- Interaction of charged and uncharged particles with matter
- Interactions between photons and matter
- Quantities used in characterizing radiation interactions with tissue
- Linear energy transfer (LET) and relative biological effectiveness (RBE) of different types of radiation
- Direct and indirect interactions in tissue
Introduction
This chapter is concerned with the deposition of energetic radiation into living tissues. Before discussing the biological effects of radiation, we will consider ionizing radiation interactions in tissue. This includes the processes through which radiation interacts with tissue and how these interactions affect biological systems. Finally, damage produced by different types of radiation will be discussed.
Charged Particle Interactions
Charged particles interact with the tissue through electrostatic interactions. These interactions result in slowing of the charged particle and a loss of kinetic energy (KE). The lost energy is transferred to the surrounding tissue by excitation and ionization. Excitation occurs when a particle interacts with and excites an atom. The excited atom may then de-excite and emit radiation. Ionization occurs when a charged particle removes an orbital electron from an atom. The result of ionization is a free electron and a positively charged ion. The negative electron and positive ionized atom are called an ion pair. For air and tissue, the average energy per ion pair is approximately 34 eV. Roughly, a third of this energy goes into removing the electron from the atom, and the rest goes into excitation and KE. Ionization in water and organic material is very important in radiation biology due to the formation of free radicals, which is an important cause of radiation damage.
The electrostatic interaction of a charged particle is proportional to the charge of the particle. Particles with multiple charges such as alpha particles (+2 charge) have stronger electrostatic interactions with the medium than singly charged particles such as electrons (−1 charge). As a result, alpha particles lose energy more rapidly in matter than do electrons.
Another mechanism of energy loss by charged particles is radiative emission. In this case, a charged particle interacts with the electromagnetic (EM) field of an atom and the resulting electrostatic force changes the direction of the charged particle. This abrupt change in direction results in the emission of EM energy. The emitted photons are referred to as “bremsstrahlung,” a German word for “braking radiation.” Bremsstrahlung emission is only of importance for light charged particles, namely electrons and positrons, which are readily deflected from a straight path.
EM Radiation Interactions
X-rays and gamma rays are high-energy EM radiations with zero mass and zero electrical charge. The basic unit or “packet” of EM radiation is the photon. As discussed in the previous chapter, high-energy photons have higher frequencies and shorter wavelengths, and they penetrate more deeply in tissue than low-energy photons.
Ionizing photons have three main interaction mechanisms—(1) the photoelectric effect, (2) Compton scattering, and (3) pair production—each of which is prevalent over a different energy range. The photoelectric interaction and Compton scattering are dominant for the lower energies used in diagnostic imaging. The total likelihood of a photon interacting with a medium decreases with increasing photon energy.
Photoelectric Effect
The photoelectric effect is the dominant process at low photon energies (tens of electron volts up to 100 keV). In a photoelectric interaction, the incident photon is completely absorbed by the atom, and the photon energy is transferred to an orbital electron. For the photoelectric interaction to occur, the orbital electron must have a binding energy (BE) that is less than the energy of the photon. Figure 4.1 illustrates the photoelectric effect. The atom is ionized when this electron, which gains enough energy from the photon to overcome its BE, is ejected from the atom. The ejected electron is called a photoelectron. Thus, the photoelectron’s KE differs from the photon energy by its BE:
(4.1)
Figure 4.1 Photoelectric effect. The net result of the photoelectric effect is the complete absorption of the incident X-ray and the ejection of a photoelectron.
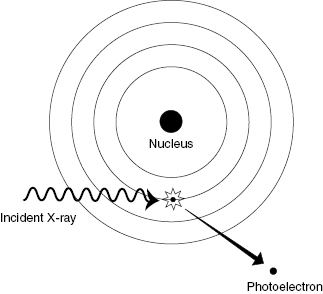
At energies encountered in diagnostic imaging, the photoelectron travels less than a few millimeters in tissue. If an inner shell electron is ejected, then an outer shell electron will fill the inner shell vacancy. The inner shell has a stronger BE, and the excess energy is usually released as a characteristic X-ray. Characteristic X-rays from tissue elements (i.e., carbon, nitrogen, and oxygen) have very low energies and do not exit the patient because of their low energies.
Compton Scattering
The Compton interaction typically involves an interaction between an incident photon and an outer shell electron, resulting in a scattered photon and a scattered electron. The incident photon loses a portion of its energy in the collision and changes direction (which causes blurring of diagnostic X-ray images). Outer shells of an atom are lightly bound, so removal of the electron from the atom does not require much energy. The incident photon energy is shared between the scattered photon and the scattered electron. Figure 4.2 shows the essentials of a Compton interaction. Compton scattering is the dominant process at intermediate photon energies (100 keV to 10 MeV).
Figure 4.2 Compton scattering. The energy of the incident photon is shared between the scattered photon and the Compton (recoil) electron.
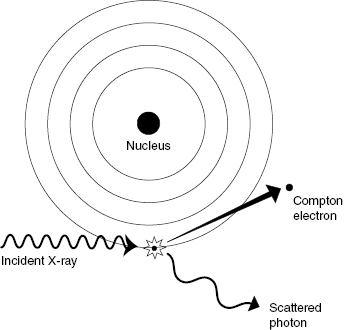
Unlike the photoelectric effect, Compton scattering results in a recoil electron and a scattered photon, both of which are capable of producing further ionization. Recall that the net result of the photoelectric interaction is complete absorption of the photon and emission of an energetic electron. The major contribution of the Compton interaction in medical imaging is an increase in patient dose and loss of contrast in the image.
Pair Production
Pair production occurs when the incident photon interacts with a nearby nucleus, creating an electron–positron pair. A positron is the antiparticle of the electron. The positron has the same mass as an electron but opposite charge. From Einstein’s equation for mass–energy equivalence (E = mc2), the mass of an electron or positron is equivalent to an energy of 0.511 MeV. For pair production to occur, the incident photon must therefore have an energy of at least 1.022 MeV, because the mass of the electron–positron pair is formed from the incident photon energy. Any energy above the threshold is shared as KE between the electron–positron pair. Figure 4.3 illustrates the pair production reaction.
Figure 4.3 Pair production. An incident photon with energy greater than 1.022 MeV can generate a positron/electron pair. The positron and electron have rest masses of 0.511 MeV and share the excess kinetic energy.
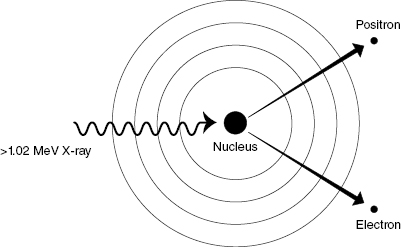
The photoelectric effect and Compton scattering are the most important modes of interaction for photons in the diagnostic energy range. As summarized in Fig. 4.4, below approximately 100 keV, the photoelectric effect is the most frequent mode of interaction between photons and tissue. Compton scattering is the dominant mode of interaction in the 100 keV to 10 MeV range. Above approximately 10 MeV, pair production becomes the most common mode of interaction for photons in tissue. It is important to keep in mind that in all three processes, energy is transferred from a photon to electrons.
Figure 4.4 Probability of interaction versus photon energy for (1) the photoelectric effect, (2) Compton scattering, and (3) pair production.
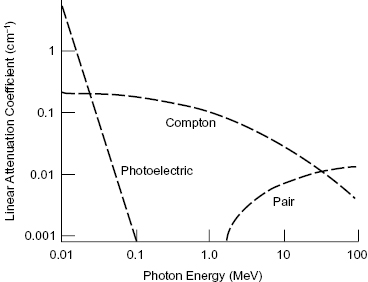
Neutrons
Neutrons are indirectly ionizing particles that cannot interact by the Coulomb force due to their neutral charge. Instead, neutrons lose energy by collisions that transfer KE to the tissue.
Neutron interactions are complex and depend on the neutron KE. A discussion of all types of neutron interactions is beyond the scope of this book. The majority of the energy lost by neutrons in a hydrogen-containing medium (such as living tissue) is through collisions with hydrogen nuclei producing recoil protons. These energetic protons, like energetic electrons, then go on to damage biological systems via ionization of atoms/molecules along the particle path. Figure 4.5 shows how an uncharged neutron can transfer energy through a collision with a charged nucleus producing subsequent ionizations.
Figure 4.5 Neutrons experience scattering, particularly in hydrogenous materials. The target nucleus is typically a single proton (hydrogen nucleus), which gains kinetic energy from the incident neutron.
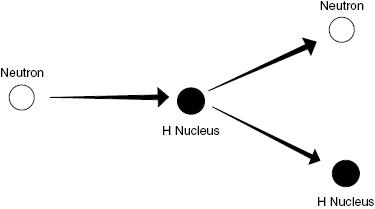
Specific Ionization
For charged particles, we can define the specific ionization as the number of ion pairs formed per unit path length. Alpha particles can produce several thousand ion pairs per millimeter. For beta particles (electrons), the specific ionization is 50–100 ion pairs per millimeter. Energetic neutral particles, such as neutrons and photons, can liberate charged particles (e.g., protons, electrons) for which the specific ionization can then be defined. Specific ionization differs for different materials and tissues.
Linear Energy Transfer (LET)
LET is the energy transferred by radiation per unit path length in soft tissue. LET is the product of the average energy transferred per ion pair and the specific ionization (number of ion pairs per unit length). LET is usually expressed in units of keV/μm. The specific ionization (ion pairs per path length) is greatest for heavy charged particles such as the alphas. Alphas leave a densely ionized particle track (high specific ionization) and deposit a relatively large amount of energy per unit length. Alphas and neutrons (which produce recoil protons) are considered high-LET radiations. On the other hand, betas and photons (which liberate electrons) leave a sparsely ionized particle track. The amount of energy deposited per unit path length is relatively low, so beta particles and X-rays/gamma rays are considered low-LET radiations.
Although photons do not carry charge, they set electrons in motion and thus produce ionization. Once a photon transfers energy to an electron, the LET is that of electrons. Likewise, neutrons do not carry charge, but they set protons in motion. The LET for neutrons is therefore similar to that of protons.
The LET depends on the type of radiation and its energy. Lower-energy particles interact more strongly and have a higher LET, compared with higher-energy particles of the same type.
Path and Range of Charged Particles
Charged particles undergo electrostatic interactions, attractive or repulsive, that may deflect the particle from a straight path. Charged particles with low mass such as the electron are easily deflected. The path of an electron is therefore a series of random scatters that appears as a contorted path as shown in Fig. 4.6. Heavy charged particles, such as alphas, which are over 7000 times more massive than an electron and have twice the charge, are not as easily deflected. These particles tend to take relatively straight paths as they lose energy in matter. The path length of a particle is a measure of the actual distance that the particle has traveled, not the distance “as the crow flies.”
Figure 4.6 Alpha particles have high specific ionization and a relatively straight path as they interact with matter. Electrons have a lower specific ionization and more contorted path.
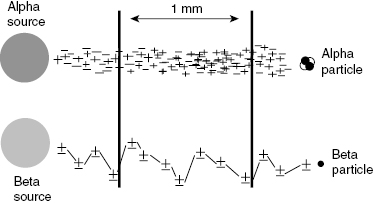
As particles slow, the rate of energy transfer increases. Heavy ions therefore exhaust most of their KE at the end of the particle track. As shown in Fig. 4.7
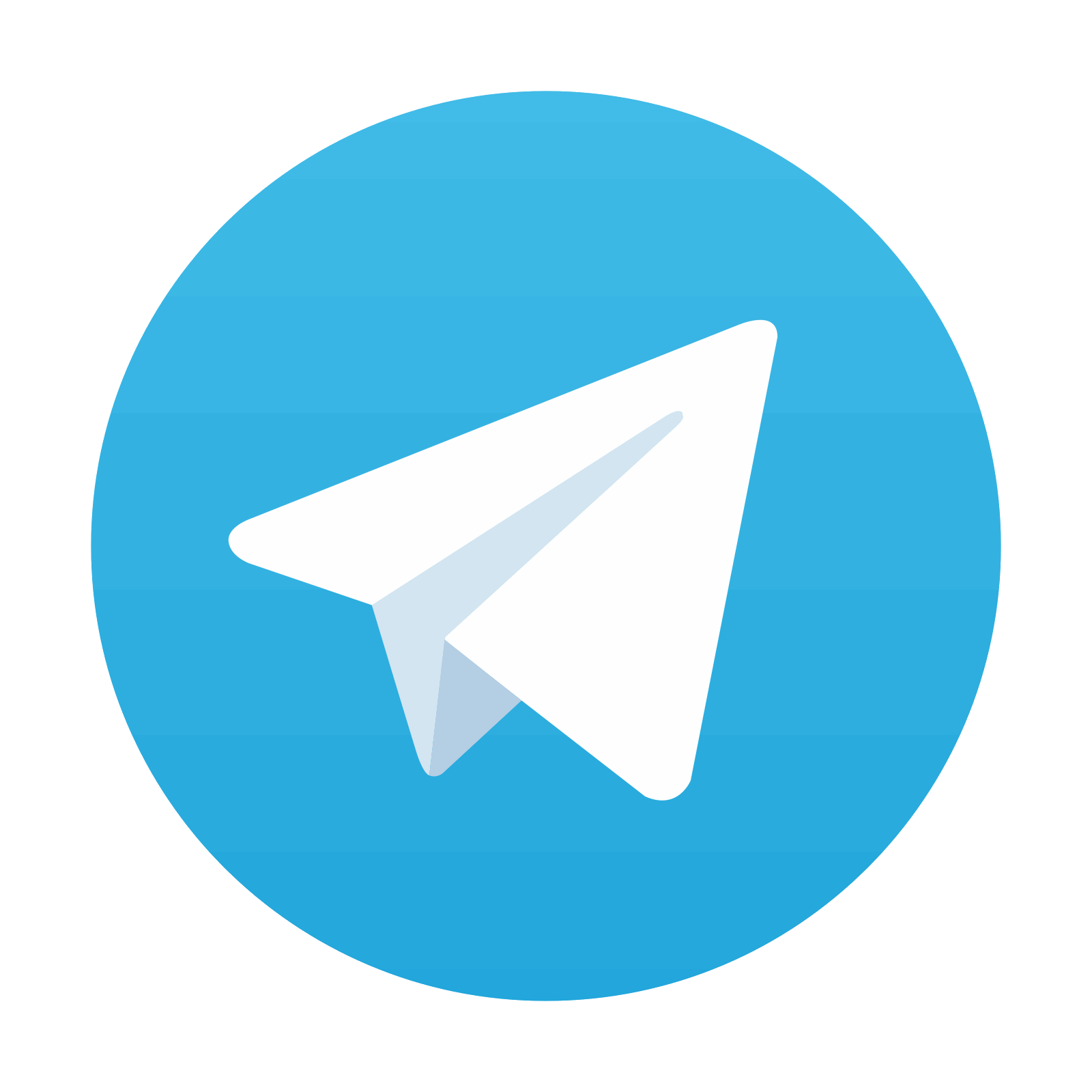
Stay updated, free articles. Join our Telegram channel

Full access? Get Clinical Tree
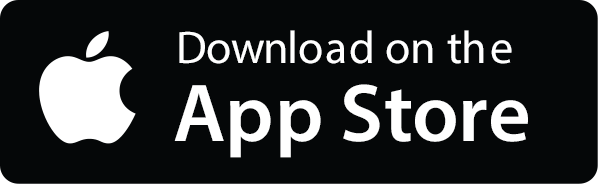
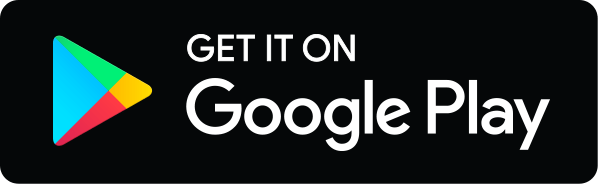