23 Yttrium-90 Radioembolization of Malignant Liver Tumors
Christopher A. Molvar and Robert J. Lewandowski
23.1 Introduction
The liver is a frequent site of metastatic disease, often of colorectal origin (metastatic colorectal cancer [mCRC]), and is also a site of lethal primary malignancy. Hepatocellular carcinoma (HCC) is the most common primary hepatic tumor and the third most common cause of cancer mortality. Surgery offers a cure for primary and secondary hepatic tumors; however, many patients are not candidates for surgical resection, and so palliative treatments dominate. Hepatic yttrium-90 (90Y) radioembolization is an evolving palliative treatment option offering new promise to patients with often limited treatment choices. As with other transarterial therapies, radioembolization relies on the dual blood supply of the liver. More specifically, malignant tumors are primarily supplied by the hepatic artery, whereas the background nontumorous liver is primarily supplied by the portal vein. Therefore, radioembolization delivers high-dose internal radiation to the tumor while sparing much of the nontumorous liver. This chapter will review patient selection, technique, and outcomes of hepatic radioembolization for primary and select secondary tumor types.
23.2 Patient Selection and Indications
90Y radioembolization is a potent treatment option for patients with unresectable liver-dominant disease who have preserved liver function and performance status. Treatment goals are varied but may include improved survival and improved quality of life, including symptomatic relief. This technique may also be used for neoadjuvant applications.
Radioembolization is currently performed with small microspheres that deliver yttrium-90, a beta-emitting isotope. These microspheres emit high-energy, low-penetration radiation (approximately 2.5 mm) to the tumor while sparing the surrounding liver parenchyma. Pathologic studies after radioembolization have demonstrated a relative 50- to 70-fold greater microsphere density at the tumor periphery compared to the density in the background liver parenchyma. 1
Two radioactive microspheres are commercially available; these microspheres differ in the type of 90Y carrier used. Thera-Sphere (BTG International, London, UK) is a glass microsphere that is 20 to 30 microns in size and has a high specific activity (2500 Bq per sphere) at calibration. SIR-Spheres (Sirtex Medical, Sydney, Australia) are resin microspheres similar in size to TheraSphere microspheres but with a lower specific activity (50 Bq per sphere). Despite these technical differences, response rates for hepatic tumors treated with these two types of microspheres appear to be equivalent. TheraSphere was approved in 1999 by the Food and Drug Administration (FDA) under a Humanitarian Device Exemption for the treatment of unresectable HCC, 2 and the SIR-Spheres microsphere was granted approval in 2002 for the treatment of mCRC. 3
For HCC, patient eligibility parallels eligibility for chemoembolization, including unresectable liver-dominant disease with preserved liver function (Child–Pugh class A or B) and performance status (Eastern Cooperative Oncology Group [ECOG] score, 0–2). The microembolic nature of radioembolization also allows for its use in patients with portal vein thrombosis (PVT), thus limiting the risk of ischemic hepatitis. 4
The fundamental patient selection criteria for radioembolization in secondary liver tumors match those described for HCC. Eligible patients typically have unresectable liver-dominant tumor after standard-of-care chemotherapy. Some patients require palliative therapy for painful bulky tumors. Many modern chemotherapeutic regimens are associated with liver toxicity, including chemotherapy-associated steatohepatitis (seen with irinotecan) and veno-occlusive disease (seen with oxaliplatin). As such, preservation of hepatic function is critical to patient selection. Biologic anticancer agents such as bevacizumab make the vasculature more prone to injury. Recent use of these agents increases the risk of vascular dissection and rupture, which can prevent the use of transarterial treatment. Careful microcatheter and microwire manipulation are required for patients exposed to systemic anticancer therapies. 5
Special considerations are made for patients lacking a competent sphincter of Oddi, often because of the presence of bilioenteric anastomosis or a biliary stent. Hepatic artery embolization in this setting is known to markedly increase the risk of hepatic abscess. 6 Recent evidence suggests that a prolonged course of antibiotics and the use of radioembolization rather than chemoembolization may lower the risk of hepatic abscess in these patients. 7 , 8
23.3 Contraindications
Appropriate patient selection is paramount to limiting adverse radioembolization outcomes. Regardless of tumor type, maintenance of performance status is critical. An ECOG score greater than 2 predicts poor treatment outcomes and represents a relative contraindication to therapy. Similarly, patients with extensive and progressive extrahepatic tumor should not undergo treatment. Cases in which more than 75% of the liver parenchyma has been replaced by tumor are at significant risk of hepatic decompensation. If treatment of these patients is undertaken, it should be carefully staged with sublobar administrations. Moreover, cases of advanced cirrhosis and liver dysfunction, as seen with Child–Pugh class C patients, are typically not eligible for embolization because of competing risk of death from liver failure. Liver function tests should be within normal limits for patients with hepatic metastasis, unless the majority of the liver is replaced by tumor. 5 Prior exposure of the liver to external beam radiation may lead to increased toxicity with radioembolization. 9
Patients clinically eligible for radioembolization undergo planning angiography and 99mTc-macroaggregated albumin (MAA) administration. This may reveal absolute contraindications to radioembolization such as uncorrectable hepatoenteric arterial communications and significant hepatopulmonary shunting. Nontarget radiation can lead to gut ulceration (sometimes necessitating surgery), pancreatitis, and radiation pneumonitis. The lungs can tolerate 30 Gray (Gy) per treatment and 50 Gy cumulatively. 10 Additional caution should therefore be exercised when treating patients with underlying pulmonary disease.
23.4 Technique
Before a patient is treated with radioembolization therapy, planning angiography with MAA administration is performed to delineate tumor perfusion and quantify arterial-venous shunting. Meticulous power-injected digital subtraction angiography (DSA) of the visceral and hepatic vasculature is essential. An abdominal aortogram should also be considered if recent cross-sectional imaging did not include an arterial phase; this will aid in visceral catheter selection and identification of extrahepatic tumor supply. The superior mesenteric artery should be assessed for evidence of possible hepatic perfusion, such as a replaced or accessory right hepatic artery or retrograde flow in the gastroduodenal artery (GDA) as is seen with hemodynamically significant celiac stenosis and tumor sump. Delayed images can be used to identify portal vein patency and flow dynamics (hepatopetal). The celiac artery should be catheterized to assess hepatic and intestinal arterial flow, and the common and proper hepatic arteries should be evaluated, along with branch supply to the left and right lobes of the liver. The use of microcatheters and high contrast injection rates (2–3 mL/s for 8–12 mL) is compulsory. It is critical to identify the position of the gastroduodenal and right gastric arteries, the latter of which often arises from the proximal left hepatic artery. Depending on flow dynamics and the intended site of 90Y microsphere infusion, embolization of branch vessels supplying the intestine should also be considered to prevent nontarget microsphere deposition. Early in an operator′s experience, aggressive prophylactic embolization of such branch vessels is commonly employed. Embolization should include the vessel origin to minimize the risk of collateral formation and recanalization, especially if staged therapy is planned. The incidence of gastroduodenal ulceration after radioembolization ranges from 0.7 to 28.6%, with a weighted mean incidence of 4.8%. 11 With experience, routine prophylactic coil embolization of the GDA and right gastric artery can be reconsidered, especially when glass microspheres are used. 12 The cystic, supraduodenal, falciform, accessory left gastric, and right/left inferior phrenic arteries should also be identified (▶ Fig. 23.1). These vessels can be embolized to prevent nontarget embolization or to redistribute therapeutic flow. 13
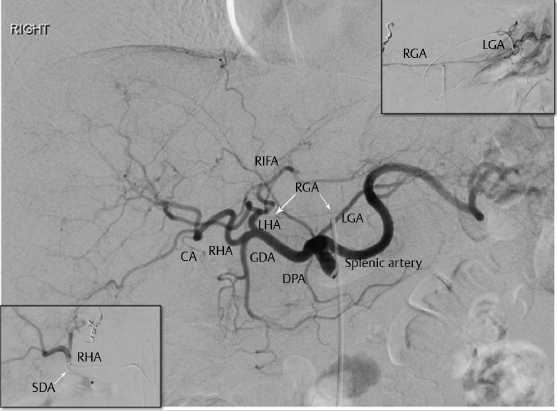
The planning angiogram concludes with the administration of MAA. Typically, a microcatheter is placed at the site(s) of intended treatment and a dose of 4 to 5 mCi is infused intra-arterially. MAA closely mimics the size, and hence, distribution of 90Y microspheres. This distribution is assessed with planar or single-photon emission computed tomography (SPECT) gamma cameras. A lung shunt fraction (LSF) is calculated (total lung counts/[total lung counts + total liver counts]) given inherent arterial venous connections in liver tumors, which shunt blood to the lungs. Determination of this shunt fraction allows estimation of the lung dose before radioembolization. In addition, scintigraphy, especially SPECT-computed tomography (CT), can detect other instances of nontarget deposition, including gut deposition. When nontarget deposition is identified, angiographic images should be carefully reviewed, with possible repeat angiography and MAA administration after correction of hepatoenteric communications.
Cone-beam CT (CBCT) produces CT-like images in an angiography suite and thus provides information about tissue perfusion in 3 dimensions. In a recent radioembolization study, CBCT was able to identify potentially hazardous instances of nontarget embolization and/or incomplete tumor perfusion that were not detected on DSA. 14 Delivery of 90Y microspheres without recognition of these vascular patterns can lead to ulcers or incomplete tumor coverage (▶ Fig. 23.2). Instead, CBCT in this study resulted in additional embolization or altered microcatheter treatment positions in one-third of patients, allowing for safe and complete tumor therapy.
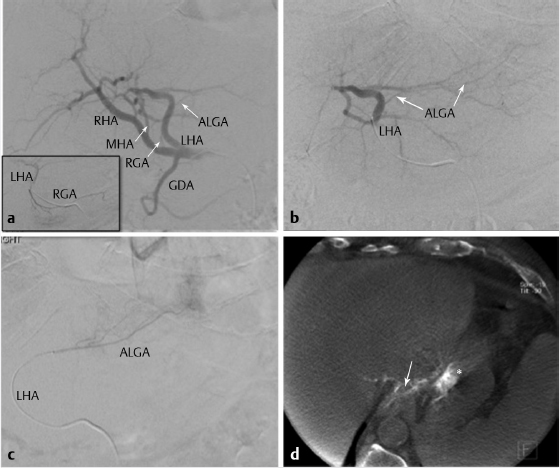
Patients who meet the criteria for radioembolization typically return on a separate day for treatment. Single-session radioembolization, in which MAA administration and 90Y microsphere infusion occur on the same day, is a novel concept that has demonstrated feasibility and safety in a pilot study. 15 Radioembolization is considered an outpatient procedure given the lack of macroscopic vessel occlusion and hence the occurrence of only mild postembolization syndrome.
In the radioembolization procedure, a microcatheter is placed into a treatment vessel and a prescribed dose of 90Y microspheres is infused per the manufacturer′s guideline. The recommended activity for TheraSphere radioembolization ranges from 80 to 150 Gy, with 120 Gy representing a dose target that balances safety and efficacy. Assuming a uniform distribution of 90Y microspheres, together with a known LSF and liver mass, a TheraSphere dose is calculated. There are several methods for calculating SIR-Spheres dosing, including the body surface area (BSA) and partition models. 3 Both methods assume a uniform distribution of 90Y microspheres and require a known LSF and liver mass and estimation of tumor burden. Activity calculators are available from both manufacturers.
23.5 Outcomes
23.5.1 Hepatocellular Carcinoma
HCC claims more than half a million lives annually. 16 Surveillance programs can lead to early diagnosis of HCC; however, only approximately half of the population at risk receives appropriate screening, resulting in frequent presentation of disease without curative options. 17 More specifically, only approximately 10% of patients with HCC receive therapy with curative intent 18 ; thus, most patients are treated with palliative intent. This treatment is often catheter based, namely transarterial chemoembolization (TACE) and 90Y radioembolization. 19 TACE has demonstrated improved survival among patients with HCC in randomized controlled trials and is considered the standard of care for appropriately selected patients with unresectable HCC according to the Barcelona Clinic Liver Cancer (BCLC) staging system, which is endorsed by the American Association for the Study of Liver Diseases. 20 , 21 , 22 , 23 Radioembolization is not recognized by the BCLC staging system; nevertheless, this therapy has received regulatory approval and acknowledgment by NCCN Clinical Practice Guidelines in Oncology (NCCN Guidelines®) for the treatment of HCC (Category 2A; ▶ Table 23.1). 24 , 25
Over the past decade, significant advancement in the science of radioembolization has occurred, along with technical standardization. Radioembolization is now a routine procedure yielding predictable results. 18 Numerous well-controlled studies have documented the safety and tumoricidal effect of 90Y microspheres. In a single-center prospective 5-year cohort study of 291 patients treated with glass radioembolization in 2010, the intricate interaction between tumor characteristics and the degree of hepatic dysfunction was highlighted. 26 As expected, survival was negatively affected by liver dysfunction (survival in patients with Child–Pugh class A, 17.2 months; Child–Pugh class B, 7.7 months). Moreover, time to progression (TTP) was also affected by Child–Pugh class and the presence of PVT (TTP in patients with Child–Pugh class A or B without PVT, 15.5 and 13 months, respectively; Child–Pugh class A or B with PVT, 5.6 and 5.9 months, respectively). These data compare favorably with the results of the Sorafenib Hepatocellular Carcinoma Assessment Randomized Protocol (SHARP), in which sorafenib therapy yielded a TTP of 5.5 months with an overall survival of 10.7 months in a principally Child–Pugh class A population. 27
Survival and prognostic factors were recently assessed in a retrospective multicenter European registry of 325 patients with unresectable HCC treated with resin radioembolization. 28 The median overall survival was 12.8 months, and survival was significantly influenced by BCLC stage (survival in patients with BCLC stage A, 24.4 months; BCLC stage B, 16.9 months; BCLC stage C, 10 months). The reproducible data achieved among the eight participating centers highlight the technical feasibility of the procedure. 18
A phase 2 study by Mazzaferro et al 29 assessed the efficacy and safety of radioembolization in patients with intermediate (BCLC-B) and advanced stage HCC (BCLC-C). The median TTP was 11 months, with a median overall survival of 15 months. The authors concluded that radioembolization is a competitive treatment option with a manageable toxicity profile. This study also further validates the reproducible outcomes of radioembolization in select patients, including those with vascular invasion.
No randomized trial has compared radioembolization with TACE for the treatment of HCC; more than 1,000 patients would need to enroll in such a trial to identify significant differences between the two treatments. 30 To date, several comparative effectiveness trials have evaluated radioembolization and TACE with regard to efficacy and their effects on downstaging and quality of life. A large comparative effectiveness study published in 2011 matched 122 patients treated with TACE with 123 patients treated with 90Y radioembolization. 30 Radioembolization resulted in a significantly longer TTP (13.3 months) versus TACE (8.4 months; p = 0.046). However, overall survival with the two techniques was not statistically different (TACE, 17.4 months; radioembolization, 20.5 months; p = 0.232), likely because of competing risks of death from HCC and cirrhosis.
TACE is a recognized method for downstaging from United Network for Organ Sharing (UNOS) T3 disease to T2 disease. 31 When this downstaging is successful, patients are considered for transplant and granted a Model for End-Stage Liver Disease (MELD) upgrade for HCC. In one study, the downstaging efficacy of TACE and radioembolization was evaluated in 86 patients with T3 disease. 32 Downstaging to UNOS T2 was achieved in 31% of TACE-treated patients and 58% of radioembolization-treated patients (p = 0.023). The median TTP was 12.8 months for TACE and 33.3 months for radioembolization (p = 0.005). These results demonstrate that radioembolization outperforms TACE for down-staging UNOS T3 disease to T2 disease, thus expanding the pool of patients considered for curative transplant.
Primary liver tumors are associated with a diminished quality of life. Disease presentation at an advanced stage means that most patients are treated with palliative intent. Because affected patients have a reduced life expectancy, quality of life becomes equally as important as overall survival. 33 In a prospective study, health-related quality of life was compared in patients treated with TACE or 90Y radioembolization. Despite the more advanced disease in patients treated with radioembolization, several quality of life metrics were significantly better, and overall quality of life reached near-significant improvement in patients treated with radioembolization versus those treated with TACE (p = 0.055).
As shown in these comparative effectiveness trials, radioembolization outperforms TACE in select patients with regard to TTP, downstaging to transplant, and maintenance of quality of life. Additionally, many investigators now consider 90Y radioembolization a preferred therapy for patients with advanced BCLC B and early BCLC C disease. 18
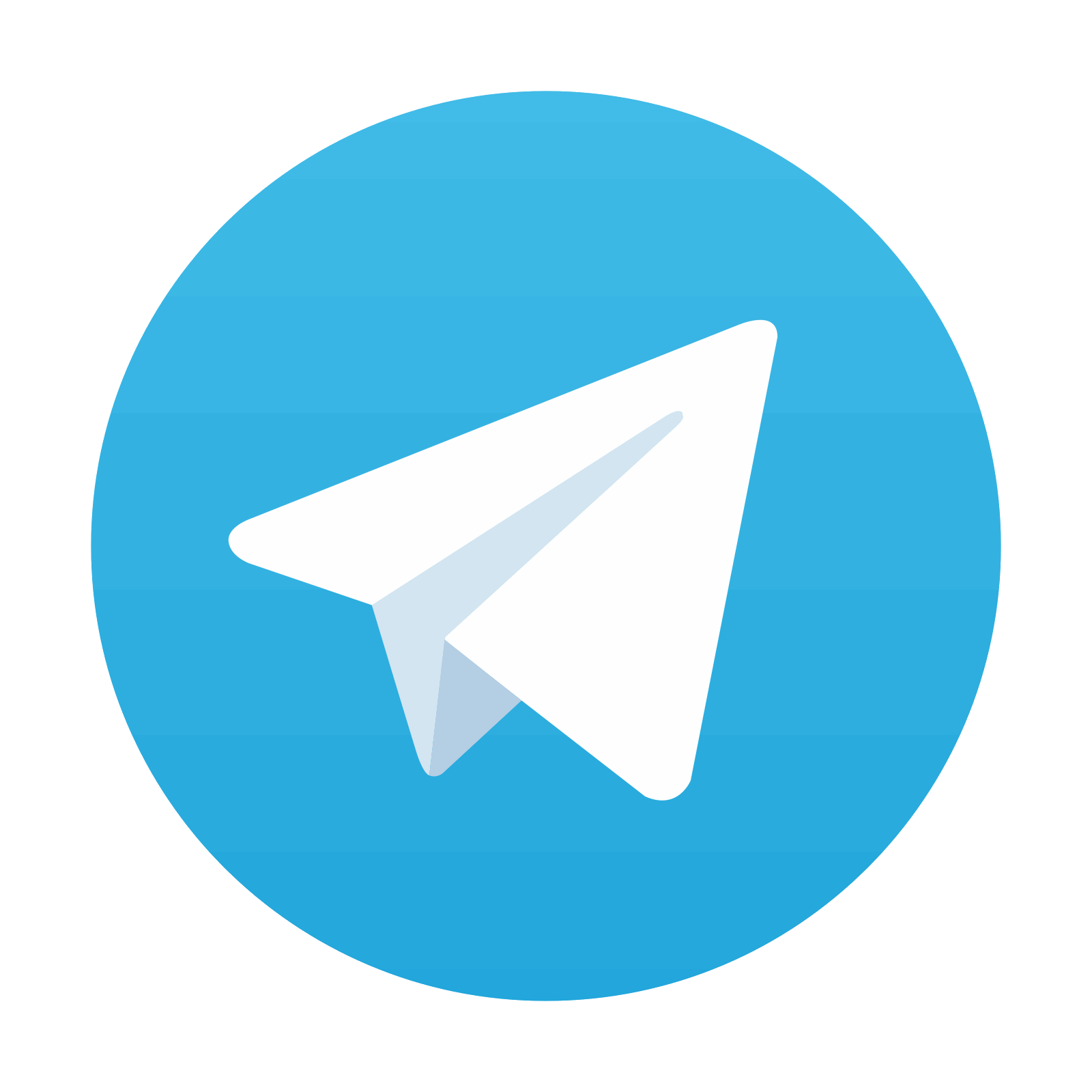
Stay updated, free articles. Join our Telegram channel

Full access? Get Clinical Tree
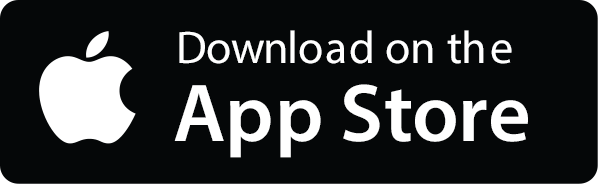
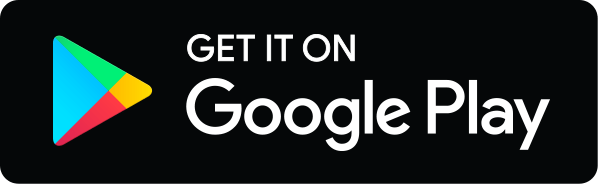
