This article discusses common injury mechanisms and the subsequent constellation of magnetic resonance (MR) imaging findings in the knee following trauma in the context of instability, as distinguished by the degree of knee flexion and tibial rotation at the time of initial injury, in addition to the direction and magnitude of the responsible force vectors. Using 3-dimensional imaging, common injury mechanisms are illustrated and correlated with MR imaging findings of the resulting osteochondral, ligamentous, meniscal, and musculotendinous lesions. The most common classification and grading systems for these individual lesions and their subsequent treatment implications are discussed.
Key points
- •
An understanding of the functional anatomy of the knee aids in recognizing common injury mechanisms of knee trauma at magnetic resonance (MR) imaging and resulting clinical instability.
- •
The presence of specific osseous and soft-tissue injuries can help elucidate the mechanism of injury and provide a targeted approach to MR imaging evaluation of the knee following trauma.
- •
Injuries of the knee can be categorized as occurring in hyperextension, in physiologic extension, and in flexion with varying degrees of angulation and rotation, and result in characteristic osteochondral, ligamentous, meniscal, and musculotendinous lesions.
- •
Recognition by the radiologist of these key injury patterns and clinical instability may aid in the detection of occult and subtle injuries that may require early surgical treatment to prevent subsequent treatment failure.
Introduction
Magnetic resonance (MR) imaging of knee injuries enables identification of soft-tissue and radiographically occult bone injuries, and facilitates analysis and reporting of injury constellations in the context of functional knee instability. Previous works have described the stabilizing structures of the knee, common destabilizing injury patterns, and the MR imaging appearance of common knee injuries. The purpose of this review is to discuss the normal functional anatomy of key soft-tissue stabilizers of the knee, summarize the currently known etiology and types of posttraumatic knee instability, present the most common resultant MR imaging injury patterns, and synthesize a unified model for use as a targeted reporting checklist during MR image interpretation. By illustrating the biomechanical mechanism and clinical relevance of potentially destabilizing injuries, an improved understanding will allow the radiologist to better identify significant, subtle, and potentially occult injuries, and thus provide more clinically relevant interpretations.
Introduction
Magnetic resonance (MR) imaging of knee injuries enables identification of soft-tissue and radiographically occult bone injuries, and facilitates analysis and reporting of injury constellations in the context of functional knee instability. Previous works have described the stabilizing structures of the knee, common destabilizing injury patterns, and the MR imaging appearance of common knee injuries. The purpose of this review is to discuss the normal functional anatomy of key soft-tissue stabilizers of the knee, summarize the currently known etiology and types of posttraumatic knee instability, present the most common resultant MR imaging injury patterns, and synthesize a unified model for use as a targeted reporting checklist during MR image interpretation. By illustrating the biomechanical mechanism and clinical relevance of potentially destabilizing injuries, an improved understanding will allow the radiologist to better identify significant, subtle, and potentially occult injuries, and thus provide more clinically relevant interpretations.
Normal functional anatomy
Normal knee motion involves flexion and extension in the sagittal plane, translation in 3 planes (anterior-posterior, medial-lateral, and proximal-distal), and both internal and external rotation of the femur relative to the tibia. In full extension, the femur is slightly internally rotated, with the lateral femoral condylar rotation locking the knee in place. As a result there is minimal abduction, adduction, or rotational laxity to the fully extended knee. With flexion, the femur rotates externally and unlocks the knee. Moderate passive rotation and mild laxity in lateral motion are normally demonstrated with the knee in 90° of flexion. Destabilizing knee injuries commonly produce clinically detectable increases in rotational or lateral knee laxity than would otherwise be expected for a given state of knee flexion.
Knee instability has been classified according to the Committee on Research and Education of the American Orthopedic Society for Sports Medicine as: (1) 1-plane or straight, (2) rotatory, or (3) combined instability. The osseous structures of the knee provide minimal inherent stability. Rather, the soft-tissue structures that span the joint provide the dynamic stabilizing action. The cruciate and collateral ligaments, the joint capsule, and the musculotendinous units play a crucial role in providing knee stability ( Fig. 1 ). The cruciate ligaments are intra-articular but extrasynovial structures that primarily resist anteroposterior displacement of the tibia relative to the femur to stabilize the knee joint. The vertical axis of flexion and rotation of the knee, termed the central pivot, normally lies close to the attachment sites of the posterior cruciate ligament (PCL). Destabilizing knee injuries result in the shift of this central pivot away from the injured structures ( Fig. 2 ).
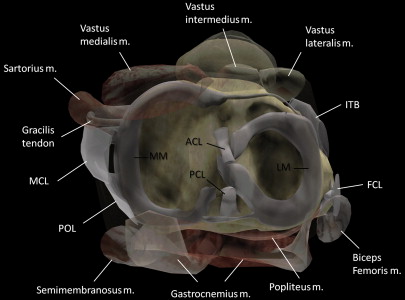
The cruciate and collateral ligaments serve complementary roles in stabilizing the knee during rotation. During tibial internal rotation the cruciate ligaments coil and effectively shorten about the central pivot. In particular, the PCL becomes increasingly taut, thus providing proportionally greater stabilization than the anterior cruciate ligament (ACL), with a concomitant increase with knee flexion. However, the collateral ligaments straighten during internal rotation, become relatively lax, and provide less stability. Conversely, during tibial external rotation the collateral ligaments shorten, assume more static tension, and provide increased stability. The cruciate ligaments uncoil and effectively lengthen during external rotation, thus diminishing their stabilizing function. With neutral rotation, the cruciate and collateral ligaments are not under any specific tension and provide less overall resistance to motion ( Fig. 3 ).
The ACL comprises 2 distinct bundles. The anteromedial bundle primarily resists anterior tibial translation with the knee in flexion, and the posterolateral bundle resists anterior tibial translation and rotation with the knee in extension. The ACL is the primary constraint against anterior tibial translation; straight anterior instability implies complete disruption of the ACL, often accompanied by medial and lateral capsular ligament injury (see later discussion). The presence of a concomitant complete PCL disruption further exacerbates this instability.
The PCL is the primary stabilizer against posterior translation of the tibia, although the posterior oblique ligament (POL), the fibular collateral ligament (FCL), the posterolateral corner structures, and the posterior joint capsule all provide key secondary roles. The PCL has been described as the primary stabilizing ligament of the knee owing in part to its function in determining straight versus rotatory instability. True rotatory instability requires that the cruciate ligaments are not completely compromised.
Lateral stabilization of the knee is provided by several structures with the FCL, or lateral collateral ligament, being the primary constraint to lateral joint opening. The anterolateral corner is stabilized primarily by the lateral capsular ligament, a focal thickening of the middle one-third of the lateral joint capsule, and to a lesser extent by the iliotibial band (ITB). The ITB represents the distal extension of the fascia lata of the lateral thigh, and helps reinforce the lateral capsular ligament. The ACL and FCL play critical supplementary roles in anterolateral stability. The posterolateral corner is primarily stabilized by the arcuate complex, which consists of the FCL, the arcuate ligament, the posterior one-third of the lateral joint capsule, the popliteofibular ligament, and fabellofibular ligament. Additional posterolateral stabilizing structures include the biceps femoris tendon, popliteus muscle and popliteomeniscal ligament, and the lateral head of the gastrocnemius. These posterolateral corner structures, along with the PCL, help to maintain posterolateral stability and to counter tibial external rotation forces.
Primary constraints against medial joint opening include the superficial medial collateral ligament (MCL) and the POL, a ligament in continuity with the posteromedial joint capsule. The ACL provides supplementary support while the medial head of the gastrocnemius aids in dynamic stabilization. The MCL and the POL also provide supplementary and reciprocal support to counter internal and external rotation, depending on the degree of knee flexion. The deep fibers of the medial collateral ligament, the meniscotibial and meniscofemoral ligaments, merge with an area of focal thickening of the middle one-third of the joint capsule termed the medial capsular ligament. These fibers provide a small but significant contribution to constraint against medial joint opening and external rotation. However, the anterior one-third of the medial joint capsule contributes little to medial knee stability and is infrequently torn. The semitendinosus and semimembranosus muscles play complementary but minor roles in medial stabilization; the semitendinosus counteracts valgus forces while the semimembranosus acts as a dynamic stabilizer of the posteromedial corner via its attachment with the POL and posterior capsule.
The patella is a sesamoid bone that articulates with the medial and lateral femoral condyles at the intercondylar groove, and serves to magnify the moment arm of knee extension via anterior displacement of the quadriceps myotendinous unit. The quadriceps and patellar tendons merge with the superior and inferior poles of the patella, respectively, and contribute to vertical stability. The normal 15° to 20° angle of the quadriceps insertion relative to the patellar tendon insertion produces a resting valgus orientation of the extensor mechanism during full extension. This situation results in a lateral resting position of the patella in the intercondylar groove on extension, and medial movement of the patella to the intercondylar midline with increasing flexion ( Fig. 4 ). Medialization of the patella on flexion increases the surface area of articular contact, thus increasing patellar stability. The patella is stabilized medially by the medial patellofemoral ligament (MPFL) and medial patellar retinaculum, with dynamic stabilization provided by the oblique head of the vastus medialis muscle. The lateral retinaculum merges with both the vastus lateralis muscle and ITB, providing lateral patellar stability.
The angle of the quadriceps tendon relative to the patellar tendon insertion also secondarily determines the magnitude of anterior tibial shear force produced during quadriceps contraction. Higher quadriceps-patella tendon angles in extension produce more anterior shear force and result in greater anterior tibial translation than would otherwise occur with knee flexion. This anterior shear force is countered primarily by the ACL, followed by the PCL and medial and lateral capsular ligaments, while the hamstring muscles provide dynamic stabilization.
An understanding of the functional anatomy and normal stabilizing role of the soft-tissue structures of the knee can provide an informed and more directed search for specific destabilizing injuries, in the context of the known clinical knee instability according to specific type and subtypes. In particular, the presence of straight instability should specifically raise suspicion for cruciate ligament injury, whereas rotatory instability is suggestive of at least partial PCL integrity ( Table 1 ).
Instability Type | Subtype | Primary Stabilizers | Secondary Stabilizers |
---|---|---|---|
One-plane (straight) instability | Anterior | ACL | Medial and lateral capsular ligaments |
Posterior | PCL | Arcuate complex, POL, posterior capsule | |
Medial a | MCL, POL, medial capsular ligament | PCL a | |
Lateral a | FCL and arcuate complex, lateral capsular ligament | PCL a , biceps femoris tendon, ITB, ACL | |
Rotatory instability | Anterolateral | Lateral capsular ligament, FCL and arcuate complex | ACL, ITB |
Anteromedial | MCL, POL, medial capsular ligament | ACL | |
Posterolateral | FCL and arcuate complex, lateral capsular ligament | PCL (incomplete injury), popliteus tendon | |
Posteromedial | MCL, POL, medial capsular ligament b | PCL b | |
Combined rotatory instability | Combinations of the above primary types of rotatory instability | Details are beyond the scope of this review |
a Straight medial and lateral clinical instability require high-grade injury to the PCL, or will alternatively present with rotatory instability.
b Posteromedial rotatory instability is controversial, and unlikely in the presence of a high-grade PCL injury.
Imaging findings of acute injury
Traumatic knee injuries often demonstrate characteristic osseous and soft-tissue injury patterns that can reveal the underlying injury mechanism, and the subsequent risk and type of potential instability. Keeping in mind the normal functional anatomy just discussed, the approach to evaluation using MR imaging should begin with an assessment for osseous and osteochondral injuries, as these often best illustrate the primary force vector of the trauma in addition to any secondary contrecoup or contralateral distraction or avulsion components. With this inferred force vector, the stabilizing ligaments at greatest risk for injury should then be evaluated, as ligament injury patterns can suggest the type of resulting instability. Attention should next be directed to the relevant myotendinous units, as these provide secondary stabilization; underlying disruption can suggest the magnitude or severity of the overall injury and subsequent instability. Finally, the menisci should be scrutinized in the setting of specific injury patterns, as unrecognized and inappropriately treated injury can result in chronic disability. The following sections provide general guidance on the assessment and grading of these injuries, although a thorough discussion is beyond the scope of this review.
Osseous and Osteochondral Lesions
Findings of acute knee trauma commonly include bone contusions, isolated or pure chondral injuries, subchondral or osteochondral fractures, or cortical extra-articular fractures. Multiple classification systems of acute traumatic osteochondral lesions have been proposed, although commonalities exist between these systems. Bone contusions likely represent microtrabecular fractures with superimposed marrow edema and hemorrhage, without overt cortical bone disruption or an identifiable cortical fracture line. Contusions can appear as ill-defined regions of hypointensity on T1-weighted and proton density (PD)-weighted sequences, with corresponding hyperintensity on short-tau inversion recovery (STIR) or T2-weighted sequences with fat suppression (FS) that often exceeds the area of T1 abnormality. Subchondral fractures are distinguished from contusions by the presence of a hypointense fracture line most often immediately beneath the subchondral lamella on T1-weighted imaging sequences, lying within a larger region of bone edema ( Fig. 5 ). These injuries are termed osteochondral fractures if they extend through the articular cartilage into the underlying cortex. Isolated or pure chondral injuries can also be seen, ranging in appearance and severity from signal heterogeneity, fissuring, chondral flap or delamination, chondral depression into an underlying impaction fracture, or osteochondral indentation or flake fractures ( Fig. 6 ). Multiple classification systems of osteochondral injury and morphologies on MR imaging have been described, although no single system has gained universal acceptance. In general, injuries without chondral disruption will more likely undergo conservative nonsurgical treatment, whereas surgical fixation will often be used for chondral and osteochondral fractures, often after a trial of conservative therapy for smaller lesions.
Contusion edema patterns occur commonly as a result of impaction or distraction, whereby the incident force vector causes direct impact of 2 or more bones on one side of the knee, with accompanying distraction on the contralateral side. For example, a severe valgus-inducing force may generate lateral knee compression and medial knee distraction. Lateral bony impaction results in bony contusion and/or osteochondral injury, whereas medial knee distraction results in tension on the collateral ligaments and subsequent sprain, tear, or avulsion types of injury ( Fig. 7 ). Avulsion fractures are commonly seen on the side of distraction, but cause relatively less bone marrow edema in comparison with impaction injuries ( Fig. 8 ). Avulsion fractures can be subtle on MR imaging, with only mild donor-site bone marrow edema and an often indistinct, small avulsed cortical fragment. Distraction without true avulsion fracture can also manifest as focal or linear edema at the associated ligamentous or tendinous attachment site, similar to a focal contusion.
MR imaging findings of contusions can develop in as little as 1 hour following trauma and can routinely persist for more than 40 weeks. Although the overall prognosis of contusion without initial overlying cartilage injury is generally good, late development of chronic overlying chondral or subchondral defects can occur if not appropriately treated.
Ligamentous Lesions
Grading of collateral ligament injuries, as grade I, II, or III, is based on clinical assessment rather than by strict imaging criteria alone, by assessing the degree of ligament injury and presence of clinical instability through provocative maneuvers during physical examination. Significant clinical MCL instability qualifies as a grade III injury, and can be further subclassified by the severity of medial joint opening with a valgus-inducing stress. MR imaging grading of collateral ligament injuries categorized as imaging grades I, II, and III describe findings of ligamentous or periligamentous edema, ligament thickening and signal abnormality, and frank ligament discontinuity, respectively, with associated osseous edema at the ligament attachment points ( Fig. 9 ). However, MR imaging grades have shown inconsistent correlation with clinical findings. Isolated MCL injuries are unlikely to cause significant functional instability, leading some clinicians to advocate that suspected MCL injuries should be imaged only if classified as grade III clinically, to evaluate for an associated destabilizing injury to the posteromedial corner structures such as the POL, posterior capsule, semimembranosus tendon, or cruciate ligaments. In contradistinction, FCL and posterolateral corner ligament injuries are less common but pose a higher risk of instability. Whereas isolated grade III MCL injuries often respond well to conservative nonsurgical therapy, grade III FCL injuries or fracture equivalents most often undergo primary surgical repair to restore stability.
ACL injuries can be identified on MR imaging by the presence of primary and secondary signs or a combination thereof, and are often best evaluated on T2-weighted or PD-weighted imaging. Primary MR imaging signs of ACL injury include abnormal signal within the ligament, abnormal contour and configuration, or frank fiber disruption, the presence of which yield a high degree of diagnostic accuracy (see Fig. 9 ). Secondary signs include anterior translation of the tibia greater than 7 mm, characteristic bony contusions of the lateral femoral condyle and lateral tibial plateau, anterior tibial spine avulsion, or buckling of the PCL, among others. Partial-thickness ACL injuries are often difficult to accurately diagnose on imaging, likely owing to the nonspecific nature of increased instrasubstance signal that can be seen to an extent in an intact ACL, and in all grades of ACL injury. As partial-thickness injuries are commonly managed nonsurgically, MR imaging is useful to evaluate for full-thickness ACL injury or other associated soft-tissue abnormalities that would lead to progression of instability.
PCL injuries are less common than ACL injuries and most often occur in the setting of multiple injuries, which is thought to contribute in part to its underdiagnosis. Full-thickness PCL tears can be diagnosed on MR imaging if there is increased signal intensity on PD-, T1-, or T2-weighted sequences in the expected location of intact PCL fibers, visualization of frank fiber disruption or retraction, or failure to identify the PCL. Focal PCL thickening greater than 7 mm or increased intrasubstance signal may reflect a partial-thickness tear. PCL injuries are commonly associated with ACL, MCL, and posterolateral corner injuries (see later discussion).
Musculotendinous Lesions
Musculotendinous structures of the knee provide dynamic stabilization across the normal range of knee motion. The myotendinous junction of the musculotendinous unit is usually the weakest structural component and the most common site of injury. Injuries are most common in muscles that cross 2 joints and demonstrate eccentric contraction (contraction while lengthening), including the semimembranosus, rectus femoris, biceps femoris, and medial head of the gastrocnemius. Musculotendinous injuries can be categorized as muscular contusion, tendon avulsion, or myotendinous strain. Increased fluid within the injured soft tissue is common to all grades of musculotendinous injury in the acute setting, and is best visualized with STIR or T2-weighted FS sequences oriented in the short and long axes of the imaged muscle. T1-weighted sequences are also useful to evaluate for architectural abnormality, associated hematoma, or fatty infiltration and atrophy.
Although multiple classification systems of strain injuries have been proposed, they are most commonly graded according to combined clinical and MR imaging findings. Low- to moderate-grade injuries usually show a “feathery” pattern of increased signal within the muscle, with small areas of frank fluid signal representing partial tears of muscle fibers on MR imaging ( Fig. 10 ). High-grade injuries generally show near full-thickness to complete rupture of the myotendinous unit with a hematoma or fluid-filled gap in the myotendinous unit and varying degrees of tendon retraction on MR imaging, and clinically present with near complete loss of muscle function. Most musculotendinous injuries are treated conservatively, depending on the involved muscle and degree of clinical dysfunction. However, myotendinous injuries should be distinguished from avulsion fractures of the adjacent osseous attachment, as avulsion injuries are often best treated with early surgical repair if displaced more than 2 to 3 cm.
Meniscal Lesions
The menisci are fibrocartilaginous structures that distribute axial load-bearing forces across the articular surface and increase the surface area for femoral condylar motion, ultimately improving joint stability. Thus meniscal injuries can contribute to knee instability and predispose patients to knee pain, locking, joint maltracking, and early degeneration. The medial meniscus is attached along its peripheral circumference to the joint capsule, closely approximated with the deep fibers of the MCL, rendering the medial meniscus vulnerable to damage with associated MCL injury. The lateral meniscus is more loosely approximated with the lateral capsule and has no direct attachment to the FCL, but is dynamically posteriorly displaced with initiation of knee flexion because of its attachment to the popliteus tendon.
The normal menisci are uniformly hypointense structures on virtually all MR imaging sequences, with a characteristic morphology. Increased signal within the abnormal meniscus in an adult patient is best seen on T1-weighted, PD-weighted, or gradient-echo sequences, and can reflect mucoid degeneration if the signal is punctate, amorphous, or linear in morphology but confined within the substance of the meniscus. Meniscal tears are diagnosed if the signal abnormality extends through the meniscus and comes into contact with the adjacent articular surface, or if the meniscus is morphologically abnormal. Secondary signs of meniscal injury include an underlying bone contusion or osteochondral lesion, presence of parameniscal cysts, peripheral meniscal extrusion, soft-tissue edema, or adjacent effusion.
Traumatic meniscal tears most commonly occur with femoral rotation against a fixed tibia during flexion or extension, with the resulting shear force applied to the meniscal surface, most often producing a vertical longitudinal tear morphology. Longitudinal tears usually occur in the peripheral one-third of the meniscus, begin in the posterior horn and propagate anteriorly, and are often associated with ACL tears. Traumatic vertical longitudinal tears can often displace or flip and can take on a bucket-handle configuration if extensive, occurring more commonly in the medial meniscus. In the case of ACL disruption attention should be directed to the posterior root of the lateral meniscus, which can sustain an associated tear, possibly because of traction on the meniscofemoral ligament of Wrisburg during the ACL injury. Traumatic radial meniscal tears can also occur; these are vertical tears arising from the free edge and extending peripherally, with or without combined complex or horizontal tear morphologies. Meniscal root tears and traumatic radial tears should be suspected in the presence of peripheral meniscal extrusion, compromising the ability of the meniscus to counteract stresses from axial loading. Extrusion is thought to predispose the patient to accelerated articular cartilage loss in the affected compartment, although a causal relationship has not been definitively demonstrated. In contradistinction, displaced or flipped longitudinal tears show normal resistance to axial stresses and can demonstrate meniscal intrusion, which is a meniscal fragment displaced toward the intercondylar notch, best identified on coronal MR images ( Fig. 11 ).
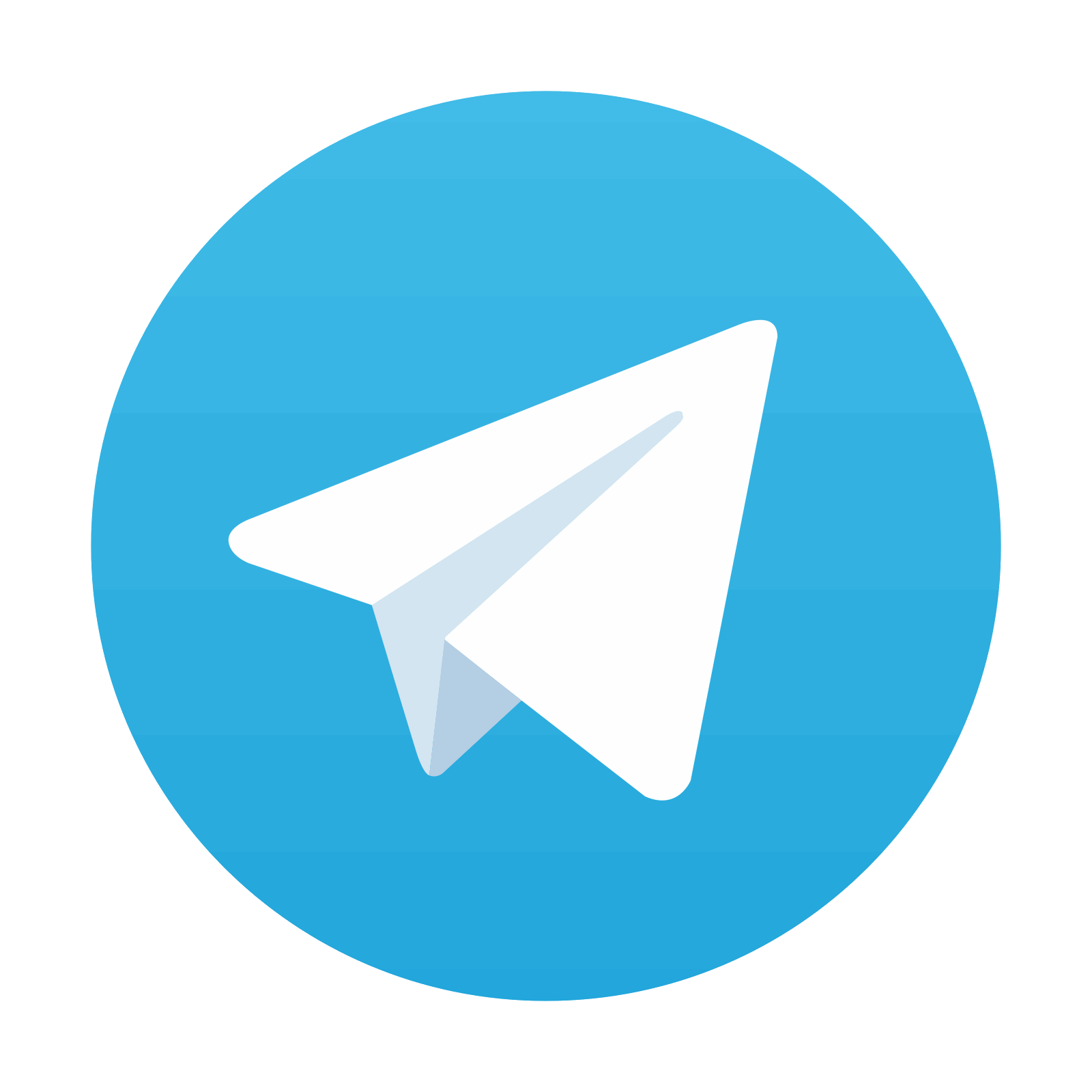
Stay updated, free articles. Join our Telegram channel

Full access? Get Clinical Tree
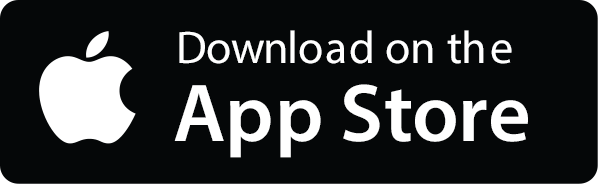
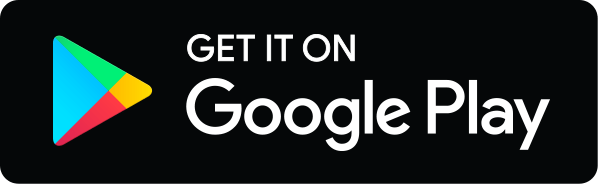
