Approximately 550,000 Americans will suffer an acute myocardial infarction (AMI) each year. Despite major advances in AMI therapies, mortality and incident heart failure (HF) remain significant problems. Five-year mortality is estimated at 36% in men and 47% in women. The five-year incidence of HF ranges from 16% in men to 22% in women. These adverse outcomes have been unequivocally linked to the development of significant left ventricular (LV) dilation and reduced systolic function after AMI, otherwise known as post-MI negative LV remodeling.
LV remodeling refers to structural and functional myocardial changes that can occur in response to physiologic stress such as exercise or because of a pathologic insult such as AMI. Postinfarction LV remodeling is an intricate cascade of biologic events set forth by the release of intracellular chemokines from necrotic myocytes and increased wall stress due to infarct region contractile and diastolic impairment. Remodeling is a healing and compensatory process that restricts myocardial damage while maintaining cardiac output.
Multiple studies have demonstrated different patterns of post-MI remodeling with varying degrees of LV dilation. LV dilation may be transient, limited or progressive. Differences in the onset of dilation have been observed. It can occur acutely (within 10 days) and/or later during the healing process. Progressive dilation has been observed even years after MI. Controlled ventricular remodeling is ultimately achieved by the formation of a strong collagen scar and remote myocardium hypertrophy which counteract intracavitary forces, thereby limiting progressive ventricular dilation. In this case, ventricular remodeling is compensatory. However, in many instances, particularly with large transmural infarcts, the remodeling process is overwhelmed by the increased LV wall stress. Ongoing dilation and remote contractile impairment ensues ( Fig. 20.1 ) in a process known as negative postinfarction LV remodeling, which increases risk of HF and mortality.
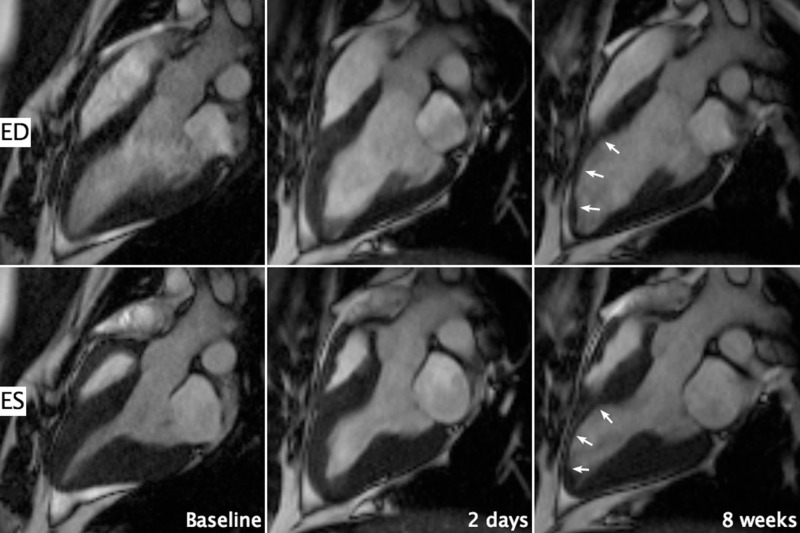
The prognostic significance of negative post-MI remodeling has been demonstrated with the use of various imaging modalities, including chest radiographs, invasive x-ray left ventriculography, radionuclide ventriculography, and echocardiography. In 1973 Kostuk and colleagues showed various patterns of LV remodeling and the association between the extent of negative remodeling and clinical outcomes by measuring serial left heart dimensions using calibrated chest radiographs. Subsequent investigations established LV ejection fraction (EF) as a strong predictor of survival after AMI. By 1987 White and colleagues found that LV end-systolic volume (ESV) measured by x-ray left ventriculography 1 to 2 months after MI was a more powerful predictor of survival than end-diastolic volume (EDV) and LVEF.
Although these modalities have laid the foundation of our understanding of post-MI LV remodeling, their utility has been hindered by technical and safety limitations. Chest radiographs are insensitive to RV and LV volumes and systolic function. Invasive x-ray left ventriculography and radionuclide techniques expose patients to potentially harmful ionizing radiation, making their routine repeated use in humans less desirable. Echocardiography is limited in a subset of patients by the availability of acoustic windows for proper endocardial definition. Finally, two-dimensional (2D) modalities, such as x-ray left ventriculography and echocardiography, rely on geometric assumptions to calculate ventricular volumes that may not apply in hearts regionally deformed by MI.
Cardiovascular magnetic resonance (CMR) is a technique that can overcome many of the limitations mentioned above. As a result it has emerged as a valuable noninvasive modality for the assessment of cardiac disease. CMR provides a comprehensive, volumetric, accurate, and reproducible cardiovascular evaluation beyond ventricular volumes. With the use of CMR it is possible to characterize the myocardial tissue for the localization and quantification of myocardial edema, microvascular obstruction (MVO), intramyocardial hemorrhage, and infarct burden. Hence, CMR is particularly well suited for the evaluation of post-MI remodeling. In this chapter we will review the research and clinical utility of CMR in the evaluation of post-MI ventricular remodeling,
Ventricular Volumes, Ejection Fraction, and Mass
LV cavity dilation and systolic dysfunction are the hallmarks of postinfarct remodeling and can be accurately quantified with high spatial and temporal resolution using cine CMR. Early breath-hold cine imaging used fast low-angle shot (FLASH), a spoiled gradient recalled echo (GRE) sequence. Currently, balanced steady-state free precession (bSSFP) is the most commonly used pulse sequence because of faster acquisition times, improved temporal resolution, and optimal contrast between the myocardium and the blood pool. The use of bSSFP facilitates qualitative and quantitative assessment of cardiac anatomy and function mainly by improving endocardial border definition.
Unlike echocardiography, acoustic windows do not limit CMR; therefore images can be acquired in any desired orientation to accurately depict LV morphology and function. A carefully prescribed stack of short-axis slices extending from the cardiac base to the apex provides a three-dimensional (3D) dataset that can be used to make accurate ventricular morphologic measurements at end diastole (ED) and end systole (ES) without the need for geometric assumptions, as is the case with 2D modalities ( Fig. 20.2 ). Ventricular volumes are calculated using the summation of disks method.
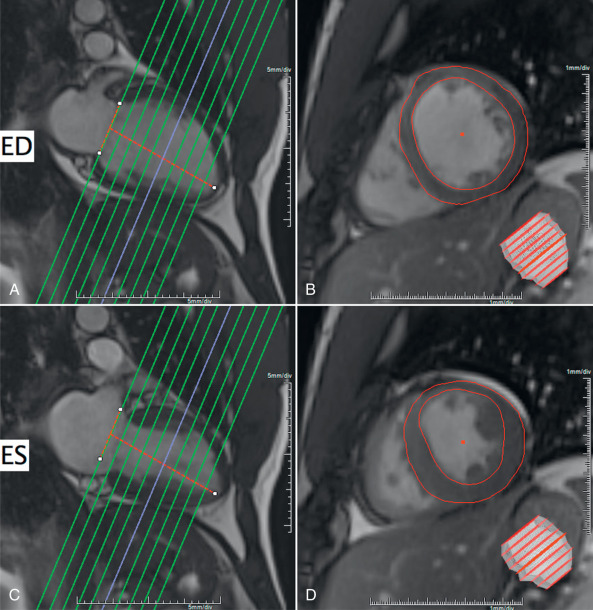
CMR derived LV volumes and mass have been validated with phantom, animal and human studies and currently considered the clinical reference standard for other imaging modalities. Although accuracy is important, reliability may be even more important because it dictates the validity of differences between serial examinations. Volumetric measurements by CMR provide high-interstudy, interscan, and interobserver reproducibility in normal hearts and those deformed by prior MI. Interstudy, interobserver, and intraobserver reproducibility of CMR-derived RV volumes is also excellent in healthy controls, patients with HF, ventricular hypertrophy, and congenital heart disease. Because of improved reproducibility compared with echocardiography, CMR facilitates the identification of clinically significant ventricular changes in serial examinations after AMI. The reduced variability of the measurement significantly reduces the sample size needed to detect differences between treatment arms in clinical trials.
Cine CMR has been successfully used to evaluate post-MI remodeling in animal models and humans. Konermann and colleagues studied the reliability of CMR in the evaluation of LV morphology and function in post-MI patients. They compared cine CMR LV volumes and LVEF with 2D echocardiography (2DE), radionuclide, and x-ray left ventriculography in a group of 65 patients who suffered a transmural MI and did not undergo thrombolysis or angioplasty. Good correlation was found between x-ray and radionuclide ventriculography. However, correlation with 2D echocardiography was limited because of inconsistent 2DE image quality. In this study, the investigators also demonstrated good correlation between creatine kinase (CK)-derived infarct size and CMR infarct mass determined from cine images.
In a follow-up publication, Konermann et al. reported the natural history of LV remodeling in the same group of 65 patients at 1, 4, and 26 weeks post-MI. Medical therapy was limited to nitrates, beta-blockers, aspirin, and diuretics. Only those patients with first transmural infarcts were included in the study. Infarct transmurality and size were defined by clinical, electrocardiographic, and enzymatic criteria. The extent of negative remodeling was not only dependent on the enzymatic size but also on the location of the infarct. Small infarcts caused modest but concordant ED and ES dilation, so that LVEF remained stable. Individuals with large anterior infarcts developed an unbalanced, progressive diastolic and systolic dilation that resulted in a marked reduction in LVEF. Infarct region thinning was almost always observed at 6 months except for small posterior infarcts. LV stroke volume index (SVI) was smaller in large anterior MI, but there was no statistically significant difference in LV SVI between 1 and 26 weeks post-MI. Despite a significant LVEF reduction in those with large infarcts, stroke volume was maintained at the expense of ventricular dilation. No clinical difference between the groups was observed when analyzing New York Heart Association (NYHA) symptom classification. Four patients died before the completion of the study and all had large anterior MI.
In a cohort of 26 patients with reperfused anterior AMI, Kramer et al. used cine tissue tagging to evaluate morphologic changes, LVEF, and regional intramyocardial function of myocardium adjacent and remote to the infarct. All patients received either angiotensin-converting enzyme inhibitor (ACEI) or beta-blocker. Most patients received ACEI and about half received both agents. Imaging was done on day 5 ± 2 and week 8 ± 1 after MI. There was a significant increase in LV end-diastolic volume index (EDVI) with stable end-systolic volume index (ESVI). Therefore there was an improvement in global LVEF 8 weeks post-MI. The increased LVEF was mediated by contractile improvement and normalization in the adjacent and remote regions, respectively. Enzymatic infarct size correlated with the degree of diastolic dilation.
Regional Contractility
Although LVEF is the most commonly used global metric of LV systolic function, it does not provide information regarding regional performance. In fact, segmental systolic abnormalities because of AMI may sometimes be concealed by a normal global LVEF. Accordingly, to understand the contributing factors to global functional alterations during the remodeling process, it is important to characterize regional contractile performance in the adjacent and remote noninfarcted myocardium. In clinical practice this is usually done by subjective visual scoring of segmental endocardial motion and thickening using cine CMR images. However, visual assessment can be insensitive to subtle abnormalities and is subject to greater interobserver variability. Thus objective methods to quantify contractile function have been developed. Wall thickening and endocardial motion can be measured from cine CMR images. A more accurate assessment of intramyocardial contractility in the infarcted heart can be determined by measuring strain with the use of myocardial tissue tagging sequences such as spatial modulation of magnetization (SPAMM) and phase-based sequences such as displacement encoding with stimulated echoes (DENSE). More recently, feature tracking analysis has allowed the measurement of circumferential and longitudinal strain from bSSFP cine images.
Wall Thickening and Endocardial Displacement: Cine Cardiovascular Magnetic Resonance
In their chronicle of nonreperfused AMI, Konermann et al. assessed regional LV contractility by measuring wall thickening and the endocardial motion toward the center of the LV cavity using cine CMR. Progressive changes in endocardial motion were identified in the infarct and remote regions during the 6-month follow-up period. The extent of regional LV dysfunction correlated with infarct size and location. The infarct region of large anterior AMI had the greatest reduction in endocardial motion, which progressed to dyskinesis (displacement away from the cavity center) at 6 months. Changes in wall thickening were not observed in the viable myocardium. It is worth noting that the authors averaged the adjacent and remote myocardium wall thickness in their analysis. Hence, regional differences could not be identified between these segments.
Holman et al. investigated the regional contractility of 25 patients 3 weeks after anterior MI. An optimized 3D analysis of the myocardium centerline was implemented to improve accuracy of wall thickness measurement. Some 100 equidistant chords were constructed between the endocardial and epicardial contours of short-axis cine images. The starting point was defined as the inferior RV insertion site, which was labeled for ED and ES, allowing to correct for rotational motion. Wall thickening was reduced in the left anterior descending (LAD) artery territory compared with a normal database. The dysfunctional LV myocardial mass 3 weeks after MI, when any myocardial stunning should have resolved, correlated with enzymatic infarct size.
Strain Imaging
Tissue Tagging
CMR strain imaging in animal and human studies has contributed greatly to our understanding of regional myocardial function during the post-MI remodeling process. In an ovine model of surgical LAD ligation, SPAMM myocardial tissue tagging was used to measure circumferential and longitudinal myocardial shortening at baseline, 1 week, 8 weeks, and 6 months after AMI. Shortening within infarcted regions was reduced throughout the study period. A persistent difference in intramyocardial shortening was found between noninfarcted regions adjacent to and remote from the infarct border. Function in adjacent noninfarcted regions fell markedly at 1 week after AMI and partially improved by 8 weeks, but remained depressed relative to baseline and to remote regions at 6 months. These findings were reproduced by Moulton et al. using a similar ovine model and DANTE (delays alternating with nutations for tailored excitation) myocardial tagging. In addition to systolic strain, Moulton et al. evaluated diastolic and isovolemic strains. They found abnormal, positive strain during isovolemic contraction in the border zone, suggesting that isovolemic myocardial fiber stretching contributes to LV systolic dysfunction in these segments.
Epstein et al. used myocardial tissue tagging to characterize acute regional function in a murine model of LAD occlusion and reperfusion. The percent circumferential shortening was measured for the infarct, adjacent and remote regions. A gradient of contractile dysfunction was found from the infarct to the remote region 1 day post-AMI compared with baseline. Thomas et al. used tissue tagging to characterize regional contractile changes in a rat model of LAD ligation at 1 to 2, 3 to 4, 6 to 8, and 9 to 12 weeks post-AMI. In this study, changes in the maximum and minimum principal stretches and strains, and the orientation of the principal stretch angle were measured. At 1 to 2 weeks post-AMI, significant changes were found in a gradient fashion from the infarct to the remote region. These abnormalities persisted relatively unchanged up to 12 weeks post-MI. The principal strain direction became more circumferentially oriented during the study period.
Using CMR tissue tagging in a cohort of patients within the first week after reperfused LAD AMI without significant disease in other coronary territories and with an EF of <50%, Kramer et al. found a significant reduction in remote region intramyocardial shortening compared with controls. Once again, there was a decrease in contractile function in all myocardial segments in a gradient fashion similar to the findings in animal studies. When this patient group was re-imaged at 8 weeks after AMI, there was improvement in regional function in all segments, including normalization of contractile function in the remote myocardium. The impact of early reperfusion is evident by the improvement in infarct region strain.
CMR myocardial tagging was used to assess the correlation between regional function and loading conditions in 16 patients after successful reperfusion of a first anterior MI compared with 31 age-matched controls. All patients had received optimal medical therapy with beta-blockers and ACEIs. Imaging was performed 1 and 12 weeks postinfarction. The LV myocardium was divided into 32 cuboid segments, defined by 4 endocardial and 4 epicardial node points derived from short- and long-axis tagged images. Regional LVEF was calculated using a pie-shaped volume defined by the endocardium and the center of the LV. This parameter was viewed as a composite marker of regional deformation. A relative metric of regional load was defined as the product of the systolic blood pressure and the mean radius of curvature in the short and long axes divided by the segment wall thickness. In healthy controls, an inverse relationship between regional LVEF and load was demonstrated. In contrast for the MI patients, the average load values significantly increased in a graded fashion from remote to infarct segments between the acute and chronic time points. At 12 weeks, remote regional LVEF decreased with a similar correlation coefficient to changes in load as controls; therefore the change in LVEF was because of an increase in loading conditions and a worsening of myocardial function. No change in regional LVEF was observed in the adjacent segments, indicating some improvement in myocardial function. The infarct segments demonstrated an average increase in regional LVEF even with significant increases in load representing an even greater true improvement in myocardial function. This study demonstrated that CMR could be used to assess LV function post-MI and differentiate improvement in intrinsic myocyte function from changes in deformation that occur as a response to alterations in Frank-Starling conditions.
Phase-Based Strain Imaging
In 1999 advanced, phase-based, strain imaging techniques were introduced to facilitate and optimize strain analysis compared with tissue tagging methods. These included harmonic phase magnetic resonance (HARP) and DENSE. These methods were validated with traditional tagging sequences in animal and human studies.
Azevedo et al. used tissue tagging with HARP analysis in combination with contrast-enhanced (CE)-CMR and radioactive microspheres to evaluate the relationship between strain properties and myocardial injury in a canine model of 90-minute LAD or left circumflex (LCX) occlusion followed by 24 hours of reperfusion. In this early period the areas at risk as defined by radioactive microspheres with preserved systolic strain and strain rate demonstrated significantly reduced diastolic strain rate compared with the remote regions. Similar to the findings of Gerber et al., infarct segments with MVO demonstrated even greater reduction in systolic and diastolic strain than those without.
In a canine model of reperfused LAD, Ashikaga et al. used DENSE to generate 3D-displacement maps, CE CMR imaging to generate infarct maps, and epicardial electrical recordings to generate electrical activation maps. CMR examinations were completed between 3 and 8 weeks post-AMI. Electrical activation times were significantly delayed in the infarct zone, but preserved in the border and remote areas. Conversely, all strain parameters were impaired in the border zone, which was not different from the infarct region. Hence, contractile abnormalities in the border segments were not mediated by impaired electrical activation. In the remote segments there was a typical transmural strain gradient from subendocardium to subepicardium, which was not observed in the infarct or border zone regions. Positive circumferential and longitudinal strains were observed in the border zone indicative of abnormal systolic stretch.
In a canine model that typically created subendocardial infarcts with large areas at risk, recovery of systolic function was evaluated with DENSE imaging and correlated to T2-weighted (T2W) imaging representing the area at risk. T2W area at risk was validated with microsphere measurements. At 2 months post-AMI there was resolution of the T2W abnormalities representing the area at risk. This correlated with a significant improvement in radial and circumferential strain compared with the acute post-MI setting. However, contractile function in the area at risk remained significantly depressed compared with remote sectors.
Feature Tracking
More recently anatomical feature tracking of cine bSSFP images or feature tracking CMR has been introduced as a practical solution for strain analysis. Although the information obtained via this method is mostly limited to the endocardium and epicardium, it still provides relevant and comparable information when compared with traditional tissue tagging. Its application to post-MI remodeling has been limited ; however, given its ease of use, it is likely to rapidly gain popularity as it has in many other disease states.
Myocardial Fiber Structure: Diffusion Tensor Magnetic Resonance Imaging
An evolving technique to study post-MI remodeling is diffusion-weighted magnetic resonance imaging (MRI). The ability to visualize and characterize the myocardial fiber structure is important to better understanding cardiomyopathies, including post-MI remodeling. Diffusion tensor imaging (DTI) is a form of diffusion-weighted CMR which has been validated against histology as a rapid and nondestructive method to depict the myocardial fibers’ architecture. Early animal and human studies have consistently shown that early after MI, the infarct region is characterized by increased diffusivity and decreased fractional anisotropy. Investigators have also shown a difference in the distribution of right- and left-handed helix fibers between the infarct and remote regions. In a study of 37 patients imaged about 4 weeks after their first MI, a transmural gradient of left- and right-handed helical fibers was observed between the infarct and remote regions. In the infarct region the percent of right-handed fibers was lowest in association with a greater percent of left-handed fibers. Using 3D DTI tractography, Sosnovik et al. performed in vivo imaging of mouse hearts 24 hours and 3 weeks after mid-LAD infarct or ischemia-reperfusion injury. DTI imaging findings were compared with CMR and histologically determined infarct and area at risk regions. Areas of increased T2, representing tissue edema, demonstrated increased diffusivity and decreased fractional anisotropy as well as loss of fiber tracts. The loss of tract coherence was uniform across the area at risk and included infarct and viable fibers when compared with late gadolinium enhancement (LGE) and histopathology. In the infarct group there was persistent loss of fiber tracts 3 weeks post-MI. Therefore presence of collagen fibers did not restrict diffusion adequately to resolve tracts by DTI. In the ischemia-reperfusion group, diffusivity and fractional anisotropy returned to baseline 3 weeks after the injury; however, fiber architecture remained abnormal, albeit relatively preserved compared with infarcted hearts.
Tissue Characterization
To thoroughly understand post-MI remodeling, it is crucial to precisely characterize the location, size, transmural extent, and type of ischemic injury. In the event of epicardial vessel occlusion, the myocardium subtended by the vessel segments distal to the occlusion is at risk of infarction. This territory is known as the area at risk. A sufficiently prolonged occlusion leads to irreversible injury, or infarction, of the entire area at risk. If a vessel is opened before the occurrence of permanent injury, a percentage of the area at risk is salvaged. The salvaged myocardium exhibits reversible contractile dysfunction, or stunning. A more deleterious form of injury can occur within the infarct core because of persistent ischemia or “no-reflow” caused by MVO. In a number of infarcts with MVO, intramyocardial hemorrhage may also occur. CMR stands out from other imaging modalities because of its superior tissue characterization capability. With the use of various noncontrast and contrast-enhanced CMR sequences, we can identify and quantify the area at risk, areas of irreversible infarction, salvaged myocardium, MVO, and intramyocardial hemorrhage, which are all important parameters that influence post-MI remodeling.
Infarct Characterization and Predictors of Left Ventricular Remodeling
Infarcted myocardium can be identified by various CMR pulse sequences, including cine, CE-CMR, and, more recently, T1 mapping. Today, CE-CMR, specifically LGE, is considered the reference standard for acute and chronic infarct characterization.
Late Gadolinium Enhancement
LGE images are acquired at least 10 minutes after gadolinium (Gd) administration using an inversion-recovery T1-weighted (T1W) pulse sequence. Gd shortens the T1 relaxation of the surrounding protons; therefore areas of increased Gd appear hyperintense on T1W imaging. Although Gd-based contrast media are extracellular, the loss of cell membrane integrity and altered Gd wash-in and wash-out kinetics lead to increased Gd in acute infarcts. In chronic MI, Gd is increased because of an increase in extracellular space, with formation of a collagenous scar as well as alterations in contrast kinetics. Consequently, both AMI and chronic MI will appear as bright myocardial regions on LGE imaging ( Fig. 20.3 ).
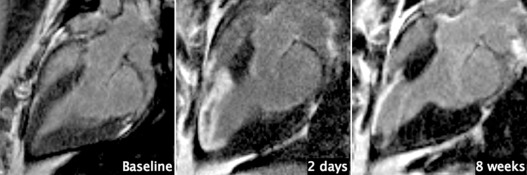
LGE imaging precisely and reproducibly demonstrates acute and chronic infarcts in comparison to histology. This was best demonstrated in an elegant canine study by Kim et al. in which acute infarcts were created by coronary ligation. A second artery was instrumented to create reversible ischemic injury. CMR examinations were performed at 1 day, 3 days, and 8 weeks postischemic injury. LGE images were acquired 30 minutes after Gd administration. Compared with histology, the extent of LGE by ex vivo imaging was the same as the extent of myocardial necrosis in the acute phase and the extent of collagenous scar at 8 weeks after reperfused and nonreperfused ischemic injury. Therefore areas of LGE specifically represent permanent myocardial injury and not stunned or salvaged myocardium. It should be noted that the accuracy of in vivo LGE is influenced by multiple factors, including imaging delay after contrast injection, spatial resolution, and postprocessing methodology. When a standardized approach is applied, LGE imaging reproducibly quantifies acute and chronic infarcts with excellent interscan, intraobserver and interobserver agreement.
This technique has been useful to define the evolution of infarct size in vivo. Rochitte et al. demonstrated an increase in infarct size from 2 to 48 hours after reperfused 90-minute LAD occlusion in a canine model. However, the investigators did not report the changes in wall thickness or circumferential expansion index to further characterize the pattern of infarct size growth in this very early phase after ischemic injury. They also observed up to 3-fold increase in the size of MVO in the infarct core, which correlated with thioflavin-S myocardial blood flow assessment. Fieno et al. assessed chronic infarct remodeling in canines after 45-minute, 90-minute, or permanent LAD occlusion. Imaging was performed on day 3, 4 weeks, and 8 weeks after MI. The infarct size at 8 weeks post-AMI decreased on average by 75% of the size on day 3, but as much as 90% in the case of small infarcts caused by the 45-minute occlusion. True infarct expansion was not observed. Although the overall myocardial mass decreased, the noninfarcted myocardium mass increased on average by 15%. The infarct involution findings were consistent with those of Richard et al., who measured infarct size on pathologic specimens 4 days, 2 weeks, and 6 weeks after 6 hours of reperfused or permanent circumflex occlusion in an open chest canine model. In both experiments, reperfusion appeared to accelerate infarct healing, but did not impact the final infarct size. Early infarct expansion and cardiac rupture were not observed in these studies.
Infarct involution has been demonstrated in humans as well. Ingkanisorn et al. evaluated infarct size within 5 days of an AMI in 33 patients, 20 of whom returned for follow-up imaging more than 8 weeks after MI. Acute infarct size correlated well with troponin-I. At follow-up, infarct size decreased from 16% to 11% of the LV mass. Choi et al. also reported a significant decrease in infarct size between week 1 and week 8 post-MI in 25 patients after reperfused AMI. The involution of MI size tended to be greater in those with MVO.
LGE CMR has been recognized as a powerful predictor of LV remodeling, regional and global functional recovery, and major adverse cardiac events (MACE) following AMI. Choi et al. performed LGE on 24 patients within 7 days of revascularization post-MI. Scans were repeated at 8 to 12 weeks to assess functional recovery. There was an inverse relationship between transmural extent of LGE and segmental recovery. Interestingly, the extent of dysfunctional myocardium with zero or <25% transmural enhancement was a better predictor of global functional recovery than peak cardiac enzyme level or total infarct size by LGE. Ingkanisorn et al. found a better correlation between acute LGE infarct size and follow-up LVEF than between acute LVEF and follow-up LVEF. This is probably caused by the presence of stunned, yet viable, myocardium in the setting of early reperfusion. Lund et al. imaged 55 patients within 1 week of reperfused MI and approximately 8 months later. LGE infarct size of 24% of the LV area predicted LV remodeling, as defined by an increase in EDV index of ≥20%, with a sensitivity and specificity of 92% and 93%, respectively. In a cohort of 231 patients with prior MI (at least 3 months), Di Bella et al. found that LGE >12.7% of LV mass, EDV >105 mL/m 2 , and a wall motion score index >1.7 were independently associated with cardiac death or appropriate intracardiac defibrillator shocks. Patients with all three factors had a 4-year event rate of 29.6%, whereas those with none of these factors had an event rate of 3.5%.
No-Reflow Phenomenon: Microvascular Obstruction and Intramyocardial Hemorrhage
It has been shown in animal and clinical studies that opening an occluded epicardial artery does not always result in homogeneous reperfusion of the area at risk. This is known as the “no-reflow” phenomenon. Areas of no-reflow can be detected in vivo by various modalities, including angiography, single-photon emission computed tomography (SPECT), positron emission tomography (PET), and contrast echocardiography, but are best evaluated by CE-CMR. In the CMR literature, no-reflow zones have been referred to as areas of MVO. Areas of MVO are detected by CE-CMR as hypoenhanced regions within the infarct core on dynamic first-pass perfusion imaging during Gd administration or on LGE imaging after Gd administration ( Fig. 20.4 ). LGE imaging 2 to 5 minutes after contrast injection reveals so-called early MVO and imaging after 10 minutes reveals late MVO. It has been demonstrated that areas of early MVO tend to be larger than late MVO, which suggests that early MVO illustrates a penumbra of slow collateral flow in addition to true no-reflow regions. Therefore early MVO is more sensitive, whereas late MVO is more specific for no-reflow. A recent systematic review shows that the prevalence of early MVO is greater than that of late MVO (65% vs. 54%, respectively).
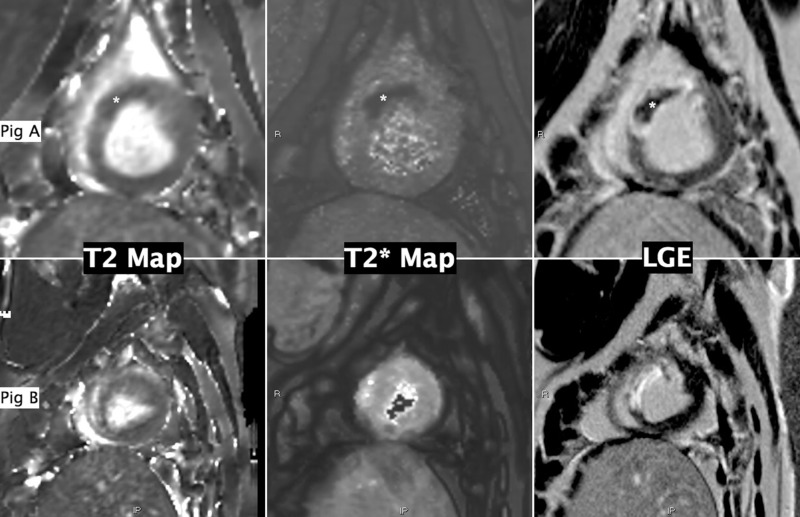
The mechanisms of the no-reflow phenomenon are incompletely understood but may include vasoconstriction mediated by inflammatory cells, external compression from surrounding edema, and microembolization of atherosclerotic cellular debris leading to small vessel plugging. Regardless of the exact mechanism, studies have demonstrated that this phenomenon is mediated by reperfusion injury. Rochitte et al. and Wu et al. showed that MVO size, as detected by in vivo CE-CMR and validated with thioflavin-S, peaks 48 hours after reperfusion and plateaus from 2 to 9 days after reperfusion. Areas of MVO involute thereafter and are rarely seen on follow-up CMR 1 month or later after AMI.
The presence of any MVO has been repeatedly associated with worse myocardial remodeling and clinical outcomes. This was first demonstrated by Wu et al., who found that early MVO predicts infarct transmurality, LV function, and remodeling after 16 months, and was also a powerful independent prognostic indicator of MACE even after controlling for infarct size. Gerber el al. studied the effect of MVO on myocardial strain and reported that the extent of MVO correlates with significant decreases in infarct region stretch and reduced radial strain in the adjacent regions. Nijveldt et al. evaluated the impact of early, mid, and late MVO in 63 patients who received percutaneous coronary intervention (PCI) and optimal medical management. They found that MVO was a better predictor of adverse remodeling at the 4-month follow-up CMR. Cochet et al. compared the prognostic significance of MVO as detected by first-pass perfusion to late MVO in 187 patients and showed that by multivariate analysis both methods are predictors of 1-year MACE. However, late MVO had an odds ratio (OR) over 3 times that of first-pass perfusion (8.7 vs. 2.5). These results are summarized in a recent systematic review by Hamirani et al., showing that both early MVO and late MVO are associated with lower LVEF, increased ventricular volumes and larger infarct size, and predict worse adverse remodeling. However, late MVO appears to portend greater risk of MACE compared with early MVO (OR 4.3 vs. 2.6), which may be a function of its improved specificity for true no-reflow zones. Furthermore, late MVO is more likely to contain intramyocardial hemorrhage, which may represent a more severe ischemic injury.
Intramyocardial Hemorrhage
Intramyocardial hemorrhage occurs as the result of capillary endothelial necrosis. In the early era of reperfusion therapy, intramyocardial hemorrhage was considered a deleterious effect of reperfusion. This idea was later challenged by Fishbein et al., who demonstrated that intramyocardial hemorrhage only occurs in areas with existing microvascular damage before epicardial reperfusion. Therefore intramyocardial hemorrhage is probably a manifestation of preexisting capillary injury and not caused by reperfusion. It is worth noting that whether intramyocardial hemorrhage is a consequence of reperfusion or a manifestation of underlying injury, it is always dependent on the duration of ischemia.
Intramyocardial hemorrhage is mostly observed in areas of no-reflow and cannot be differentiated from nonhemorrhagic MVO by CE-CMR. However, CMR is the only modality that allows in vivo visualization of intramyocardial hemorrhage with the use of T2-weighted or T2*-weighted imaging (see Fig. 20.4 ) . T2*W imaging has been validated in animals and is considered the preferred method because of improved sensitivity compared with T2W imaging.
An increasing body of literature indicates that the presence of intramyocardial hemorrhage may represent a more severe form of injury in the ischemic spectrum. However, its clinical prognostic value is not well defined. In their systematic review Hamirani et al. identified 9 studies comprising 1106 patients that evaluated the impact of intramyocardial hemorrhage on LV remodeling, and the pooled analysis demonstrates that intramyocardial hemorrhage is associated with larger ventricular volumes, infarct size, and lower LVEF. In a recent study of 151 patients, Kandler et al. also report that intramyocardial hemorrhage, as detected by T2*, was independently associated with larger infarct size, larger LV volumes, smaller salvage index, and lower LVEF. In a study of 346 patients with reperfused ST elevation MI (STEMI) within 12 hours of symptom onset, Eitel et al. evaluated the prognostic significance of intramyocardial hemorrhage, as defined by hypointense core on T2W imaging. Intramyocardial hemorrhage was observed in 35% of the patients and it was associated with larger acute infarct size, MVO size, less myocardial salvage, and lower LVEF. Using stepwise multiple Cox-regression analysis, the presence of intramyocardial hemorrhage was an independent predictor of MACE 6 months after AMI (hazard ratio 2.04), whereas late MVO alone was not. The addition of intramyocardial hemorrhage to a predictive model that included clinical history, LV function, and infarct size increased the c-statistic (area under the curve [AUC]) from 0.76 to 0.8 ( P = .046). The results of this study require confirmation in larger multicenter studies.
Bulluck et al. reported a new insight regarding the fate of intramyocardial hemorrhage in a cohort of STEMI patients who had CMR within the first week post-MI and a follow-up examination 5 months later. Review of T2 and T2* maps at both time points indicated that about 87% of those with intramyocardial hemorrhage had evidence of residual iron deposits in the infarct zone. The T2 relaxation times were higher in infarcts with residual iron deposits. Adverse remodeling was equally associated with infarct size, extent of MVO, and intramyocardial hemorrhage. Hence, myocardial iron deposits could represent an important therapeutic target in those with intramyocardial hemorrhage.
T1 Mapping: Infarct Characterization and Extracellular Volume
Although LGE is an excellent technique for infarct characterization, there is a need for noncontrast methods that facilitate the evaluation of patients with advanced renal disease who are at potential risk of nephrogenic systemic fibrosis if exposed to Gd-based contrast agents. Recently, native T1 mapping has been proposed as a noncontrast method to characterize acute and chronic MI. In the early 1980s a series of studies indicated that T1 relaxation times are prolonged in acute infarcts. However, technical difficulties prevented further investigations in this area. With the introduction of T1-mapping techniques, it is now possible reproducibly to measure myocardial T1 relaxation times with a breath-hold acquisition.
So far, T1-mapping studies after MI have reproduced earlier findings showing that native T1 relaxation times are increased in AMI and chronic MI compared with remote regions. Furthermore, native T1 values are significantly higher acutely post-MI than in the chronic phase, indicating histopathologic differences between AMI and chronic MI. Acute T1 alterations may be most influenced by tissue edema, whereas in the chronic phase replacement fibrosis represents the underlying mechanism. The diagnostic performance of native T1 mapping for the detection of STEMI and non-STEMI (NSTEMI) appears to be high (receiver operating characteristic = 0.91). The accuracy of infarct size and transmurality with T1 mapping has been inferior to LGE and seems dependent on field strength. Kali et al. were the first to prospectively compare infarct size by native T1 mapping to LGE in a canine model of reperfused LAD MI. Examinations were performed 7 days and 4 months post-MI at 1.5 T and 3 T. The infarct region was defined as the mean T1 value of the remote myocardium plus 5 standard deviations (mean ± 5 SD criterion). The LGE infarct was similarly defined by the mean ± 5 SDs of the remote signal intensity. Whereas T1 maps at 1.5 T underestimated AMI and chronic MI size, 3 T-derived maps overestimated acute infarct size, but accurately represented chronic infarcts. In a follow-up study of chronic MI in humans at 3 T, the investigators showed high sensitivity and specificity for infarct size using the mean ± 5 SD threshold method. However, sensitivity by visual assessment was significantly compromised (60% for STEMI, 64% for NSTEMI). This is because of the significantly lower contrast between the infarcted and normal myocardium native T1 as compared with the contrast between LGE regions and remote nulled myocardium. Thus further efforts are needed to optimize image contrast to improve infarct detection and quantification. Furthermore, studies of intrascan, interobserver and intraobserver reproducibility of infarct size by T1 mapping are needed.
Area at Risk and Myocardial Salvage
In the study of interventions intended to minimize ischemic injury, infarct size is not an adequate measure of therapeutic effect. This is primarily because of wide variability in the area at risk of infarction, which defines the maximum potential infarct size. The main determinants of size of the area at risk include the location of the occlusion along the length of a coronary vessel and the extent of available collateral circulation, both of which vary greatly between individuals in human studies. Furthermore, as mentioned previously, most infarcts will naturally undergo significant involution. However, there is great variability in the extent of infarct resorption and it is not possible to reproducibly predict the final infarct size based on acute measurements. Hence, an endpoint that evaluates the ischemic injury as a function of the area at risk, such as the amount of salvaged myocardium, is preferred. Compared with other markers of LV remodeling, controversy remains regarding the optimal CMR method to assess the area at risk. The main proposed techniques include T2W imaging and “early” LGE with an inversion recovery sequence or cine bSSFP.
Aletras et al. evaluated the use of in vivo T2W imaging as a method to retrospectively measure the area at risk after reperfused MI. The area of increased T2 signal correlated well with the fluorescent microspheres, and both were reported as consistently greater than the LGE and triphenyl tetrazolium chloride (TTC) areas. At 2 months post-MI there was evidence of functional recovery in the T2 areas, as demonstrated by DENSE radial strain maps, suggesting that this region contained stunned myocardium.
With the availability of LGE imaging for infarct quantification and the mounting evidence pointing to T2W imaging as a measure of the area at risk, Friedrich et al. used dark blood T2W imaging in a cohort of 92 patients presenting with their first MI to measure the salvaged myocardium after reperfusion. Imaging was obtained about 3 days after presentation. They found that the area of T2 was consistently greater than the area of LGE by about 16%. Although lacking a reference method, the investigators concluded that T2W imaging can be used to image the area at risk and calculate the salvaged myocardium in clinical practice and trials. Eitel et al. studied 208 patients with acute STEMI who underwent PCI and used CMR with T2W imaging and LGE to calculate myocardial salvage. They showed that patients with myocardial salvage index below the median had a higher MACE rate (22.1%) over 6 months follow-up than those with an index greater than or equal to the median (2.9%). In that study, myocardial salvage index was the strongest predictor of MACE, outperforming MVO and infarct size.
Despite a growing number of studies supporting the use of T2W imaging for area at risk and salvaged myocardium, some investigators have questioned the validity of the technique. The group of Kim et al. have listed a number of concerns, including inconsistencies in the pathophysiologic basis for T2W-derived area at risk, technical limitations, and the incongruent relationship of the T2 area at risk and infarct region. Based on these concerns, Kim et al. recently conducted a validation study in canines to compare the area of increased T2 signal with fluorescence microsphere area at risk, TTC, and LGE infarct territory. They imaged 21 canines with variable ischemic injuries and 24 patients about 3 days after ischemia using dark-blood and bright-blood T2W pulse sequences. Their analysis was based on quantitative measurement of the mean transmural extent of the abnormality and picture matching of contours. They found that the area of increased T2 signal correlated better with LGE and TTC regions than with histopathologic area at risk. Thus they concluded that T2W imaging does not depict the area at risk and should not be used to measure myocardial salvage.
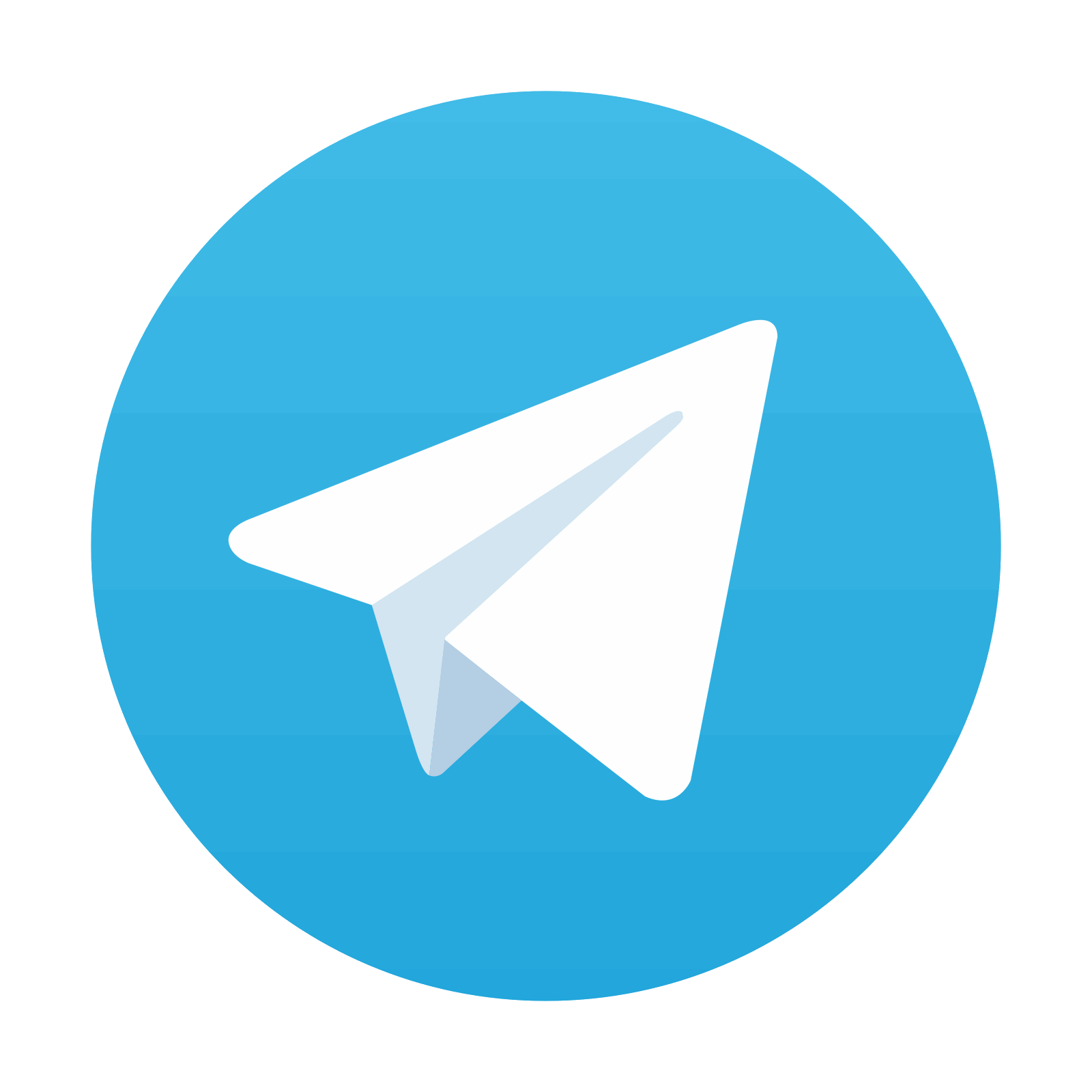
Stay updated, free articles. Join our Telegram channel

Full access? Get Clinical Tree
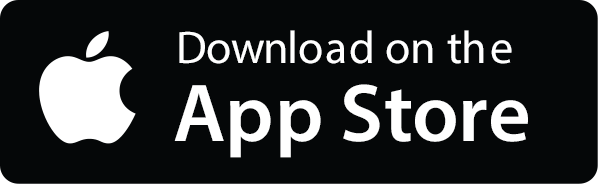
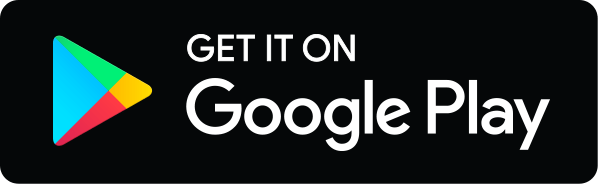