and maintenance. Other magnet configurations were also developed, including a high-field-strength cylindrical superconducting short-bore system, and a biplanar open MRI design. 18 Rudolph Fahlbusch and colleagues at Erlangen developed a side-opening high-field system where the operative table would rotate into a 1.5 T close-bored magnet. 19 Moshe Hadani and his colleagues in conjunction with Medtronic Navigation (Louisville, CO) introduced the PoleStar N-10 iMRI, which offered an open-configuration 0.12 T portable magnet. 20 This particular system had a much smaller footprint and could be stored within a small area in the operating room and brought into the surgical field. In addition, its small footprint meant that the system could be installed in a conventional operating room.
The open-configuration iMRI systems were limited by low-field magnets, and over time could not produce the same high-quality image resolution as the 1.5 T and 3 T scanners that were being introduced for diagnostic purposes. As a result, efforts to introduce iMRI systems using closed high-field scanners continued apace. One such configuration involved using a separate conventional operating room connected via a transport corridor to a room containing the scanner. 21 This design had some advantages. It allowed the institution to use a commercially available scanner instead of an iMRI-specific scanner. In addition, the use of a conventional operating room also allowed for standard surgical instruments. The twin room configuration also meant the MRI was available for conventional studies when not being used during surgery. The cumulative result was a more cost-effective approach than some of the dedicated intraoperative units in use elsewhere. Another configuration involves the use of an intraoperative rail-mounted system that brings the scanner to the patient. The 1.5 T magnet is stored in a separate room that is shielded from the operating room via sliding radiofrequency- and sound-shielded doors. This configuration also allows the magnet to be used while not in use in the operating room. The operating suite features an MR-compatible operating room table and an MR-compatible head holder designed to fit within special eight-channel intraoperative radiofrequency coils. Closed-magnet systems represent a larger percentage of iMRI systems that are currently being adopted worldwide.
15.3 Intracranial Tumor Surgery
The chief benefit of iMRI is in aiding with tumor resection (Fig. 15.1). During surgery, the brain parenchyma becomes distorted due to the loss of cerebrospinal fluid, edema, and tumor resection. As surgery progresses, this brain shift can lead to inaccuracies with neuronavigation that is based on a preoperative scan. iMRI can assist surgical procedures by providing real-time updates of image-guided neuronavigation. Prior to the introduction of iMRI systems, a patient might have to return to the operating room in the case of inadequate resection. With iMRI a surgeon can access real-time imaging to assess the extent of tumor resection (EOTR) and carry out any necessary re-resection prior to concluding the operation, thus eliminating the risk of return to surgery for inadequate resections. With such a tool neurosurgeons are able to provide more complete resections and theoretically improve survival for patients with gliomas. The initial experience with iMRI showed no adverse events related to use, and most authors cited a perceived benefit in assisting with resection control. 17 , 22 , 23 , 24 , 25 , 26 , 27 , 28 Characteristically there was a great deal of heterogeneity in patient populations within these studies with a mixture of low-grade and malignant gliomas. In addition, not all tumors operated on were intended to undergo gross total resection due to proximity to eloquent or critical structures.
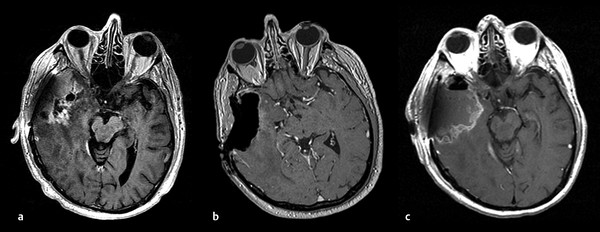
15.3.1 Low-Grade Gliomas
Patients with low-grade gliomas represent a population that can potentially benefit greatly from surgery with iMRI (Fig. 15.2). From a clinical standpoint, low-grade gliomas can have an initially indolent subclinical growth rate, but the normal pattern is for continuous growth and systematic progression to malignant transformation, in turn leading to neurologic disability and, ultimately, death. 29 , 30 From a treatment standpoint, there is a growing amount of evidence that EOTR can have a significant effect not only on the rate of tumor progression and overall survival but also on the risk of anaplastic transformation. 31 , 32 , 33 , 34 , 35 , 36 , 37 , 38 Unfortunately, low-grade gliomas can present a unique surgical challenge because it can be difficult to distinguish tumor from brain parenchyma. When the iMRI was first introduced, the role of surgery in the treatment of low-grade gliomas was still somewhat controversial.
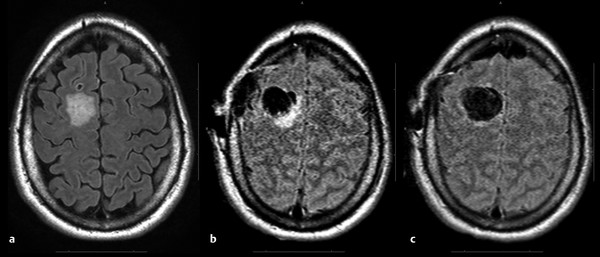
The Brigham and Women’s Hospital group published a study describing the use of iMRI for the treatment of low-grade gliomas that illustrated a survival benefit. 39 The study was retrospective with no true control group. Surgical data were pooled and compared with survival data using national databases. Given the results, this study was the first to illustrate a benefit of surgical resection combined with the use of iMRI for patients with low-grade gliomas. Unfortunately, data on exact EOTR were not given; therefore, it is difficult to say what role the use of iMRI with surgical resection contributed to the observed survival benefit. Subsequent studies have tried to quantify the benefit of iMRI with volumetric studies evaluating EOTR. Senft et al published a study using a portable low-field scanner. 40 They found that, in low-grade glioma patients, iMRI resection control led to a 47.6% chance of further tumor. However, this was coupled with a 90.5% chance of meeting surgical goals compared to 100% in the high-grade glioma group. This difference was attributed to differences of low-field iMRI images compared to those of postoperative imaging obtained with high-field scanners. Hatiboglu et al published a prospective study that specifically evaluated the benefit in EOTR using iMRI. 41 This particular study used a closed magnet high-field system, where a scan would be conducted at the discretion of the surgeon. EOTR would be measured at the time of the first intraoperative scan and at the postoperative scan. They observed an increase of EOTR from 63 to 100%, 69 to 78%, and 57 to 71% for grades I, II, and III, respectively.
Despite a lack of class I evidence, it seems plausible that there is a potential clinical benefit with the regular use of iMRI in patients with low-grade gliomas. With an observed benefit in increasing EOTR with the use of iMRI in select studies coupled with an expanding body of literature supporting a correlation of EOTR and overall survival, it seems fairly evident that iMRI can, in the right hands, increase survival in low-grade glioma patients. Given its relatively lower incidence compared with high-grade gliomas, it seems unlikely that a randomized control study evaluating the use of iMRI—or surgery in general for low-grade gliomas—will ever come to fruition. Nevertheless, the evidence does suggest a tangible benefit with the use of iMRI in treating low-grade gliomas.
15.3.2 High-Grade Gliomas
Despite advancements in treatment modalities, the prognosis of surgically treated patients with high-grade gliomas remains poor. 42 , 43 The treatment paradigm is relatively simple: maximized surgical resection with preserved neurologic function followed by adjuvant therapy. 44 , 45 , 46 As in the case of low-grade gliomas, iMRI provides resection control through real-time updates to EOTR. Updated neuronavigation can also be obtained with iMRI using an intraoperative scan and recalibration before resuming a resection. In addition, intraoperative markers of distinct anatomical structures (i.e., blood vessels) can be identified by the neurosurgeon within a resection cavity and correlated radiographic images identified by a neuroradiologist on an intraoperative scan (Fig. 15.3). This can provide useful orientation for increased resection control.
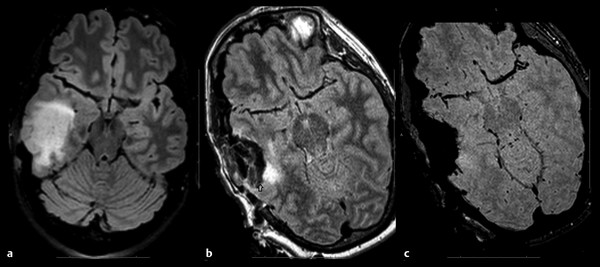
Kubben et al published a review examining the added benefit to iMRI for resection of high-grade gliomas and/or glioblastoma multiforme specifically. 47 Quantitative assessment on EOTR with the use of iMRI was one of the inclusion criteria for review. Twelve nonrandomized studies were identified with a total 439 patients. Gross total resection was one of the assessments for EOTR and was assessed in either qualitative or quantitative (through volumetric analysis) fashion (summarized in Table 15.1). Data on EOTR during the first intraoperative scan were not provided in all studies. Where it was measured, EOTR was improved across all studies between the first and final iMRI scan. Four of the studies provided survival data, one of which had a matched control group without iMRI. 48 In this particular study, although not statistically significant to the level of 95% confidence (p = 0.14), survival was increased in the iMRI versus without-iMRI cohorts with a mean survival of 14.5 months and 12.1 months, respectively. In the study by Senft et al, two cohorts were examined, one with iMRI and another using conventional neuronavigation with gross total resection intended for all patients. 49 For all patients undergoing surgery (with or without iMRI) those with gross total resection had a median survival of 74 weeks versus 46 weeks for subtotal resections (p < 0.001). In addition, patients who had surgery with iMRI had a median survival of 88 weeks versus 68 weeks for those that had surgery without iMRI (p = 0.07). In the other two studies, comparisons were done between cohorts with gross total resection versus subtotal resection. Wirtz et al showed a statistically significant improved survival (p = 0.0035) with median survival of 13.3 months versus 9.2 months. 50 Schneider et al also showed improved survival with a median survival of 537 days versus 237 days (p = 0.003). 28 At the conclusion of this review, there was at best class 2 evidence to suggest a superiority of iMRI over conventional methods for surgery on high-grade gliomas.
One of the notable points of this review was made regarding attribution bias. With the poor prognosis of high-grade gliomas, in particular glioblastoma, neurosurgeons are careful to avoid additional deficits when resecting these lesions. With the availability of iMRI, a surgeon is given leave to take a more conservative approach with the expectation of an intraoperative scan to further guide a resection. Without iMRI, a surgeon may be compelled to be as aggressive as possible, with the expectation being to maximize a resection. This bias would serve to depress the EOTR during an initial scan and would falsely elevate the potential gain in EOTR when comparing a first iMRI scan versus the final postoperative scan. In addition, although EOTR can be a useful surrogate in assessing the usefulness of iMRI, the impact of iMRI on survival would be the most meaningful assessment of its value.
At the time of this writing, there is only one published randomized, controlled trial evaluating the effect of iMRI guidance on EOTR. Senft et al published a series of 24 glioma patients randomized to surgery with iMRI and 25 randomized to conventional surgery. 51 The tumor histology was predominantly World Health Organization (WHO) grade IV glioma (glioblastoma multiforme and gliosarcoma) with two grade III and one grade I gliomas. All patients included in the study were intended to have gross total resections. In the iMRI group 96% of patients achieved total tumor removal compared to 68% in the control group. Of the patients in the iMRI group, 33% underwent further resection after the iMRI scan. At 6 months, 67% of the iMRI group had stable disease, with only 36% in the conventional group (p = 0.046). Kaplan-Meier estimates of progression-free survival suggested a median of 226 days in the iMRI group and 154 days in the conventional group; however, this difference was not statistically significant. Nevertheless, class I evidence does exist showing that iMRI can have an impact in terms of maximizing EOTR, as well as improving survival. It will be interesting to see if future studies will further corroborate these findings.
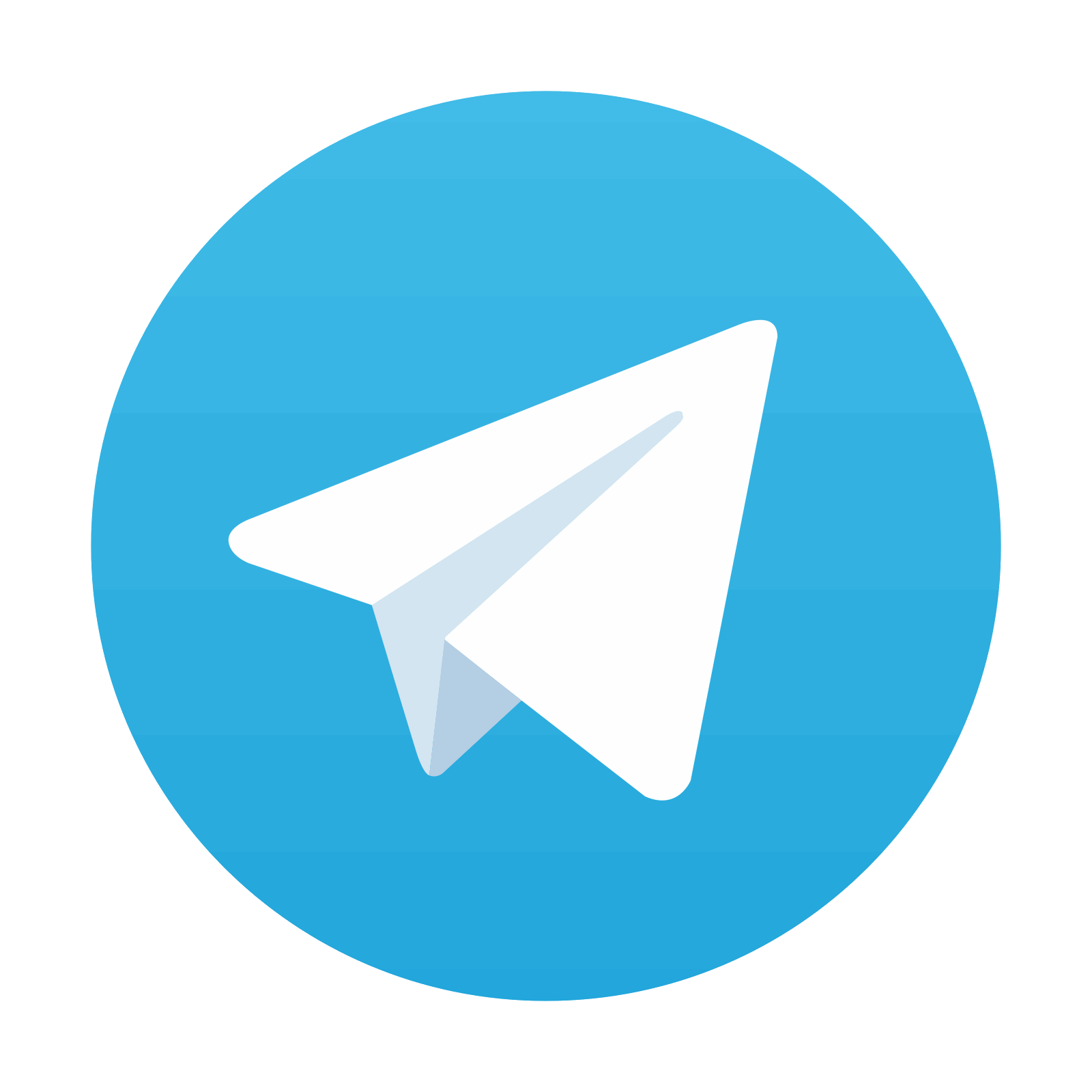
Stay updated, free articles. Join our Telegram channel

Full access? Get Clinical Tree
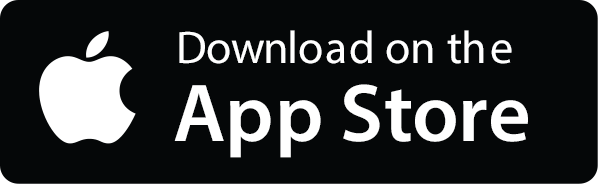
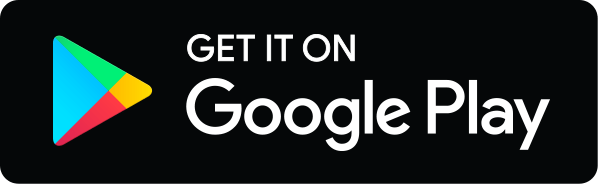
