3 Portal Hypertension
Robert O′Shea and Rachel Abou Mrad
3.1 Introduction
The term “portal hypertension” was first credited to Gilbert and Villaret in 1906 in research studies performed to investigate the etiology of ascites, gastrointestinal bleeding, and hepatofugal collateral development. 1 The normal hepatic venous pressure typically ranges from approximately 5 to 10 mm Hg2; portal hypertension represents an increase in pressure in the portal venous system to a level that exceeds the pressure in the inferior vena cava by more than 5 mm Hg. 3 However, the clinical manifestations of portal hypertension typically do not occur until this pressure difference is considerably higher, and thresholds at which patients typically develop bleeding or ascites have been established.
For many patients with cirrhosis, portal hypertension represents the clinical manifestation of liver disease—the actual signs and symptoms that patients with end-stage liver disease experience. Portal hypertension is the final common pathway of many forms of chronic liver disease, and its development is the turning point in the course of a chronic liver disease, a threshold event that signifies the transition from asymptomatic liver disease to decompensated cirrhosis. Portal hypertension can also occur in patients with acute severe liver disease, and the signs and symptoms in these cases may be indistinguishable from those in cases of chronic liver disease. Clinically, portal hypertension is recognized by the development of variceal bleeding, ascites, or hepatic encephalopathy. In early clinical studies, the prognosis of patients who presented with these forms of decompensation was invariably grim. 4 Before advances such as liver transplant were available, these studies reported 1-year survival rates of less than 40% when patients presented with either varices or ascites and 5-year survival rates of approximately 10%. 4
Liver disease was previously classified as either compensated or decompensated. Liver disease, however, is more intuitively thought of as a spectrum, with implications for patients with advanced liver disease who are asymptomatic—classified as chronic liver failure or acute-on-chronic liver failure in patients who have developed acute decompensation (ascites, hemorrhage, encephalopathy, and/or bacterial infections). 5 , 6 When patients are stratified by this method, it is still clear that those who have developed decompensation are at an increased mortality risk, but it is also clear that the symptomatic presentation of decompensation more typically represents the end stage of a patient′s evolution through the course of his or her disease (▶ Table 3.1). 7 Thus, patients who are diagnosed with cirrhosis while asymptomatic are at a very low risk of short-term mortality, which is manifestly not the case once patients become symptomatic (▶ Fig. 3.1). 7 It is clear from natural history studies that there is also a hierarchy of decompensation events that may culminate in mortality, and that as a patient experiences more of these events, the risks increase. Thus, patients with cirrhosis may be further subclassified more discretely into separate stages of disease. ▶ Fig. 3.2 shows an example of this progression risk based on a cohort of patients followed in a liver unit; the cases have been subclassified into five discrete stages, and transition rates have been calculated. 8
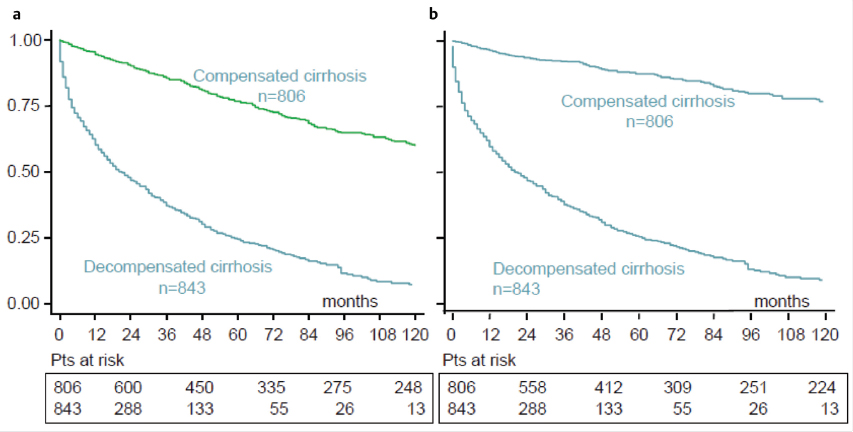
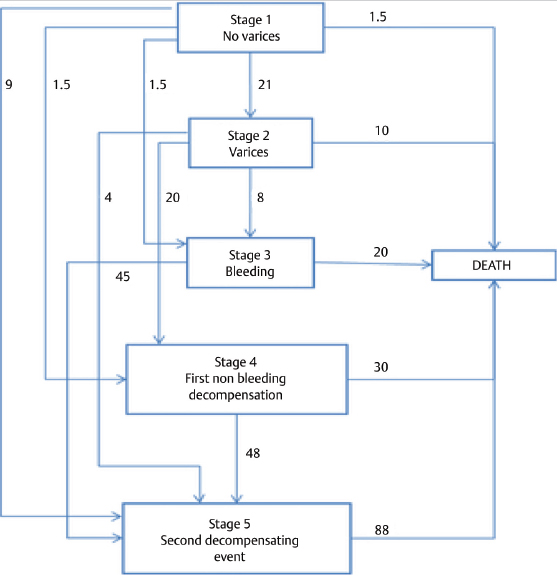
3.2 Anatomy of Portal Hypertension
Portal hypertension develops as a complication of resistance to portal blood flow (▶ Fig. 3.3). This resistance most often occurs within the liver (as in cases of cirrhosis) but can also be prehepatic (as in cases of portal vein thrombosis) or posthepatic, in which an obstruction to outflow of the liver exists (as in cases of Budd–Chiari syndrome) (▶ Table 3.2). 9 Portal hypertension can be further subdivided into presinusoidal, sinusoidal, and postsinusoidal pressure elevations.
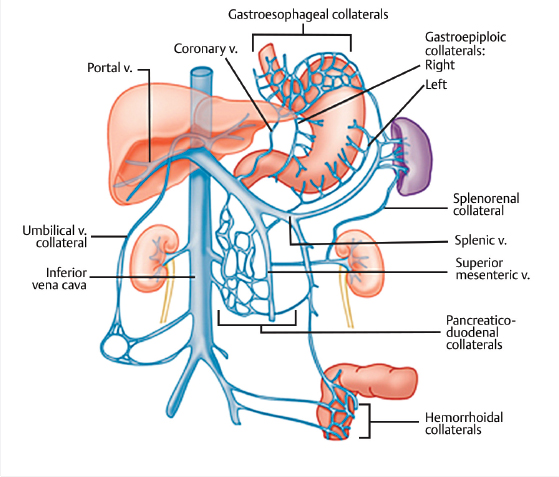
3.3 Noncirrhotic Portal Hypertension
Although considerably less common than portal hypertension in the setting of cirrhosis, noncirrhotic portal hypertension can occur in patients with certain vascular disorders. These cases can be classified by the site of blood flow obstruction (prehepatic, hepatic, or posthepatic). In patients with noncirrhotic portal hypertension, the hepatic venous pressure gradient (HVPG) is typically normal or minimally increased and liver function is usually preserved.
The incidence of noncirrhotic portal hypertension varies across the globe. For instance, this condition is very common among men in their second and third decades of life in India and is possibly responsible for almost one-third of all variceal bleeding episodes in this population, 10 whereas in Japan it is more common among women in their fourth decade of life.
A number of etiologies for noncirrhotic portal hypertension have been proposed, including chronic infections, exposure to certain medications or toxins, the presence of a hypercoagulable condition such as thrombophilia, and the presence of immunological or genetic disorders. 9 In Europe and the United States, other etiologies include congenital hepatic fibrosis and nodular regenerative hyperplasia, often seen in patients who have been treated with a number of chemotherapeutic agents or immunosuppressants. 11 Regardless of the inciting event, these injuries are thought to result in obstruction of the extrahepatic portal vein or microinfarction of the small- or medium-sized portal veins.
Clinicians must be able to distinguish between cirrhotic and noncirrhotic portal hypertension, as the approaches to treatment and prognosis are different for each entity. For example, patients with portal vein thrombosis (a presinusoidal cause of portal hypertension) are at high risk of developing bleeding but tend to have entirely preserved hepatic synthetic function; as a result, these patients tend to have a much better chance of surviving a variceal bleed than patients with decompensated alcoholic cirrhosis. 12 , 13 , 14
3.4 Normal Anatomy/Physiology
To understand the derangements of the portal circulation, one must first understand normal liver histology, particularly the spaces between the hepatocytes and the liver sinusoids, in which much of the molecular transport into the hepatocytes takes place and where the hepatic stellate cells are found. 15 These cells store fat-soluble vitamins but also have the ability to transform into activated cells that play a key role in chronic liver injury.
The liver receives approximately 25% of the total resting cardiac output and has a dual blood supply: the hepatic artery supplies approximately 25% of the total and the portal vein supplies the remainder. This blood is passed to the sinusoids, composed of one discontinuous layer of fenestrated endothelial cells, which are meant to function as low-pressure vascular channels, allowing blood to percolate across the hepatocytes before being delivered to the central veins. The total hepatic blood flow from both sources is typically 800 to 1,200 mL/min, or approximately 100 mL/min per 100 g of liver weight. 16 , 17 These two distinct sources of blood (oxygen-rich arterial blood from the hepatic artery and nutrient-rich blood from the portal vein) blend in the sinusoids, and the overall perfusion pressure is maintained by the hepatic arterial buffer response (▶ Fig. 3.4). 18
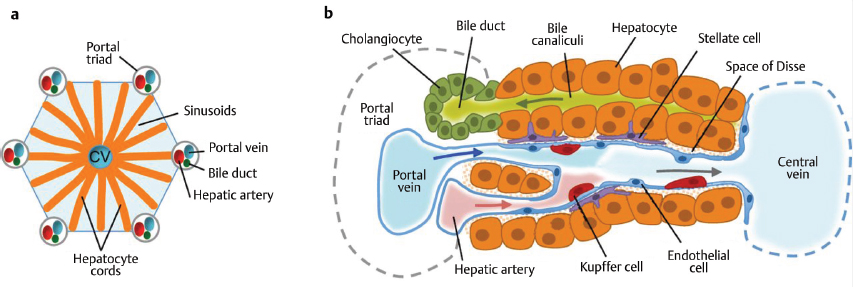
The space between the sinusoidal endothelium and hepatocytes is the space of Disse, where the resident macrophages of the liver (Kupffer cells) and the hepatic stellate cells (Ito cells) are located. Because of the presence of fenestrae between the sinusoidal endothelial cells, there is free flow of sinusoidal blood into the space of Disse. This arrangement has important consequences; in the healthy liver, plasma that collects in the space of Disse flows back toward the portal tracts, collecting in lymphatic vessels and forming a large fraction of the body′s lymph. However, in case of cirrhosis, the obstruction to flow results in overaccumulation of lymph, which presents as ascites. The stellate cells, which are typically quiescent cells that store vitamin A, may respond to injury by transforming into an activated form, proliferating and causing contraction and secretion of collagen. These stellate cells are therefore central to the development and maintenance of portal hypertension. 19
3.5 Definition and Measurement
The pressure in the portal vein is difficult to measure directly. A close estimate, however, can be obtained by measuring the wedged hepatic venous pressure, which estimates the sinusoidal pressure. This technique was described in 1951 by Myers and Taylor. 20
In this procedure, a balloon-tipped catheter is introduced via a transjugular approach and advanced into the hepatic veins, effectively occluding hepatic flow; this results in a continuous column of fluid between the catheter and the sinusoid, which can be measured. The gradient that exists between the wedged hepatic venous pressure and the free hepatic venous pressure, reflected in the intra-abdominal vena caval pressure, represents the resistance to flow across the liver. 21 , 22 Portal hypertension is diagnosed when the resistance to blood flow across the liver, defined as the difference between the wedged hepatic venous pressure (a measure of the flow into the liver from the portal vein) and the free hepatic pressure, is more than 5 mm Hg. 23 Clinically significant portal hypertension typically does not become manifest until the gradient is at least twice that. 24 , 25
This indirect measurement of portal pressure can be performed at the same time as a transjugular biopsy. In patients with suspected advanced liver disease, this approach is helpful not only in establishing a histologic diagnosis but also in assessing prognosis. Some have described this technique as a “splanchnic sphygmomanometer” that can be used to monitor the effect of therapy and assess the risk of bleeding. As this field has evolved, it has become increasingly clear that a threshold HVPG exists that may predict the likelihood of a patient developing variceal bleeding (initial or recurrent), ascites, or other end points that define decompensation. 26 The importance of the HVPG, which is a function of both the fixed and dynamic elements in cirrhosis, has been widely accepted, as this measurement conveys important prognostic information for patients with compensated cirrhosis, variceal bleeding, or decompensation. The HVPG has been recommended as a validated surrogate outcome measure for clinical trials in portal hypertension. 27 Moreover, direct measurement of the HVPG has been used to predict the risk of development of esophageal varices, 25 to predict outcomes in cases of acute variceal bleeding, 28 and to assess the prognosis of patients with recurrent hepatitis C after liver transplant. 29 Several authorities have recommended the routine use of HVPG measurement to help manage portal hypertension. However, HVPG measurement is invasive, expensive, and associated with a small but real incidence of complications.
3.6 Overview of Physiology/Pathophysiology of Cirrhosis/Portal Hypertension
At the macro level, portal hypertension can be understood in terms of the laws of physics governing flow, or Ohm′s law: pressure = vascular resistance × blood flow. The first event that occurs in the pathway to portal hypertension is an increase in resistance to flow, in part as a result of the disruption of the normal venous channels with the accumulation of progressive fibrosis. 30
The resistance to flow is proportional to the length of the blood vessel and the viscosity of the blood and inversely proportional to the radius to the fourth power. Because vessel length and blood viscosity are essentially fixed, the radius of the blood vessels becomes the most important driver of portal pressure, and changes in a blood vessel′s diameter—or by extension, cumulatively throughout the whole liver—are the biggest drivers of resistance since the radius term is raised to the fourth power. Thus, if a vessel′s size were reduced by half, there would be a 16-fold increase in resistance. Blood flow, however, is also markedly altered in cirrhosis.
As pressure increases across the portal venous system, an extensive network of portosystemic collaterals develops, diverting a large fraction of portal blood to the systemic circulation and bypassing the liver. Cirrhosis also tends to be accompanied by an increase in the presence of vasodilators. These vasodilators perpetuate a hyperkinetic systemic circulation, with reduced arterial pressure and peripheral resistance and increased cardiac output. This was first described in detail by Kowalski and Abelmann, 31 who reported that patients with cirrhosis typically presented with the signs and symptoms of “warm extremities, cutaneous vascular spiders, wide pulse pressure, and capillary pulsations in the nail beds.” This set of symptoms is sometimes referred to as “warm shock” and is now considered one of the hallmarks of cirrhosis.
A secondary event that occurs in all forms of portal hypertension is the emergence of an expanded plasma volume. This expansion of plasma volume is caused by renal sodium retention, which precedes the increase in cardiac output. The expanded blood volume represents a negative feedback loop and serves to further increase portal pressure.
Portal venous inflow is itself driven by the hyperdynamic circulation of cirrhosis and by increased plasma volume (▶ Fig. 3.5). 32 , 33
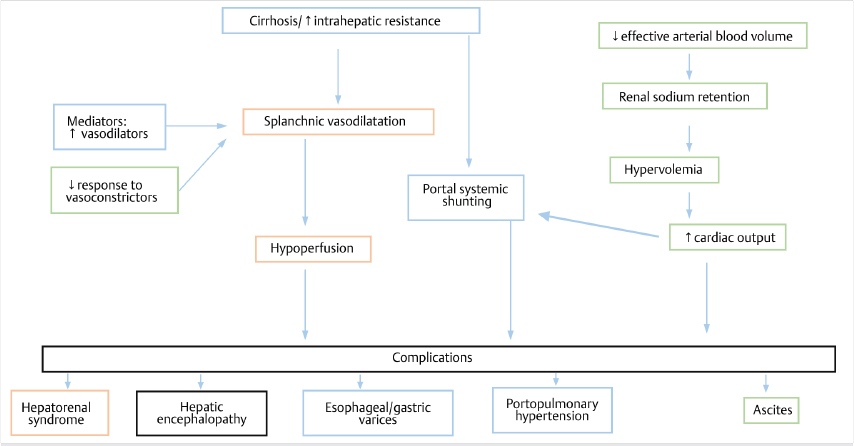
Historically, two theories regarding the evolution of portal hypertension were proposed, referred to as the “backward flow theory” and the “forward flow theory.” 34 The former theory speculated that the progressive increase in resistance across the portal venous stem would lead to a relatively hypodynamic circulation in the mesentery, which is seen in early cirrhosis. 35 However, in later stages, there is strong evidence of a hyperdynamic splanchnic circulation, with an increase in portal inflow driving the development of portal hypertension 33 , 36 (hence the “forward flow” theory).
Splanchnic vasodilatation itself can be explained by the combination of increased local vasodilator production and increased systemic vasodilators in the circulation, both of which lead in turn to a decreased response to vasoconstrictors. 37 Both the amount of portal venous inflow to the liver and the degree of resistance to flow can be modified. 38 The recognition that this was the case greatly influenced our current understanding of how patients with cirrhosis should be cared for clinically.
3.7 Hepatic Resistance
Although most forms of cirrhosis encompass aspects of both increased resistance to blood flow and increased splanchnic flow, increased intrahepatic resistance to flow is the critical element in most cases of portal hypertension. This may occur at a presinusoidal, sinusoidal, or postsinusoidal level. Cirrhosis is often thought of as a fixed obstruction, based on deposition of scar and development of regenerative nodules that distort normal patterns of blood flow. In support of this concept, histologic studies that have compared the extent of fibrosis with measurements of portal hypertension have demonstrated specific features, including small nodularity and septal thickness, to be independently predictive of clinically significant portal hypertension. 39
However, research has shown all of intrahepatic resistance is not fixed. Early seminal studies demonstrated that there was a dynamic component involved in the total resistance to flow across the liver, as the blood flow in an isolated rat liver in this research was responsive to vasodilators. 40 These vasodilators were thought to be acting on the myofibroblasts in perivenous and perisinusoidal locations. The extent of vasodilatation, moreover, is substantial and therefore has clinical implications, accounting for as much as 40% of the total resistance across the liver. 41
A large body of work has shown that the hepatic stellate cells play a major role in the physiologic control of portal flow. Because of the contractile properties and perisinusoidal location of these cells, they may regulate sinusoidal blood flow and resistance and thus may serve as a potentially modifiable target in portal hypertension. The microenvironment that regulates hepatic stellate cell contractility is a control point that determines the relative degree of relaxation or constriction. Several studies have shown that vascular mediators (e.g., angiotensin II) may act to stimulate proliferation of hepatic stellate cells and increase their ability to contract. 42 Moreover, stellate cells likely participate in the immunologic response to injury, both as targets and as sources of a number of autocrine and paracrine signals for other cells of the immune response. 19
Although multiple components of the normal hemodynamics of the liver are deranged in portal hypertension, it is likely that the hyperdynamic circulation of cirrhosis is driven primarily by abnormalities in vasodilatation, with relative imbalance in nitric oxide (NO) as the primary effector; this was first postulated in 1991 by Vallance and Moncada. 43 Multiple studies have since demonstrated the central role of NO in creating and sustaining the hyperdynamic circulation. This fact takes on particular significance in cirrhosis because of the possibility that NO may be a modifiable factor that could affect the outcome of portal hypertension. Extensive studies have investigated the control of NO synthesis and the potential counter-regulatory agents that promote constriction. 44 , 45 , 46 , 47 , 48
3.8 Mechanisms of Portal Hypertension
The resistance to flow and the amount of flow through the portal venous system can be envisioned as a two-compartment model, each of which has its own modifiers of circulatory hemodynamics: (1) the intrahepatic circulation, which reflects the longstanding injury related to chronic liver disease in terms of the accumulation of endothelial injury and scar tissue, and (2) the changes in splanchnic blood flow, which feeds the portal system. Each of these is modified by a number of mechanisms and agents that interact with these pathways (▶ Table 3.3, ▶ Table 3.4, ▶ Table 3.5). The cumulative effect of these pathways is what can be seen clinically.
Vasoconstrictors | Vasodilators |
Norepinephrine | Nitric oxide |
Thrombin | Carbon monoxide |
Angiotensin II | Heme oxygenase |
Endothelin | Hydrogen sulfide |
Prostanoids | |
Leukotrienes | |
Thromboxane A2 |
Vascular endothelial growth factor |
Nitric oxide |
Prostacyclin |
Carbon monoxide |
Endocannabinoids |
Endothelium-derived hyperpolarizing factor |
Hydrogen sulfate |
Glucagon |
Adrenomedullin |
Calcitonin gene-related peptide |
Cyclooxygenase-derived prostanoids (prostaglandin H2, thromboxane A2) |
Vasoactive intestinal peptide |
Tumor necrosis factor/bacterial translocation → endotoxin |
Substance P |
Estrogen |
Cholecystokinin |
Adenosine |
Bile acids |
Ammonia |
Histamine |
By far, the most important mediator of intrahepatic resistance is NO. NO is a gas that diffuses across the cell membranes and can therefore act in an autocrine or paracrine fashion. It is produced from the amino acid L-arginine by a family of NO synthases, including an endothelial NO synthase (eNOS) responsible for most of the physiologic production of NO, a neuronal form of synthase (nNOS), and an inducible form of NO synthase (iNOS). When produced, NO binds to soluble guanylate cyclase, resulting in increased levels of cyclic guanosine monophosphate, which in turn results in an increase in cytosolic Ca+ + and leads to relaxation of the vascular wall. 55
The regulation of NO is primarily controlled by regulation of the synthases; synthesis is activated by increases in intracellular calcium or by fluid shear stress and insulinlike growth factor.
Phosphorylation of eNOS by the serine/threonine protein kinase Akt activates the enzyme, leading to NO production. 56 NO in turn is inhibited by caveolin 1, which acts as a molecular chaperone and serves to regulate production and drive inactivation of NO. 57
eNOS is regulated by complex protein–protein interactions and posttranslational modification. 58 Among positive regulator proteins, Hsp9062 and serine/threonine kinases Akt/protein kinase B contribute to the activation of eNOS in the splanchnic arterial circulation. 59 Akt/protein kinase B directly phosphorylates eNOS at Ser1177 (human) or Ser1179 (bovine) and enhances its ability to generate NO. 56 , 60 , 61 , 62
Although NO synthesis does take place in cirrhotic livers (with normal levels of eNOS mRNA and protein), several mechanisms have been proposed to explain the differences in NO produced in cirrhotic liver: there is an increased expression of the proteins responsible for inactivation (caveolin), and the overall production of NO is not sufficient to overcome the increase in inflow and powerful mechanisms of vasoconstriction in these patients.
Another important vasodilator is carbon monoxide (CO), which is produced by heme oxygenases (HOs) in the process of breaking down heme to biliverdin and serves as an activator of guanylate cyclase. Two isoforms of HO have been identified: HO-1 and HO-2. HO-1 is inducible by multiple agents, whereas HO-2 is constitutively expressed. 63 , 64 HO levels tend to be higher in patients with liver disease (particularly in those with cirrhosis), leading to high CO levels, which correlate with increases in cardiac output and inversely with systemic vascular resistance. 65 Portal inflow is dictated by the degree of splanchnic vasodilation, the other vascular bed central to the clinical syndromes brought on by portal hypertension 38 ; this in turn is fueled by the hyperdynamic circulation and the increase in intravascular volume.
The same mediators that drive vascular resistance and vasodilatation are active in the splanchnic circulation, including NO, which is produced in relative excess. Studies have demonstrated that metabolites of NO are relatively increased in patients with cirrhosis, 66 which is thought to result from increases in shear stress (perhaps through upregulation of vascular endothelial growth factor [VEGF] expression) and from bacterial translocation and portosystemic shunting, all of which characterize cirrhosis. A number of studies have also suggested an association between the levels of NO (or its metabolites) and the degree of decompensation of liver disease, finding, for example, higher levels in patients with Child–Pugh class C cirrhosis than in patients with earlier stages of liver disease and in patients with peritonitis versus those with ascites but without peritonitis. Many of these same mediators are also involved in angiogenesis, 37 which plays a major role in the development of portal systemic shunts but also contributes to the progression of fibrosis within the liver. 67 , 68
Other major players in this system include the vasodilator prostacyclin (PGI2), which acts by stimulating adenylyl cyclase and increasing the generation of cyclic adenosine monophosphate. 69 Glucagon also plays a central role in splanchnic vasodilatation, acting to relax vascular smooth muscle and decrease the effect of endogenous vasoconstrictors. Glucagon levels in turn are relatively high because of overproduction and decreased clearance. Hydrogen sulfide (H2S) may also be important in vasodilatation. Preliminary studies have found that patients with portal hypertension had significantly lower serum H2S concentrations and that disease severity and the portal vein diameter (a proxy for the degree of portal hypertension) were inversely correlated with H2S concentration. 70
Endocannabinoids may also be important mediators of the hyperkinetic circulation, partly through activation of NO production. Endocannabinoid levels are increased in patients with cirrhosis, and studies using blockade of the cannabinoid receptors in vascular smooth muscle have demonstrated improvements in systemic blood pressure. 71 Unfortunately, these receptor blockers have not yet become available clinically.
Increased levels of tumor necrosis factor alpha (TNFα) have been found in multiple liver diseases and are thought to reflect the interaction between endotoxin from the gut and immune function (via the pathogen-associated molecular pattern molecules and damage-associated molecular patterns) by their effects on mononuclear cells. In alcoholic hepatitis, the presence of detectable TNF conveys a poor prognosis, but the overall levels are elevated in liver diseases associated with portal hypertension. 72 , 73 , 74 The mechanism by which TNFα induces NO is unclear but may be via increasing the gene expression and activity of one of the key cofactors of NO synthesis, tetrahydrobiopterin BH4 GTP-cyclohydrolase I, in endothelial cells, 75 , 76 as enhanced BH4 production directly increases eNOS-derived NO bioavailability.
Adrenomedullin is another vasodilator that may act via effects on NO production; it phosphorylates and activates Akt and increases cGMP production and has been found to circulate in increased levels in patients with cirrhosis. 77
Other agents that have been tested based on the pathophysiologic understanding of portal hypertension include angiotensin receptor antagonists, 78 which have been shown to improve the generation of NO and hepatic endothelial dysfunction, perhaps via an effect on developing hepatic fibrosis. 79 , 80
Balancing these changes in vasodilator levels is an accompanying increase in the relative amounts of circulating vasoconstrictors. A number of physiologic mediators have been implicated in this process, most notably endothelin 1 (ET-1) and ET-3, both of which are produced in the splanchnic circulation and found in increased concentration in cirrhotic livers, 81 as are their receptors. ET-1 has been shown to increase intrahepatic resistance, as have a number of other mediators, including norepinephrine, cysteinyl leukotrienes, thromboxane A2, and angiotensin, as well as the sympathetic nervous system. 82 , 83
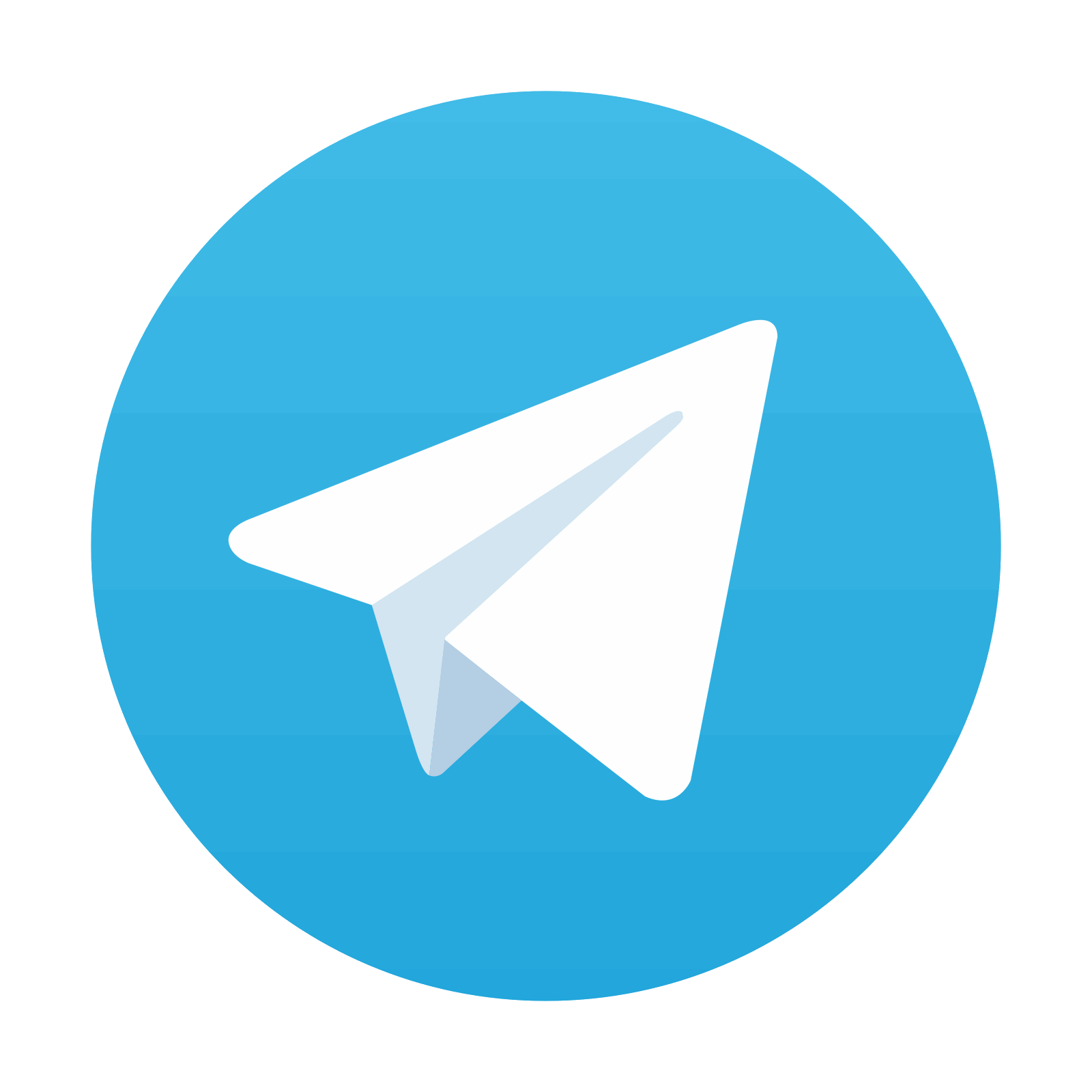
Stay updated, free articles. Join our Telegram channel

Full access? Get Clinical Tree
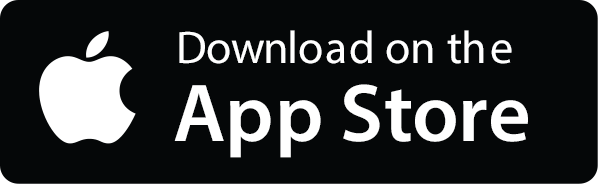
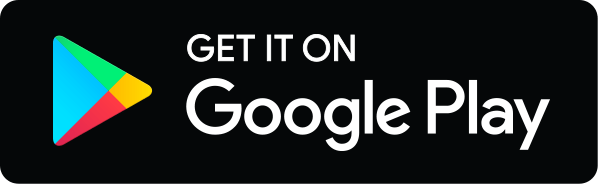