principle are vitally important for the pediatric angiographer. Angiography, particularly during lengthy percutaneous interventions, has the potential to deliver the highest radiation dose of any imaging study. Methods to reduce radiation exposure during fluoroscopy include the use of pulse fluoroscopy, last-image hold, copper filtration, optimal collimating, and removal of anti-scatter grids while imaging neonates and small infants.
the peritoneal ligaments are the greater sac and the lesser sac, also known as the omental bursa.
TABLE 20.1 Anatomic Spaces of the Peritoneal Cavity | |||||||||||||||||||||||||||||||||
---|---|---|---|---|---|---|---|---|---|---|---|---|---|---|---|---|---|---|---|---|---|---|---|---|---|---|---|---|---|---|---|---|---|
|
TABLE 20.2 Collateral Pathways of Mesenteric Arteries | ||||||||
---|---|---|---|---|---|---|---|---|
|
This artery is the smallest of the mesenteric arteries and nourishes the distal transverse colon, descending colon, sigmoid colon, and rectum. The major branches of the IMA are the left colic, sigmoid, and hemorrhoidal arteries, all of which branch off to the left. The left colic ascends from its origin off the IMA to anastomoses with branches from the SMA. In ˜12% of individuals, the left colic artery is absent. In this situation, the perfusion of the descending and sigmoid colon is provided by the colosigmoid artery. Occasionally, the left colic artery may arise from the SMA. The left colic artery extends cephalad to the splenic flexure in the majority of patients and reaches the mid-aspect of the transverse colon in ˜15% to 20% of patients. However, perfusion of the splenic flexure is highly variable, and in some patients, the middle colic artery may be the only artery irrigating this territory.
Typically an omphalocele is diagnosed on prenatal US showing an anterior midline herniation with a covering membrane (Fig. 20.8) and the umbilical cord vessels seen inserting at the base of the hernia.
mesodermal development, leading to urinary tract distention, abnormal abdominal wall muscular development, and failure of testicular descent.16,17 The abdominal wall defect classically consists of disorganized central abdominal wall muscles that are infiltrated with collagen bundles.18 Cryptorchidism has been attributed to both impeded testicular descent by the distended urinary system as well as atresia of the gubernaculum, which does not pull adequately.16
be accompanied by fever or malaise. Most commonly, cellulitis is a complication of an overlying skin disorder, such as a penetrating wound (including a puncture, abrasion, or bite), ulcer (e.g., varicella lesions or newborn omphalitis), or dermatosis.27,28 Abdominal wall cellulitis in children may also be a sequela of abdominal surgery, such as appendectomy. Most cases of cellulitis are due to infection by Streptococcus and Staphylococcus skin flora. Less typical organisms include oral flora in cases of cellulitis secondary to a bite or Gram-negative rods, anaerobic flora, and fungi in immunocompromised patients.28
(<5 per million annual incidence) arise mostly in young adults and less commonly in children, with a slight female predominance and peak incidence in the third and fourth decades.31 Desmoid tumor locations are traditionally classified as intraabdominal (mesenteric or pelvic), abdominal wall, or extra-abdominal (most commonly in the proximal extremities, head, and neck). Patients with Gardner-type familial adenomatous polyposis (FAP) have a >800-fold increased incidence of desmoids, which are usually intra-abdominal or mesenteric in location.32,33 Abdominal wall desmoids occurring in the rectus abdominis or internal oblique muscles have an association with pregnancy.34 Desmoid tumors usually occur as single tumors, although ˜15% are multiple.34
In children, soft tissue sarcomas occurring in the abdominal wall are primarily nonrhabdomyosarcoma soft tissue sarcomas (NRSTS). Such NRSTS are a heterogeneous group of histologic subtypes derived from mesenchymal cells, which often have characteristic genetic translocations that aid in their diagnosis.40 This includes infantile fibrosarcoma, which is a rare NRSTS that occurs specifically in infants under 2 years of age (median age 3 months) and presents typically as a large infiltrative soft tissue mass with associated skin discoloration that may mimic vascular malformation.42 Synovial sarcoma (Fig. 20.14) is one of the common sarcomas in older children. All NRSTS typically present as a slowing growing and painless mass.
primarily as a result of high-energy lap-belt trauma during motor vehicle collisions or from low-energy falls onto bicycle handlebars (the so-called handlebar hernia).49,50,51 The vast majority of these injuries that are bicycle related are in boys, with a mean age of ˜10 years.51
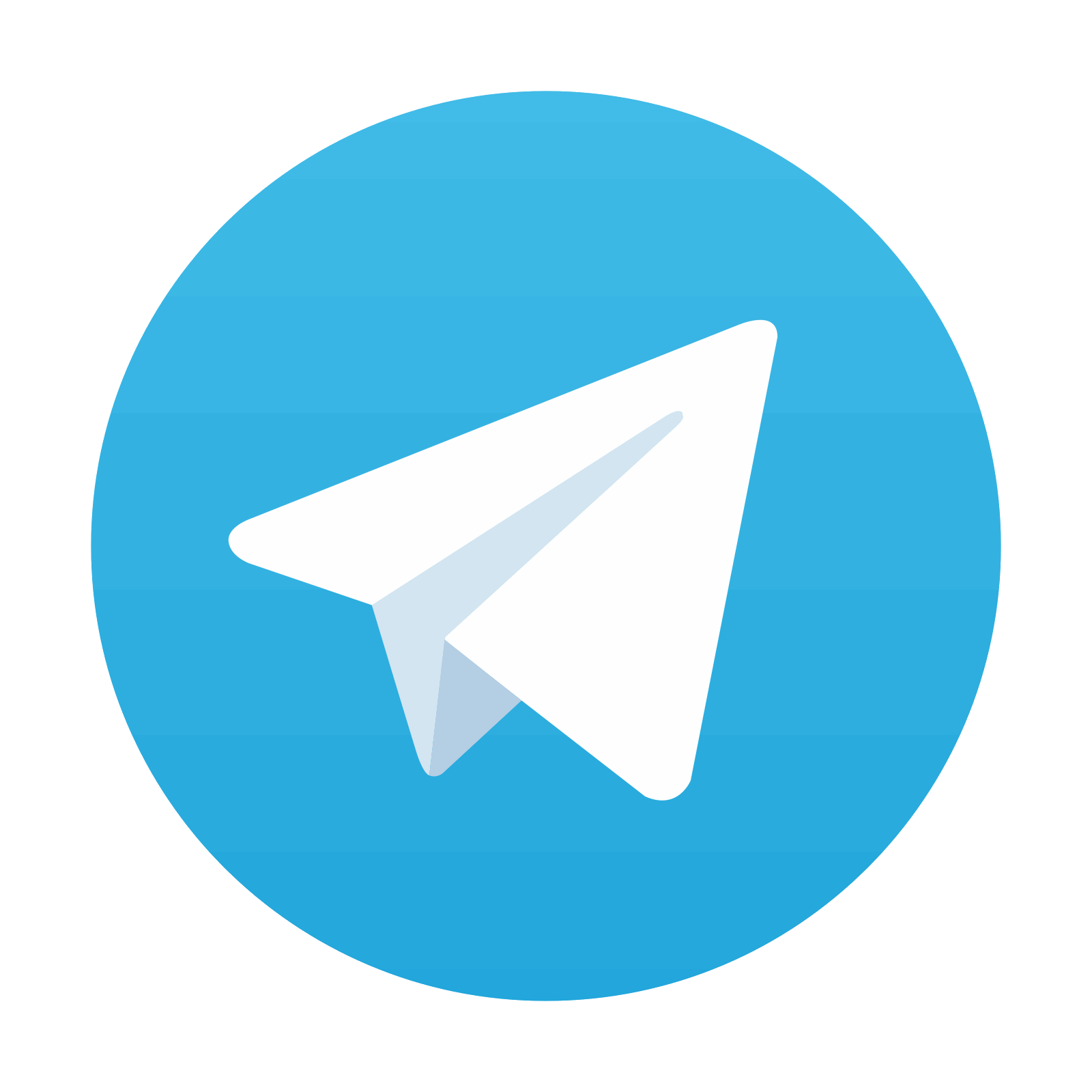
Stay updated, free articles. Join our Telegram channel

Full access? Get Clinical Tree
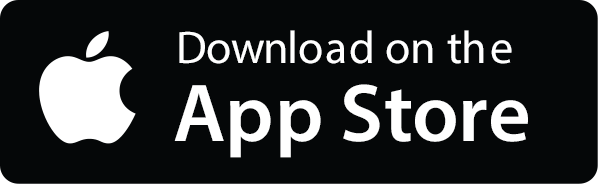
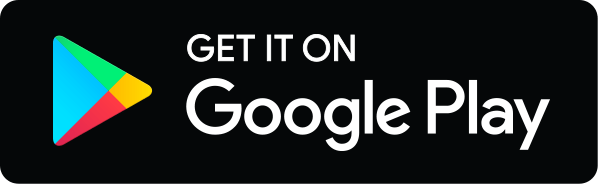
