18 Coronary Arteries and Myocardial Perfusion
Introduction
Knowledge of gross anatomy and microcirculation of the coronary arterial system is important not only to address the spatial location of a lesion but also to understand the mechanisms that lead to myocardial dysfunction. The standard clinical tool to visualize the coronary artery has been invasive cardiac catheterization and coronary angiography. Catheter angiography images have higher spatial resolution compared to recently developed noninvasive coronary computed tomography angiography (CTA). The anatomy of the epicardial coronary arteries can be shown down to third-generation (medium- to small-sized) branches by catheter angiography and to second-generation (medium-sized) branches by CTA. As opposed to projectional views in catheter angiography, CT imaging has solved the problem of overlapping vessels by generating a volume of data consisting of 0.5 mm3 voxels that can be reconstructed into 0.5-mm cuts in any desired direction. Although, the spatial and temporal resolutions of CT are lower than in invasive angiography, the noninvasive nature of the test has led to a rapidly increasing use in clinical practice. Using modern postprocessing workstations, maximum intensity projections or volume-rendered 3D images can be reconstructed. Vessel tracking, automated segmentation methods, and color-coding techniques have further improved the quality of postprocessing capabilities. In contrast to epicardial vessels, demonstration of microcirculation and tissue perfusion requires sophisticated imaging techniques such as micro-CT to show microcirculation and perfusion CT, magnetic resonance (MR) imaging, or echocardiography to evaluate tissue perfusion. This chapter will place special emphasis on typical epicardial coronary anatomy as seen in coronary CTA. Myocardial microcirculation will be discussed briefly.
Epicardial Coronary Arteries
Embryology
It was suggested in earlier experiments that coronary vessels arise from the proepicardium, a transitory structure in the embryonic heart that forms epicardium and several internal tissues. 1 Recent histological analysis in mouse and cardiac organ culture has shown that coronary vessels arise from angiogenic sprouts of the sinus venosus, the major vein that returns circulating blood to the embryonic heart. 2 Some sprouting venous endothelial cells dedifferentiate into arteries and capillaries as they invade the myocardium and some remain on the surface and differentiate into veins. 2
Gross Anatomy
Coronary arteries are variable in anatomic origin, course, and branching. 3 In general, coronary vessels are named for the structures that they supply rather than for their origin. This nomenclature is based on the embryologic development pattern that the coronary vessel sprouts arise on the surface of the heart and only later connect to the aorta. Thus, various perturbations in the connections of the coronary vessels to each other and to the aorta occur subsequent to the formation of the coronary vessels. In spite of variability in coronary artery origin, course, and branching, the vast majority of individuals have two coronary arteries: the right coronary artery (RCA) and the left coronary artery. The left main (LM) coronary artery branches into the left anterior descending (LAD) and left circumflex (LCx) arteries (Fig. 18‑1). These three major coronary vessels are considered arteries, while the secondary ramifications are generally termed coronary branches. In spite of this generalization, it is not uncommon to call some small branches “artery,” that is, sinoatrial node (SAN) artery or posterior descending artery (PDA).
Instead of bifurcating into the LAD and LCx arteries, in 10 to 15% of patients, the LM trifurcates into the LAD, LCx, and ramus intermedius arteries 1 , 3 (Fig. 18‑2). The ramus may arise directly from the LM artery (trifurcation) or near the orifice of the LAD or the LCx. Therefore, the ramus may actually be considered the first branch of the LAD or the LCx when arising from these vessels.
The relationship between the coronary artery branches at the crux cordis is called coronary artery dominance and is determined by the artery giving rise to the posterior interventricular branch/artery (PDA) 1 , 3 , 4 , 5 , 6 (Fig. 18‑3). The PDA and posterolateral (PL) branches that supply the inferior ventricular septum and the inferior wall, respectively, can originate from only the RCA (right dominant, 65–90%), from only the LCx artery (left dominant), or from both arteries (codominant). In CT studies, a “codominant pattern” is commonly described when the RCA gives off to the PDA, but all PL branches of the left ventricle (LV) and sometimes a second PDA arise from the LCx artery. The left-dominant system is more common in bicuspid aortic valve particularly when fusion is between right and left leaflets and may reach up to 48%. 7 Another definition of coronary dominance involves the artery that gives rise to the atrioventricular nodal (AVN) artery. The AVN artery arises from the RCA in almost 85% of cases.
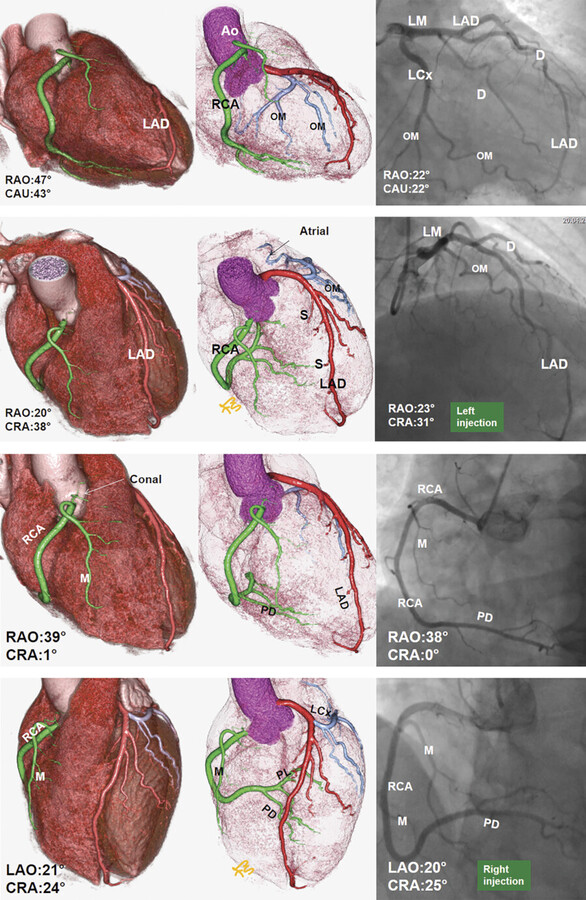
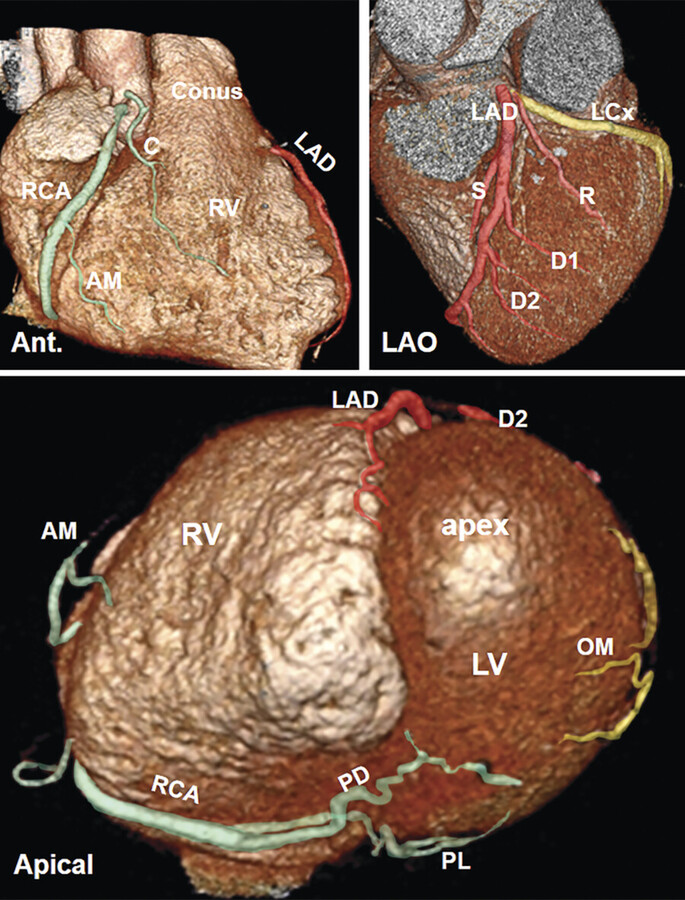
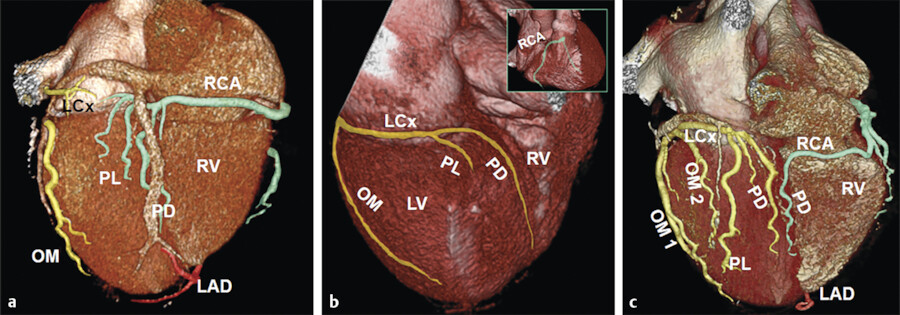
Segmental Classification
Coronary anatomy varies, and several nomenclatures have been proposed to describe the angiographic anatomy of the coronary arteries. Currently, the most commonly used classification is based on three major coronary arteries with right-dominant, balanced, or left-dominant circulations. The extent of disease is usually defined as one-vessel, two-vessel, three-vessel, or LM disease. Significant stenotic disease is the presence of a stenosis of greater than 70% diameter reduction. 8
The American College of Cardiology and American Heart Association (AHA) Guidelines for invasive coronary angiography propose a topographic angiographic model that classifies the coronary arteries into 29 segments. 9 A more simplified classification based on an Ad Hoc Committee Report of the AHA is commonly used to interpret catheter and CT angiography studies 9 (Fig. 18‑4). Obviously, this model only shows a classic pattern of coronary vessel distribution and does not involve the variability that is frequently seen in the coronary artery branches.
Diagram of the topographic distribution of the coronary anatomy segments with description of the segments are shown in Fig. 18‑4. This diagram has been modified to include the right PL branch and the ramus branches, which were not shown in the original version of this classification.
For cross-sectional imaging, the standard 17–myocardial segment model of LV is suggested by the AHA for all cardiac imaging modalities to facilitate a more detailed analysis of regional left ventricular function and perfusion 10 (Fig. 18‑5). However, the adopted model shows significant variability and overlap of these segments assigned to a specific territory that makes it difficult to compare the results between different studies (Fig. 18‑6).
The vascular supply to the inferior wall of the LV has a spectrum of variants. It is mainly supplied by the RCA and the LCx. The LAD artery that wraps around the apex can supply some of the apical inferior wall (Fig. 18‑3). The lateral wall territory is supplied by the LCx in a vast majority of individuals but can be shared by a large diagonal from the LAD or the ramus branch (Fig. 18‑6). Myocardial blood supply in the inferolateral region corresponds to LCx or RCA distribution, and the inferoseptal region can be supplied by the LAD or RCA. 15 In a correlative MR study, the presence of hyperenhancement in the basal and mid-anterior and anteroseptal or apical anterior wall has been 100% specific for LAD artery occlusion. 14 The LAD infarct frequently involves the mid-anterolateral, apical lateral, and apical inferior walls. No segment is 100% specific for the RCA occlusion. A combination of hyperenhancement in the anterolateral and inferolateral walls is 100% specific for an LCx occlusion, and, when extended to the inferior wall, is also 100% specific for a dominant or codominant LCx occlusion. Hybrid imaging combined with anatomic information of vascular distribution provided by CTA is useful to study myocardial tissue perfusion abnormalities and the coronary anatomy for more accurate assignment to culprit vessels and to improve monitoring of targeted therapy. 11 CT studies have shown common overlap between LAD and RCA perfusion territories at the inferoseptal region and between LAD and LCx territories at the anterolateral region. It is important to realize that the LAD territory can be larger than the AHA-proposed 17-segment model, due to the involvement of the anterolateral and all the apical segments of the LV. 15
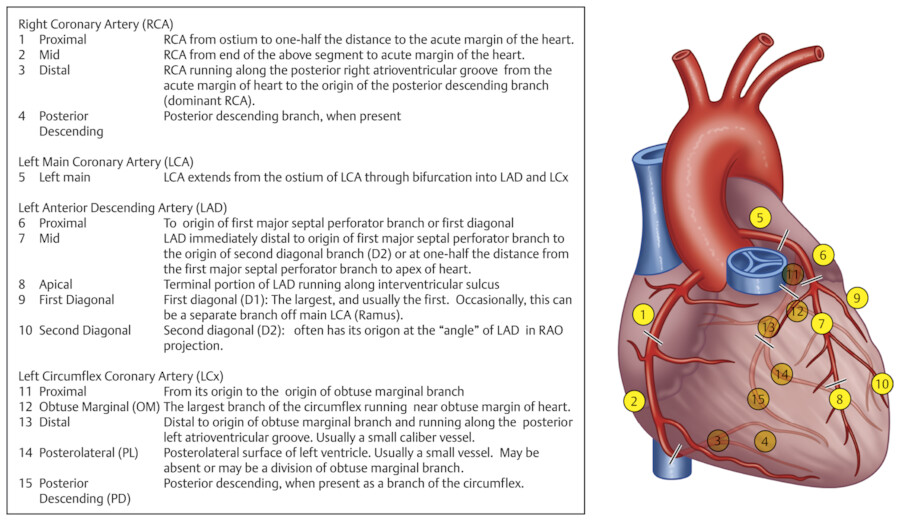
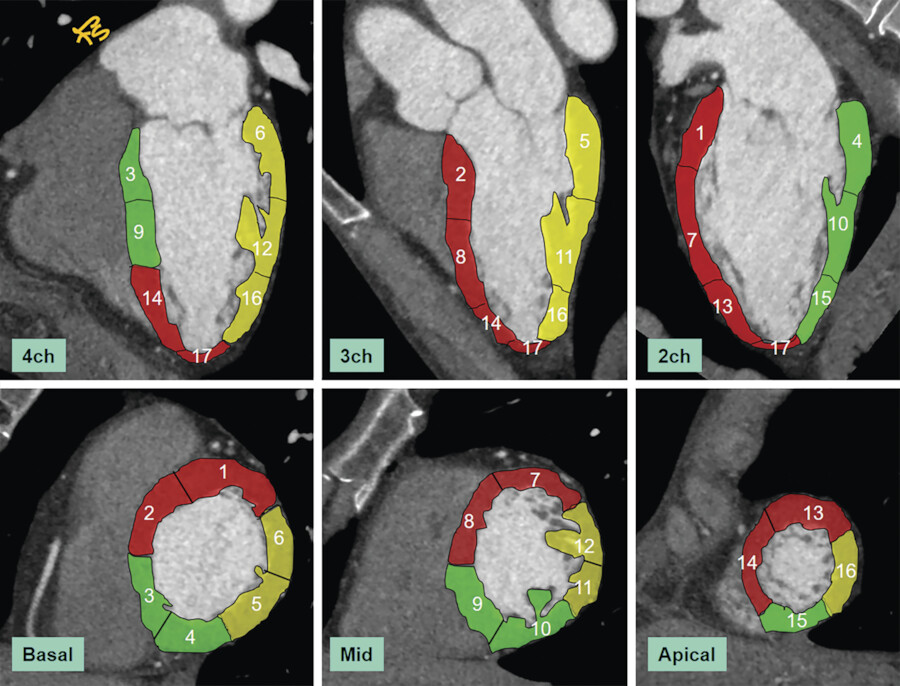
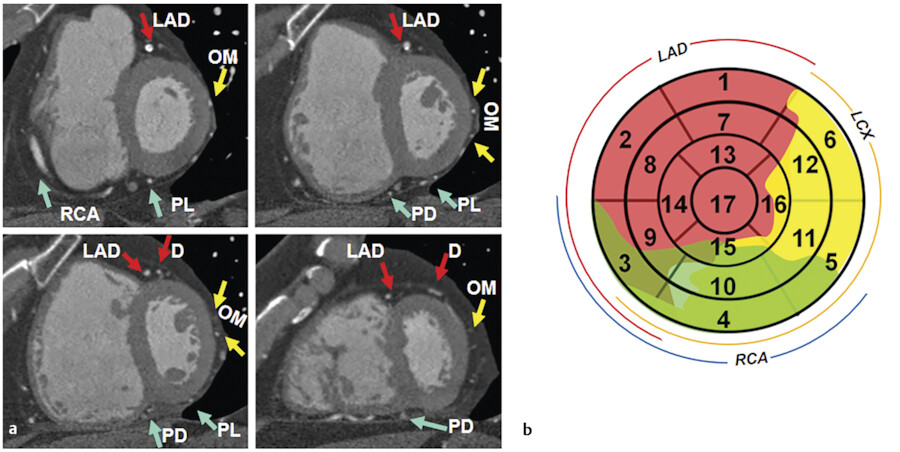
Common Variants
Left Coronary Artery
The LM coronary ostium is located in the midportion of the left sinus of Valsalva with a mean distance of 14 ± 3 mm from the annulus. It measures approximately 8 mm in diameter and travels for 5 to 15 mm before dividing into the LAD and LCx arteries. The mean bifurcation angle between the LAD and LCx is 80 ± 23 degrees 12 (Fig. 18‑7 , Fig. 18‑8 , Fig. 18‑9). Knowing the angle of bifurcation may be important for optimal stent deployment.
Anatomical variants of the LM are common. The ostium of the LM artery may arise at or above the sinotubular junction. When it arises at the sinotubular, the ostium may be small. A high origin the LM may be prone to accidental cross clamping or transection during aortotomy (Fig. 18‑10). A very low position ostium may become obstructive after percutaneous aortic valve implantation. A very short LM artery can cause technical problem during stenting of the LAD stenosis at its takeoff. Separate origins of the LAD and LCx in left sinus of Valsalva are another relatively common variants (Fig. 18‑11). The incidence is more common in bicuspid aortic valve, especially with left–right fusion and may reach up to 28%. 7
The LAD passes behind the main pulmonary artery to enter the anterior (superior) interventricular groove (sulcus) where it travels to reach the apex (Fig. 18‑7 , Fig. 18‑9). It gives rise to the perforating septal and one to three diagonal branches with viable sizes to supply the anterior two-thirds of the septum and the anterolateral wall of the LV including the superolateral papillary muscle, respectively (Fig. 18‑9). Small branches to the anterior wall of the right ventricle (RV) or the RV infundibulum may arise from the LAD. The distal segment of the LAD usually ends up near the apex, but it may wrap around it and travel further in the inferior interventricular groove. In rare cases, it may reach the cardiac crux (Fig. 18‑3 a).
A confusing variant of the LAD is called dual LAD. Type I dual LAD is worth mentioning because it is relatively common and may have clinical implications. In this variant, the LAD trunk is bifurcated (split LAD) into a short and a long branch to give off septal perforators. The short LAD stays in the proximal anterior interventricular groove branching into several septal perforators (usually 3–4; Fig. 18‑12). 17 This artery should not be confused with a large first perforating septal branch during catheter angiographies, which may need to be embolized for treatment of obstructive hypertrophic cardiomyopathy.
The LCx or circumflex artery arises from the LM coronary artery. It then passes under the left atrial appendage, to reach the left AV groove (Fig. 18‑7 , Fig. 18‑9). At this level, small atrial branches including a left SAN artery may arise from the LCx (Fig. 18‑13). The position of the LCx in relation to the AV groove is variable. The LCx gives off one or more obtuse marginal (left marginal) branches to supply the lateral and posteroinferior walls of the LV. It is not uncommon to see a small LCx to continue in the AV groove after giving off to a large obtuse marginal branch. The distal LCx can travel in the AV groove to reach cardiac crux. At the AV groove, it could be damaged during mitral valve surgical or percutaneous interventions. When dominant, the LCx gives rise to the posterior interventricular artery (Fig. 18‑3).
Myocardial bridging can be found in any epicardial artery, but greater than 60% occurs in the proximal LAD artery or one of diagonal branches (Fig. 18‑14). It refers to the intramyocardial course of a portion of a normally positioned epicardial coronary artery. The degree in which the coronary artery travels into the myocardium is variable in depth and length. The bridged segment is usually protected from atherosclerotic diseases and calcifications, but plaques can form proximal to it. 16 The significance of transient systolic narrowing (milking effect) of the bridged segment is not clear since most coronary blood flow occurs in diastole. The hemodynamic impact of myocardial bridging depends on the thickness and length of the bridge and rarely may be associated with exertional angina and acute coronary syndromes.
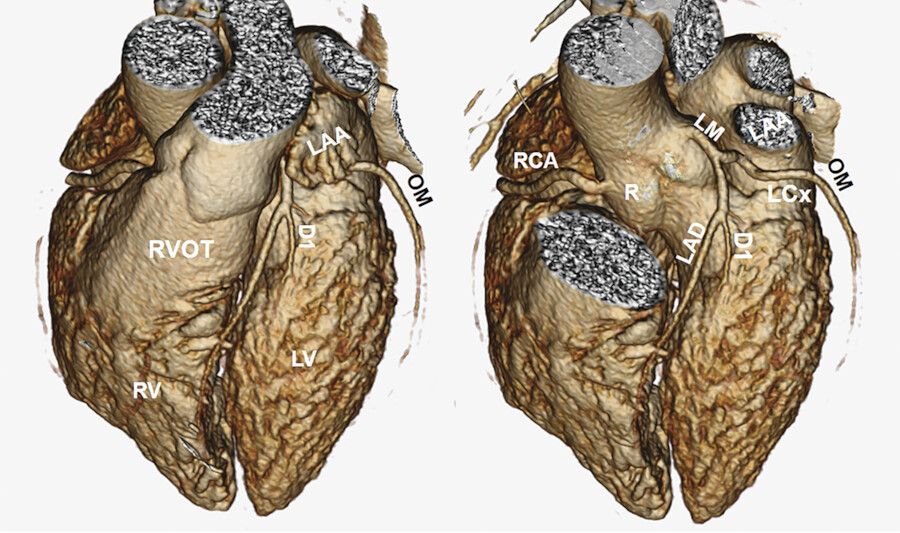
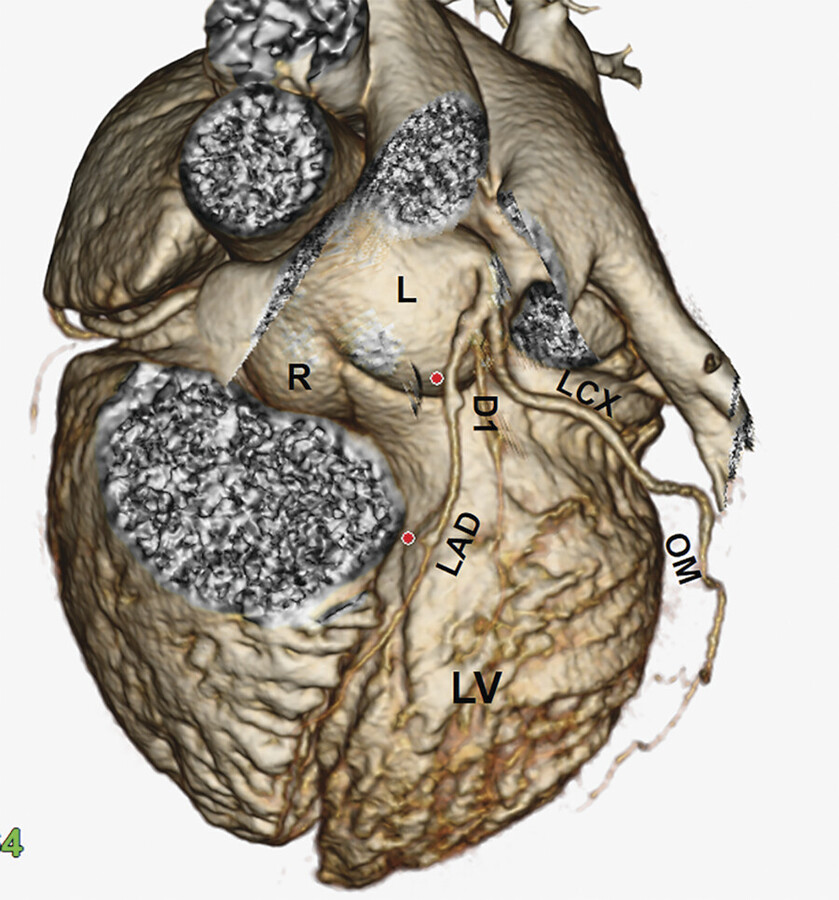
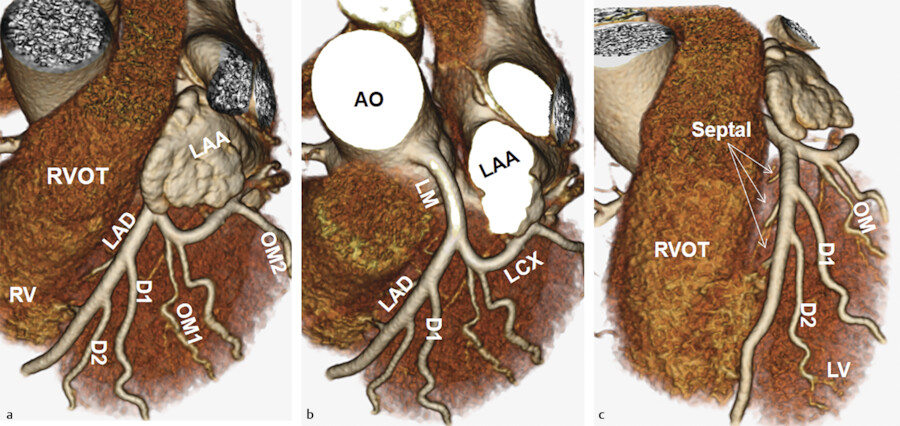
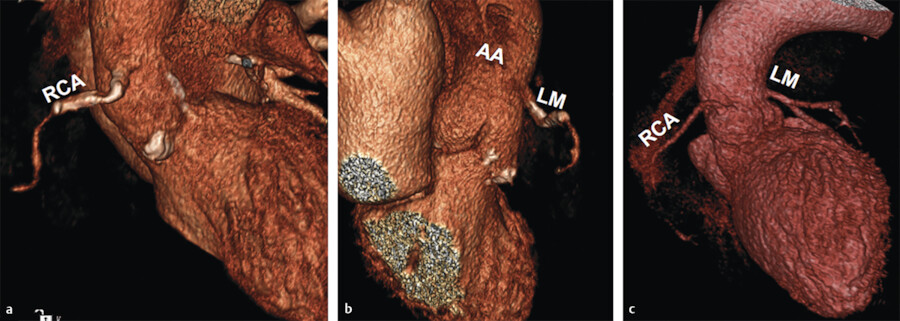
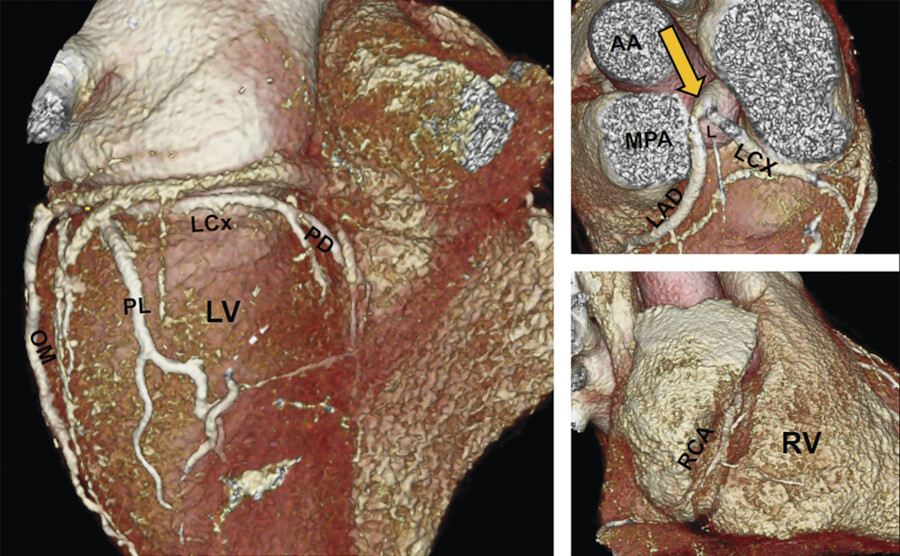
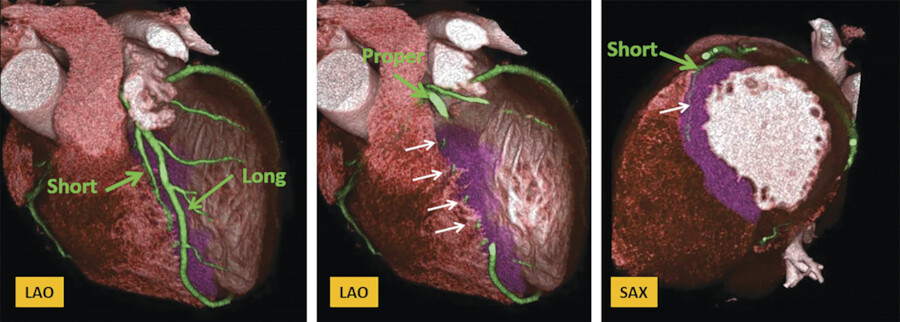
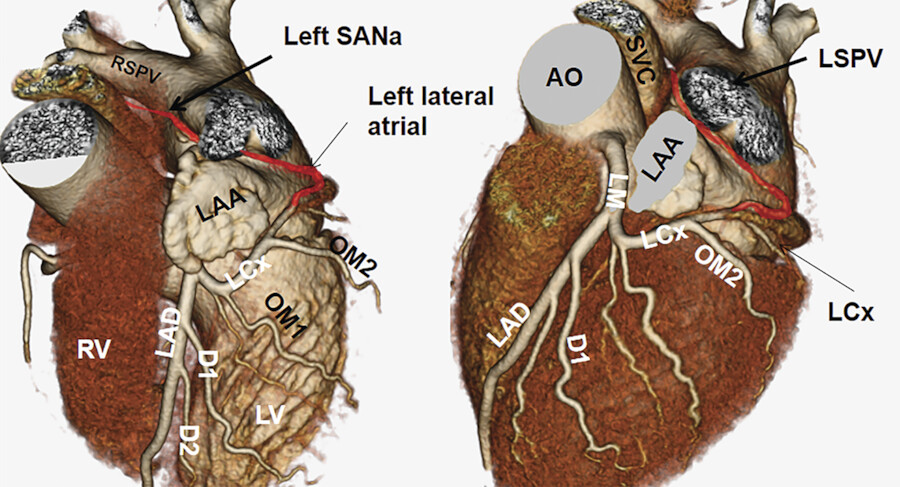
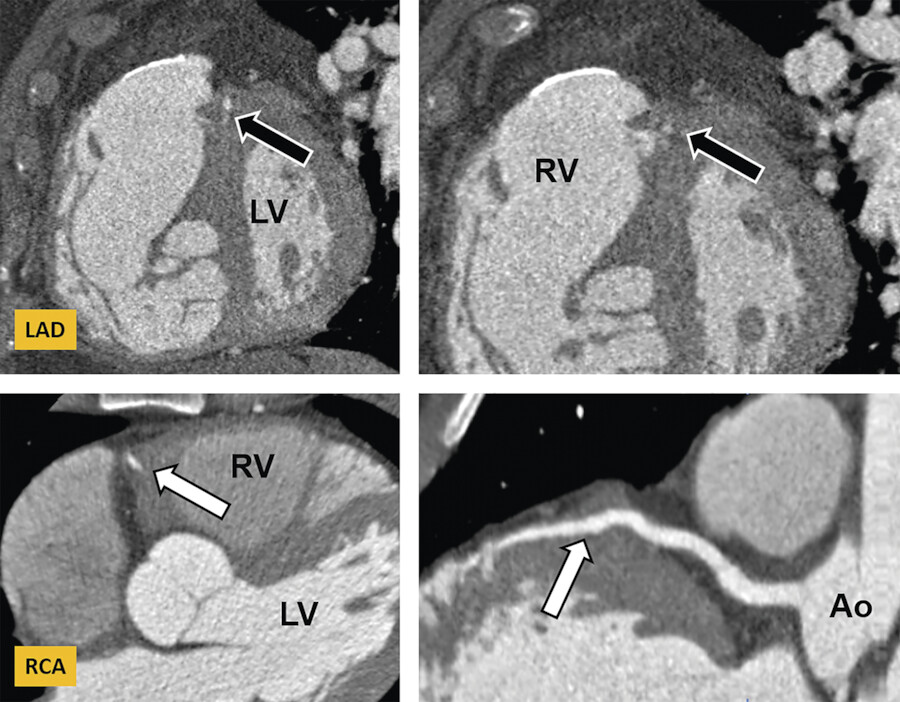
Right Coronary Artery
The RCA arises from the right coronary sinus (Fig. 18‑15). Mean distance between the aortic annulus and the ostium of the RCA is 17 ± 3 mm. 13 There is remarkable variability in the branches of the proximal RCA or of the right aortic sinus close to the coronary ostium. The five arteries that may arise from the first 2 cm of the RCA include an anterior conal artery, a right SAN artery, an adventitial artery (vas vasorum) of the aortic root, a preventricular artery, and occasionally a right superior septal artery (right Kugel’s; Fig. 18‑16 , Fig. 18‑17). The conus, SAN, and superior septal arteries can arise with a separate ostium directly from the right coronary sinus, with the right conus artery being the most common variant. In its proximal course, the RCA enters the right AV groove and travels in it toward the posterior part of the interventricular groove to reach the crux of the heart. At this point or some time earlier, the RCA bifurcates into two branches: the posterior-descending branch and the PL branch(s) (Fig. 18‑3).
The PDA travels in the inferior (posterior) interventricular groove toward the apex of the heart. The right PL branch/branches supply the PL wall of the LV. These branches are usually small but can be large when the RCA is super-dominant and the LCx is diminutive (Fig. 18‑18). One or two marginal (acute marginal) branches arise from the mid-portion of the RCA to supply the RV free wall.
Variation in the course of middle and distal segments of the RCA is uncommon. A segment of the RCA may move outside the atrioventricular groove, dive into the myocardium (bridging; Fig. 18‑14), or enter the cavity of the right atrium (intracameral). 18
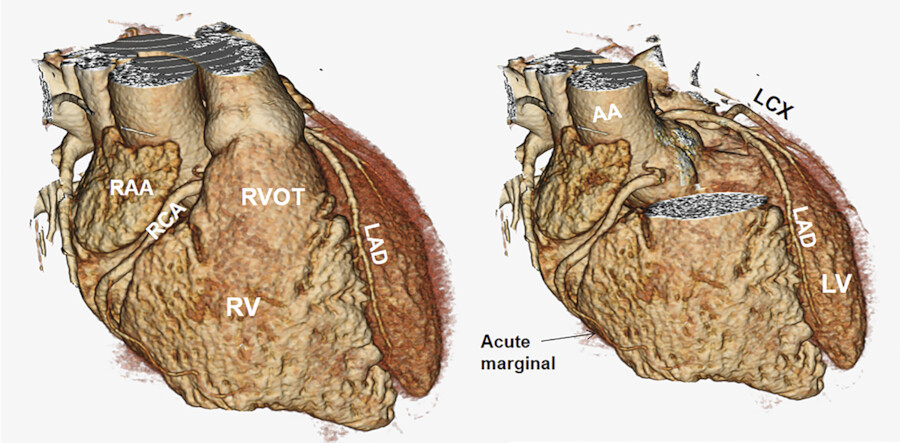
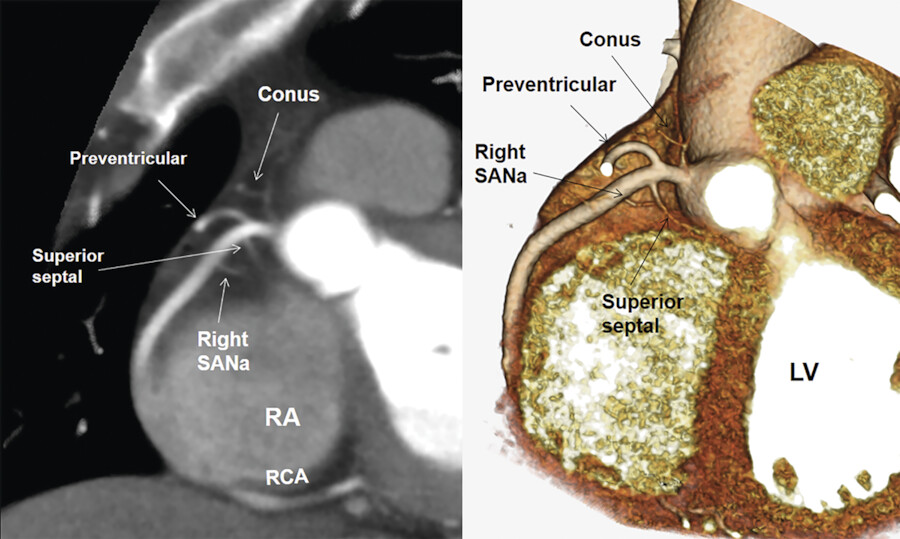
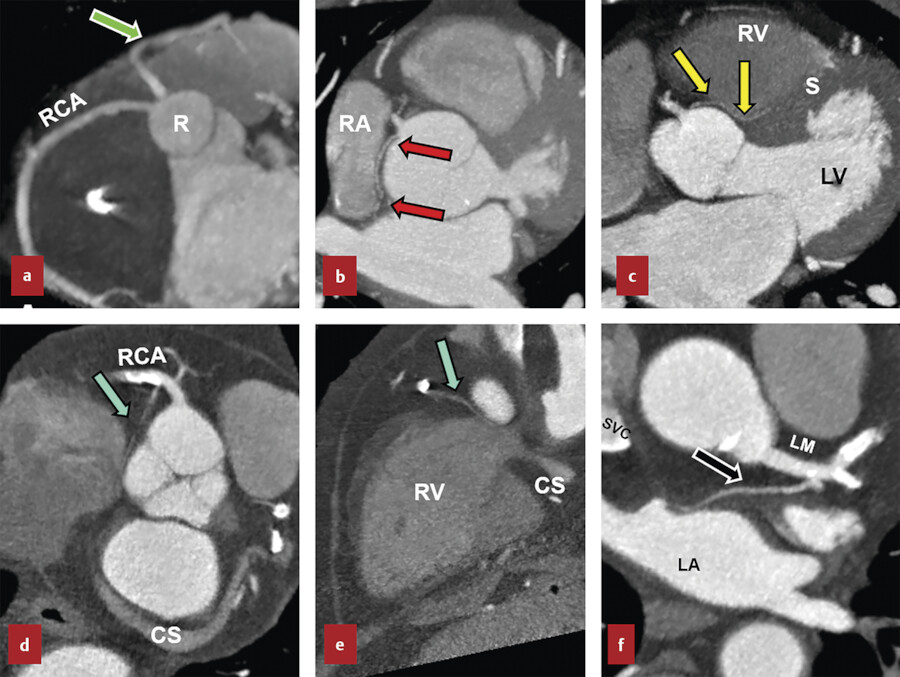
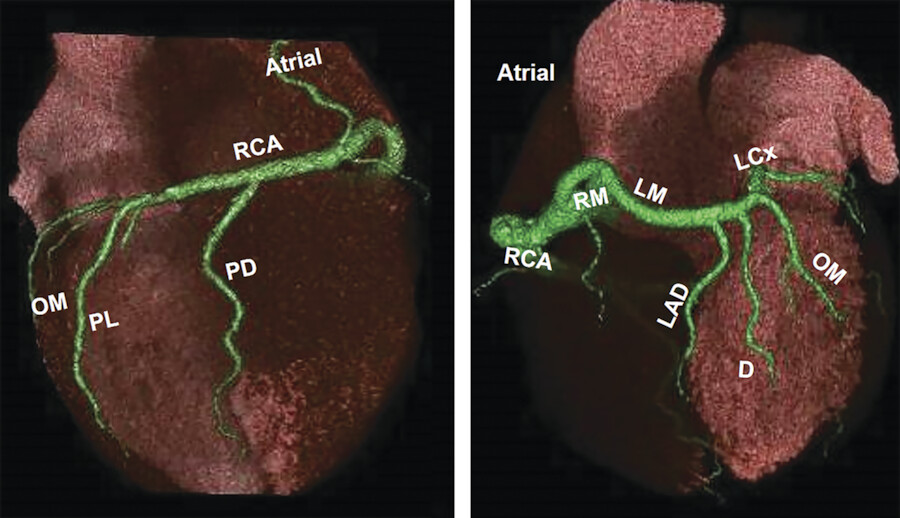
Posterior Descending Artery
Evaluation of the anatomical origin and course of the PDA is important in angiographic studies of the coronary arterial system. The PDA determines the coronary dominance. In most patients, the PDA arises from the distal portion of the coronary artery, but in some cases an early takeoff of the PDA from the RCA is seen, which then courses toward the apex along the diaphragmatic surface of the RV. This common variant is referred to as “split RCA” 17 , 19 (Fig. 18‑19). The splitting is usually proximal and leads to one branch with normal anatomical course in the right AV groove, giving rise to a PDA that supplies the proximal (basal) portion of the inferior interventricular groove, and a second branch that courses over the right ventricular free wall (acute marginal), and terminates in the distal part of the inferior interventricular groove as the distal PDA. 17 Other unusual variants include duplicated PDA or a single PDA that immediately bifurcates in two equal-sized branches (split PDA) running along the sides of the inferior interventricular groove (Fig. 18‑20). Split-RCA and dual-PDA cases appear to be benign and do not warrant a special management, except in the presence of atherosclerotic stenoses, which can make cannulation challenging during percutaneous coronary intervention. 20
In the codominant (balanced) coronary system, branches from both the LCx and RCA share vascular supply to the inferior interventricular septum. 5 , 6 In other words, a “dual perfusion” exists that provides blood flow to both sides of the septum (Fig. 18‑3 , Fig. 18‑21). Therefore, using the perfusion concept, several vessel arrangements exist that can fit in the definition of a codominant coronary system. In patients presenting with an acute coronary syndrome, left coronary dominance appears to be associated with increased long-term mortality, especially with involvement of the inferior wall of the heart. 21 In most individuals with left coronary dominance, the RCA is usually a small and unreliable source of left myocardial perfusion.
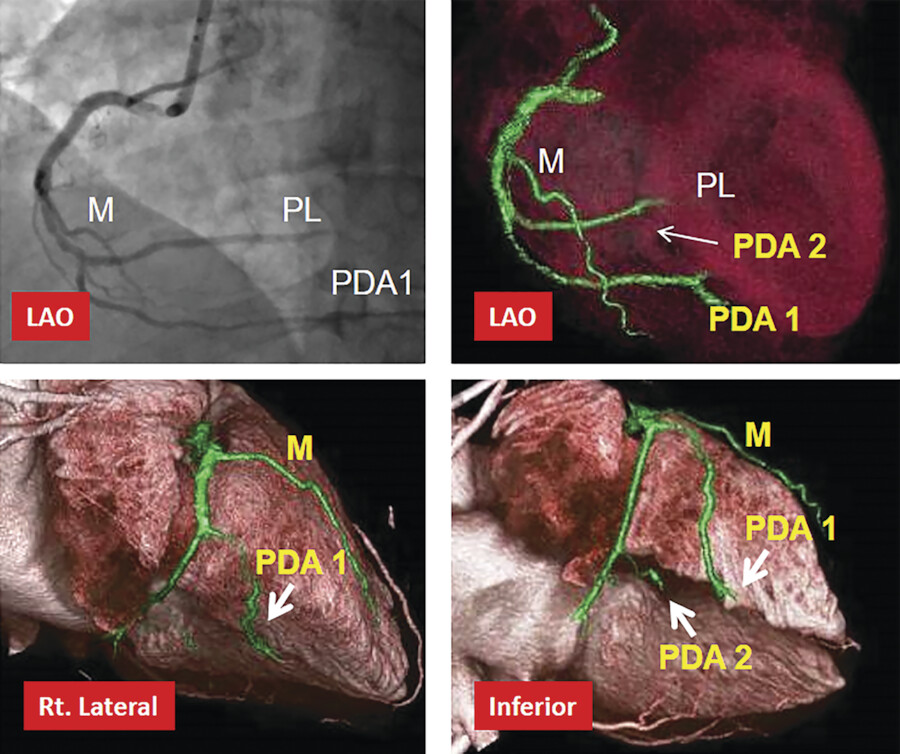
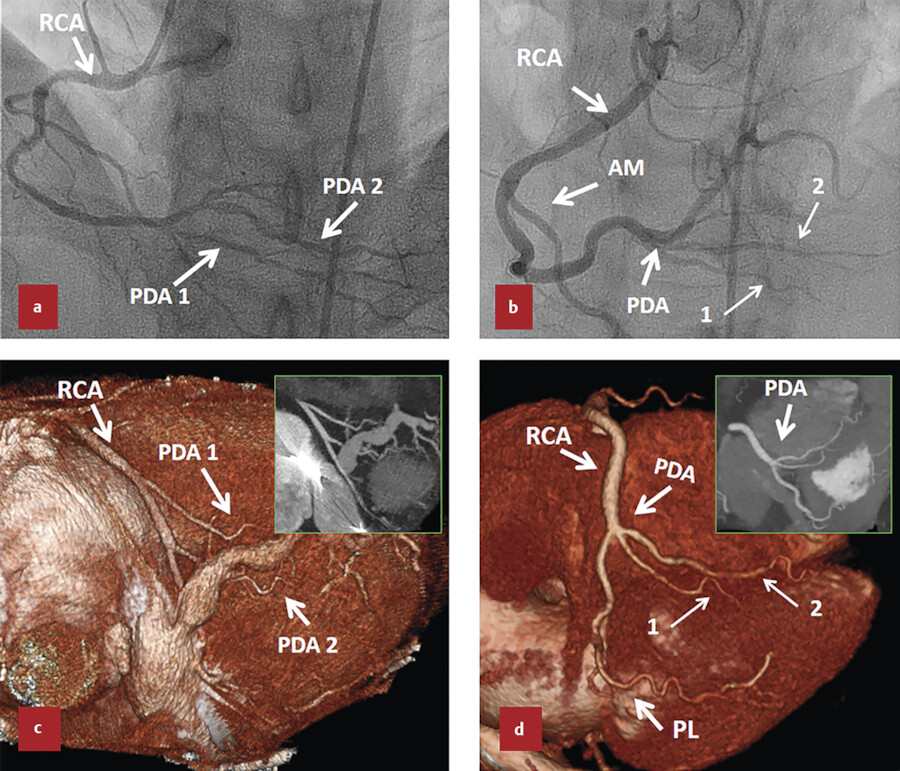
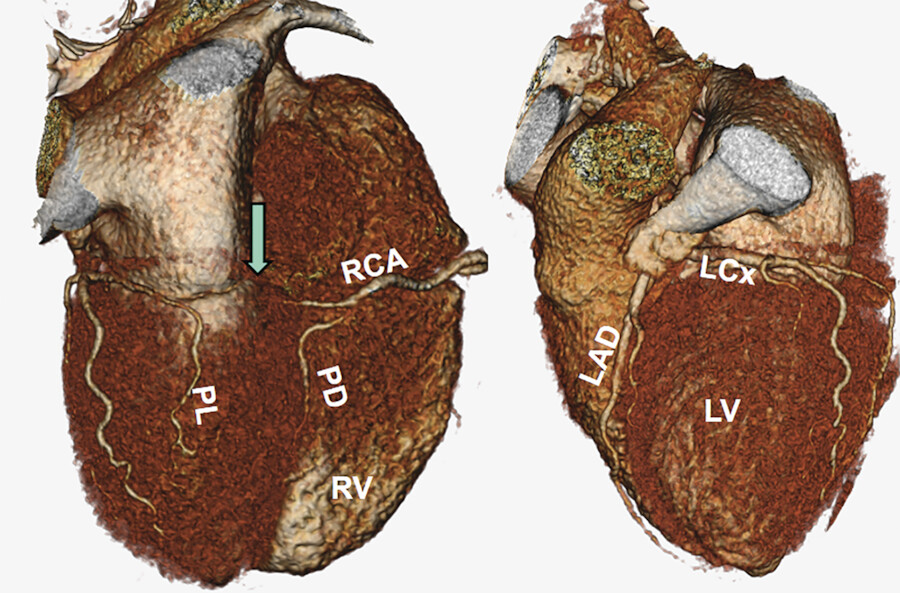
Participants of Intercoronary Connections
Collaterals are interarterial connections that provide blood flow to a vascular territory whose original supply vessel is obstructed (acquired or congenital). The interarterial collateral connections are small with a wide range, between 40 and 200 µm, which may reach up to 1 mm. 22 The size of the majority of these connections is below the 0.2-mm spatial resolution of most angiographic imaging equipment. The spatial resolution of most current CT scanners is above 0.4 mm. In case a major artery becomes occluded (>90% stenosis), because of increased pressure through these small anastomotic vessels, they dilate and become visible by imaging methods.
Common sources for inter- and intracoronary collaterals include (1) peripheral branches of the right and left coronary arteries, (2) transseptal anterior and posterior septal perforators, (3) conotruncal anastomotic pathways, (5) distal LAD with PDA, (6) interatrial anastomotic pathways, and (7) intramyocardial pathways (Fig. 18‑22 , Fig. 18‑23).
The most common types of extracardiac aortocoronary collaterals are those between bronchial and coronary arteries and between the internal thoracic and coronary arteries 23 , 24 (Fig. 18‑24). The connections are through the sites of pericardial reflections, which allow the collaterals to enter the subepicardial layer. Extracardiac collaterals are occasionally seen between the SAN artery and the mediastinal or bronchial arteries in chronic pulmonary and bronchial diseases (Fig. 18‑23).
Collateral flow may protect myocardium from transmural infarction and preserve wall function when a vessel is totally occluded. This can occur in congenital absence or acquired obstructions (Fig. 18‑23). Collateral after infarction usually occurs within weeks after occlusion or severe stenosis with a great inter-individual variability in the potential to recruit collateral vessels. 22 Collaterals will regress once the ischemic territory is revascularized. 25
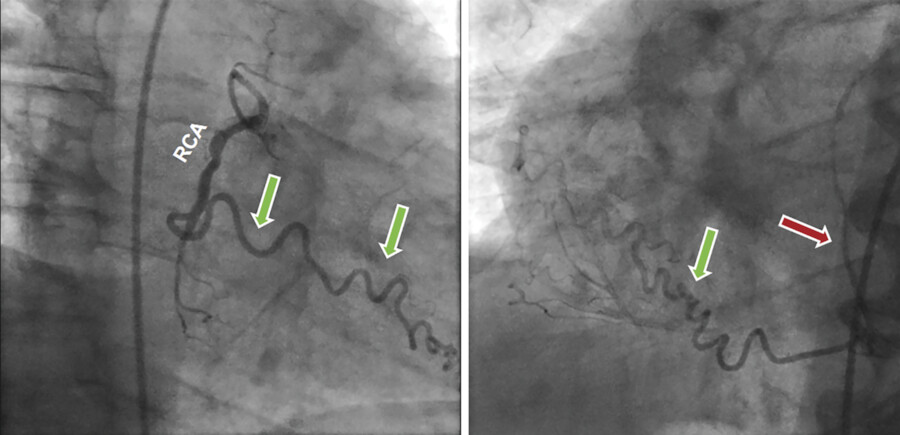
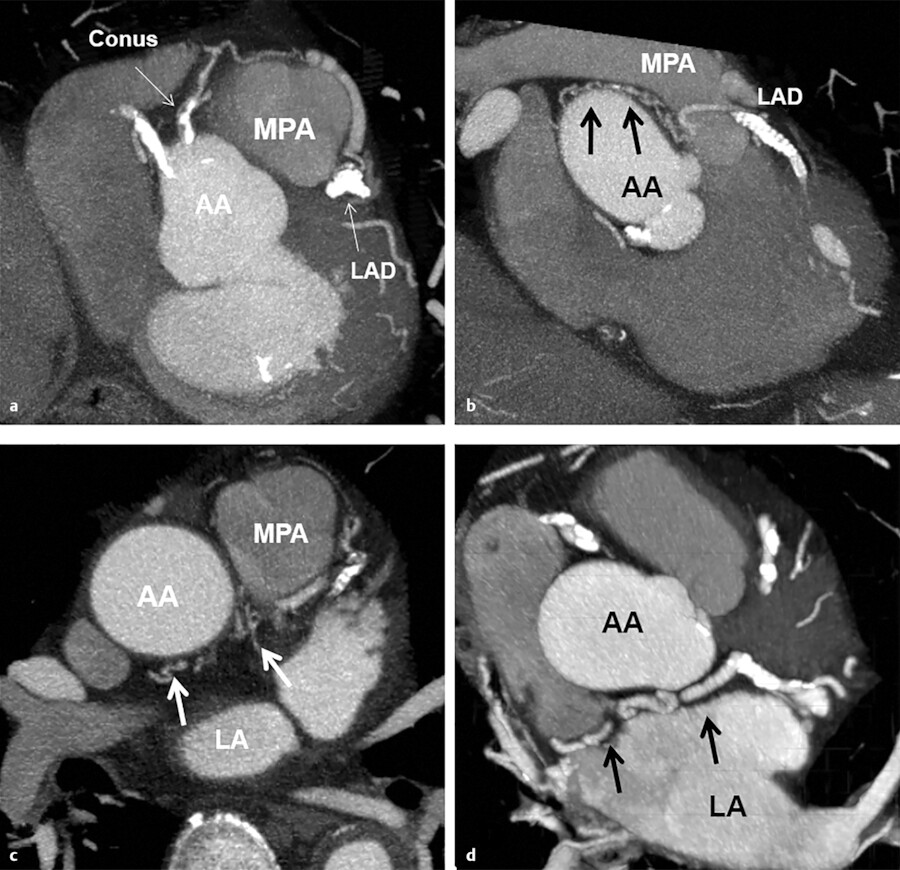
Interventricular Septum
Two groups of arteries are responsible for blood supply to the interventricular septum. These perforating septal branches are divided into the anterior and posterior septals. This nomenclature to define the anatomical position of these vessels is based on their relation within the heart. Within the thorax, however, the positions can more accurately be described as superior and inferior. 26
The anterior septal branches arise from the LAD and the posterior septal branches arise from the PDA (Fig. 18‑9 , Fig. 18‑12). They travel within the septum close to the right ventricular endocardium (Fig. 18‑12). The anterior perforating septal arteries are significantly larger and more numerous than their inferior counterparts. The first branch is the largest in 80% of cases. The number of superior septal arteries ranges from 1 to 10, with an average of 4.2, and the inferior one’s ranges from 0 to 7, with an average of 2. 26 The septal arteries are the basis of efficient intercoronary collateralization between the LAD and PDA and probably the most common type. 25
The first septal perforating artery is a branch of LAD artery that originates between 13 and 37 mm from the LAD ostium and has a diameter of 1 mm (Fig. 18‑25). The artery branches into three smaller ones. The main branch passes toward the medial papillary muscle and the other two passes into the septomarginal trabeculation. 26 When the medial papillary muscle is present, the first septal artery tends to pass toward the base of the medial papillary muscle. The artery supplies the base of the ventricular septum and the right ventricular side of the septum including the moderator band with branches to the conduction system including the bundle of His and proximal bundle branches. In hypertrophic cardiomyopathy, the artery is enlarged. Embolization of this artery is commonly performed as a way to treat left ventricular outflow tract obstruction seen in some cases of hypertrophic cardiomyopathy. 27 The source of the moderator band artery is one of the first three anterior septal arteries, with the second septal being the most common. This artery can extend to the anterior papillary muscle where it may anastomose with the marginal branches of the RCA. 28
The right superior descending (septal) artery is seen in 3 to 6% of angiography studies arising from the proximal RCA 29 , 30 (Fig. 18‑25). It could be a potential intercoronary collateral connecting the proximal RCA to the distal RCA, LCx, or LAD through the atrial anastomotic network, retroaortic anastomotic pathway or trans septal connections (Fig. 18‑26; see the following sections).
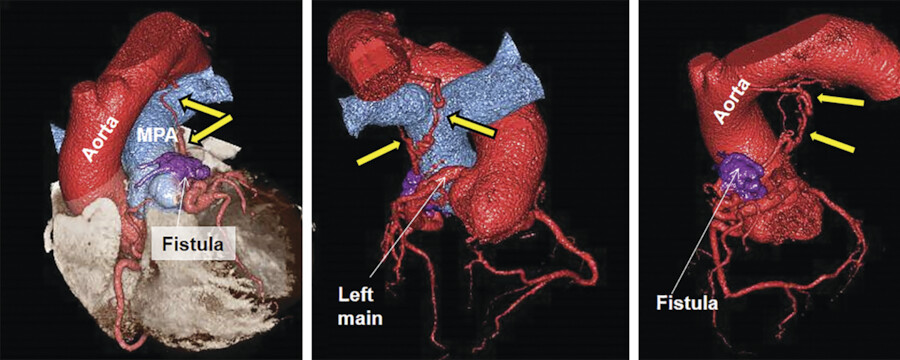
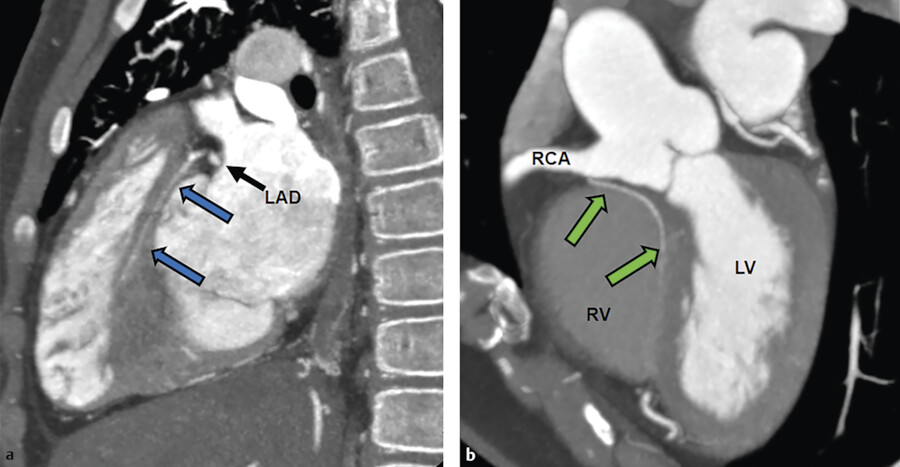
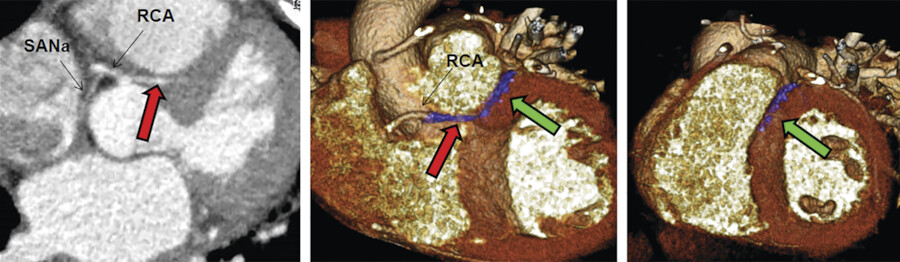
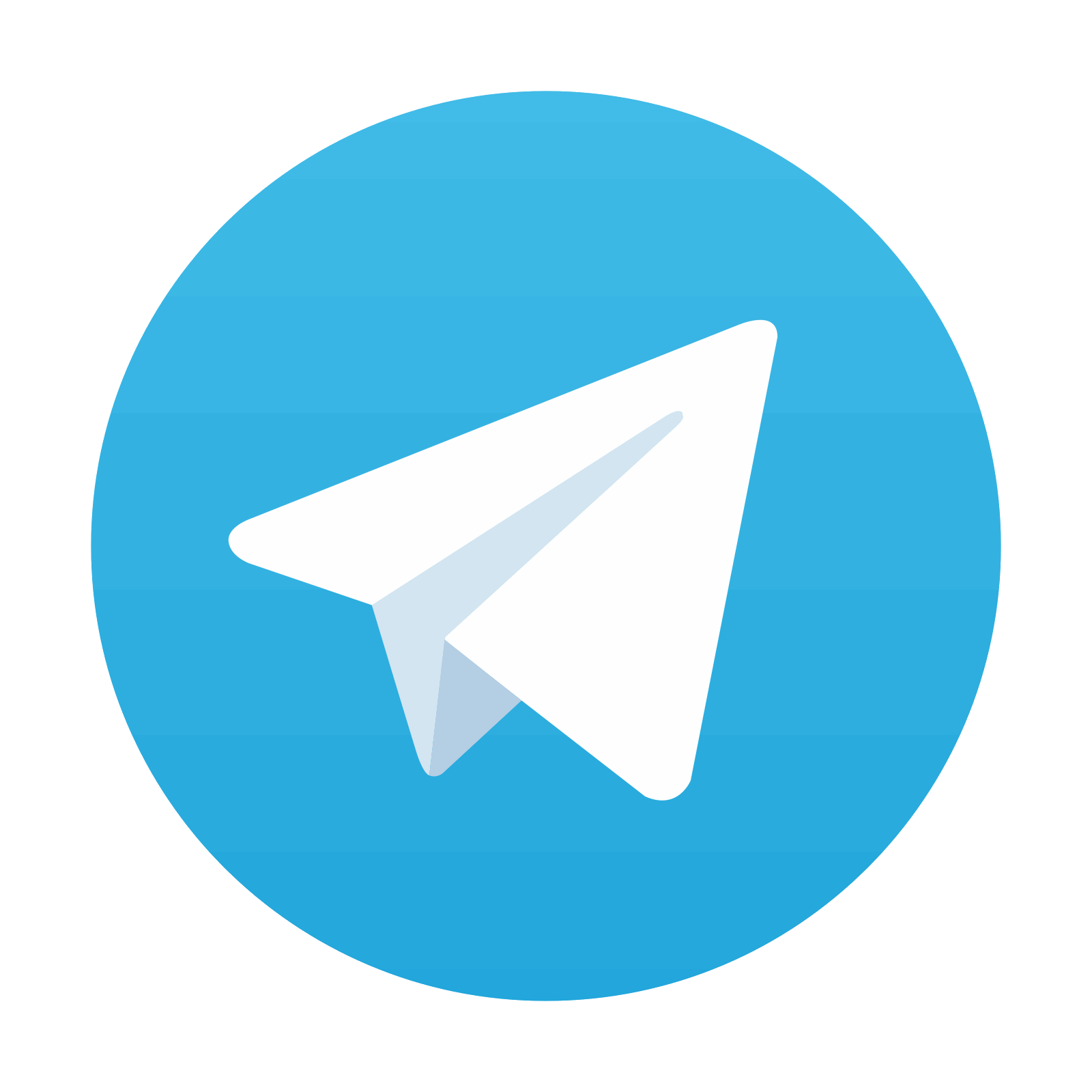
Stay updated, free articles. Join our Telegram channel

Full access? Get Clinical Tree
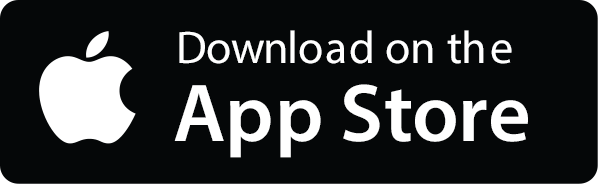
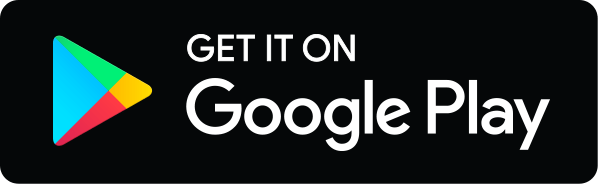