Fig. 1
Conventional lateral thoracic spine radiographs demonstrating vertebral fractures. a Osteoporotic fractures at the mid thoracic spine with slight anterior wedging of the vertebral bodies (arrows). b Mild anterior wedging of the T11 and T12 vertebral bodies with convex posterior vertebral border suggestive of malignant disease (arrows). c Significant loss of height of the T10 vertebral body and wedge deformity of approximately 50 % of the T6 vertebral body, with associated pedicle involvement (arrows). These features are in keeping with malignant compression fractures. d Patient with a history of prostate cancer. The lateral radiograph shows biconcave shape of the T12 vertebral body (arrow). Moreover, there is diffuse sclerosis noted at this level and the adjacent vertebral bodies, findings consistent with metastatic disease. e Same patient as in d. Sagittal CT reformat demonstrating the extent of sclerotic change
2.2 Findings Suggestive of Malignant Vertebral Fracture
Location of the fracture above the T7 level, presence of an epidural or paravertebral mass, osseous destruction and involvement of the posterior part of the vertebral body are most frequently features of malignant aetiology. In particular, pedicle involvement has been described as specific for malignant lesions (Baur-Melnyk and Reiser 2004). In addition, a convex posterior vertebral border suggests malignant disease (Fig. 1b–e).
2.3 Vertebral Collapse Associated with Specific Diseases
Vertebral fractures due to osteomalacia in adults (Fig. 2) are demonstrated as a biconcave deformity of the endplates (“cod fish” vertebrae) and are similar to those encountered in osteoporosis (Resnick 1982). Other characteristic radiographic findings in association with benign vertebral collapse include the “H” deformity in sickle cell anaemia and Gaucher’s disease. This type of deformity is visualized as a step-like depression of the superior and inferior vertebral margins and can also be found in thalassemia and congenital hereditary spherocytosis. In Scheuermann’s disease Schmorl’s nodes are seen. These are localised depressions of the endplate as a result of invagination of disc material into the subchondral vertebral body. Lastly, infection is a cause of endplate erosion and loss of disc height. In these cases MRI is used for the diagnosis, since it has been shown to have good to excellent sensitivity. Nonetheless, it should be pointed out that pathologic fractures can complicate infectious spondylitis (Ledermann et al. 2003).
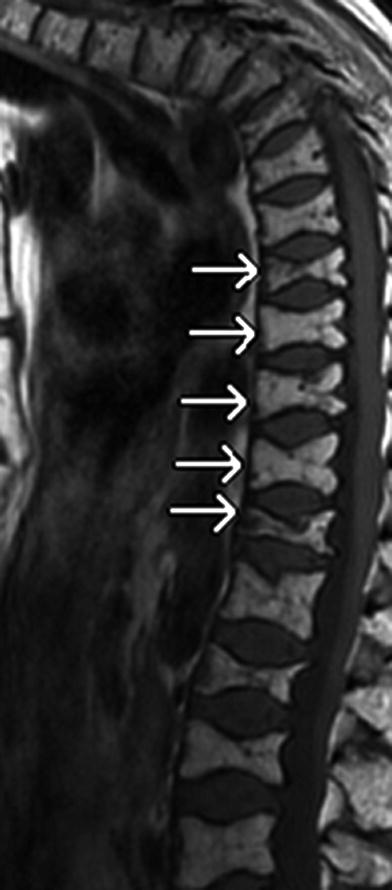
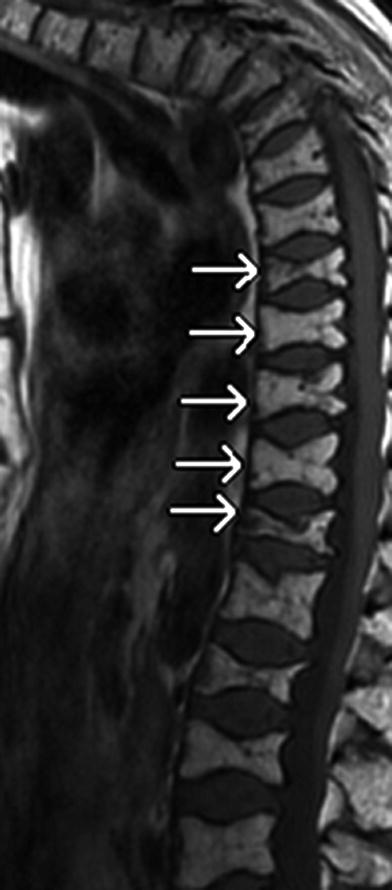
Fig. 2
Sagittal T1-weighted image shows multiple thoracic vertebral fractures due to osteomalacia (arrows) in a 68-year-old female patient. Biconcave deformity of the endplates (“cod fish” vertebrae) and kyphotic deformity are visualised
3 Computed Tomography
The use of multislice spiral CT has significantly improved the image quality and spatial resolution. CT is less sensitive than MRI in depicting abnormalities of the bone marrow. Nonetheless, CT may provide more detailed information than MRI on the margins of a lesion and matrix alterations, which is vital for the differentiation between benign and malignant vertebral fractures (Yuzawa et al. 2005). In addition, CT is more sensitive in demonstrating the vacuum phenomenon, which is a sign of benign disease. Lastly, CT is superior in visualising the bony structures and in demonstrating the fracture lines (Laredo et al. 1995; Stäbler et al. 1999).
3.1 CT Findings Suggestive of Benign Vertebral Fracture
The intravertebral vacuum phenomenon, which is commonly visualised following vertebral fractures, is associated with osteoporotic collapse and increase in frequency with age (Stäbler et al. 1999). Furthermore, benign vertebral compression fractures usually show retropulsion of a posterior bone fragment into the spinal canal or intravertebral fluid (Fig. 3a–c).
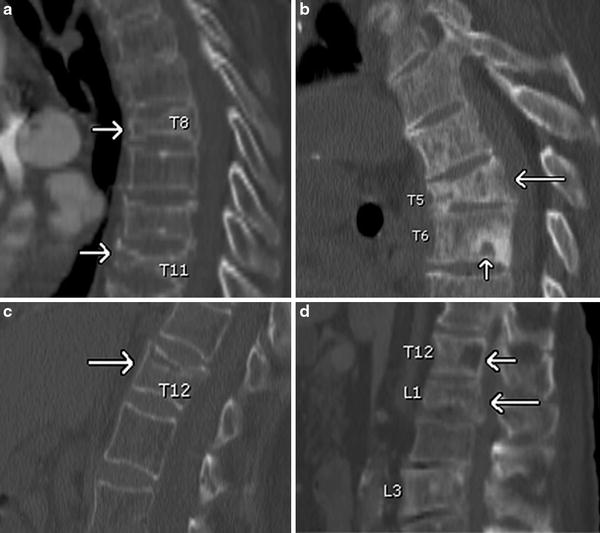
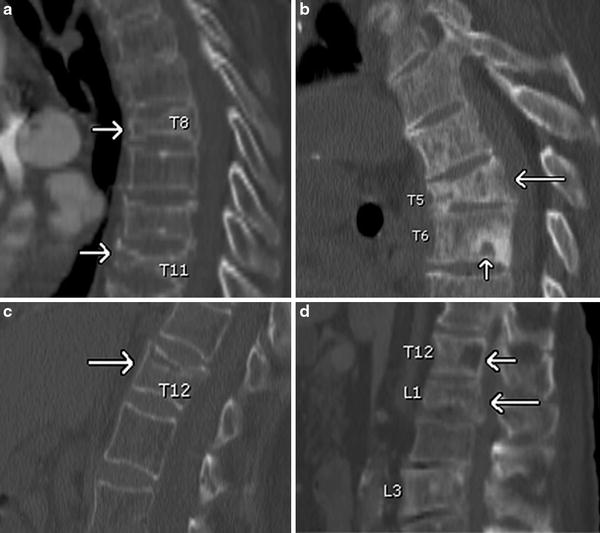
Fig. 3
Sagittal CT reformats. a Osteoporotic fractures of the T8 and T11 vertebral bodies (arrows) with anterior wedging, which is more pronounced at the T11 level. b Anterior wedging of the T5 vertebral body (long arrow) with retropulsion of its posterior inferior part, in keeping with an osteoporotic fracture. Also, Schmorl’s node is noticed at the inferior endplate of the T6 vertebra (short arrow). c Benign fracture of the T12 vertebral body (arrow) with slight retropulsion of its posterior superior part. d Loss of height of the L1 vertebral body with associated convex posterior cortex (long arrow), suggestive of malignant cause. Also, there is a lytic lesion noted at the posterior part of the T12 vertebral body (short arrow)
3.2 CT Findings Suggestive of Malignant Vertebral Fracture
In malignant vertebral fractures, the vertebral bodies have a convex posterior cortex and associated paravertebral or epidural masses might be found (Fig. 3d). Another highly specific finding is the posterior element infiltration, in particular that of the pedicle.
4 Magnetic Resonance Imaging
Although several recent studies have applied new MRI techniques in assessing vertebral collapse, on conventional spin echo MRI benign vertebral fractures can usually be distinguished from malignant ones (Baker et al. 1990). The reported sensitivity, specificity and accuracy of MRI for the diagnosis of metastatic compression fractures is 100, 93, and 95 %, respectively (Shih et al. 1999). In elderly patients, because of the almost complete replacement of the bone marrow by fatty tissue, fat suppression is thought to be necessary for the evaluation of the diseased vertebrae.
4.1 MRI Findings Suggestive of Benign Vertebral Fracture
4.1.1 Patterns of Signal Intensity and Enhancement
The signal intensity patterns of benign osteoporotic compression fractures are usually variable. In the majority of cases, the signal-intensity changes are localised (Fig. 4a) and normal bone marrow is visualised in at least one area within the vertebral body (Sugimura et al. 1987). Indeed, most vertebral metastases do not result in compression fractures until the entire body is infiltrated by the tumour (Fig. 4b), causing destruction of the trabeculae, the cortex or both (Yuh et al. 1989). However, it has been reported that in 15 % of acute osteoporotic compression fractures (Fig. 4c) there is complete replacement with abnormal signal intensity (Shih et al. 1999). This might be due to spread to the entire vertebra of a reactive process induced by the fracture. Therefore, in acute osteoporotic fractures, when the height of the vertebral body is preserved and diffused signal-intensity changes are seen on T1-weighted, STIR, and contrast-enhanced T1-weighted images, the diagnosis may be very difficult, since these imaging features mimic those encountered in malignant compression fractures. In chronic osteoporotic fractures there is no abnormal signal intensity identified in the bone marrow of the compressed vertebra (Tan et al. 2002).
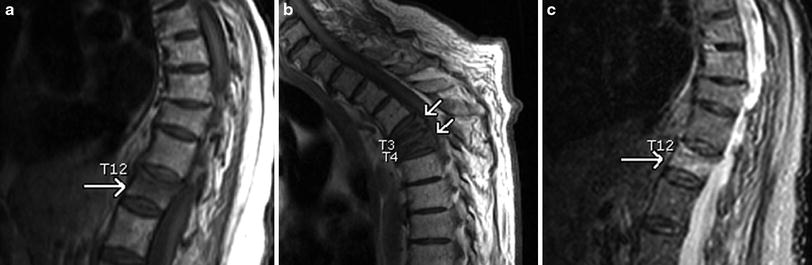
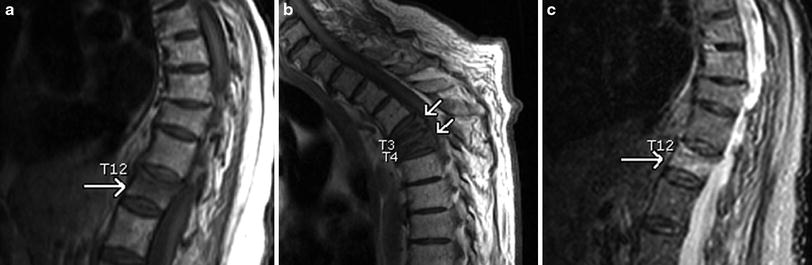
Fig. 4
a Sagittal T1-weighted image showing biconcave morphology and diffuse low signal-intensity within the T12 vertebral body. However, at least one area of normal bone marrow is detected (arrow). Also, retropulsion of the posterior superior part of the vertebra is seen. These features are in keeping with benign aetiology. b Sagittal T1-weighted image demonstrating anterior wedging of the T3 and T4 vertebral bodies, with complete replacement of the normal bone marrow by low signal intensity. Also, there is slight convexity of the posterior part of the vertebrae (arrows) noticed. These findings are in keeping with malignant disease. c Same patient as in a. STIR sagittal image. Almost complete and diffuse replacement of the bone marrow with high signal intensity (arrow) is detected, representing bone marrow oedema
4.1.2 Fluid Sign
A cleft of fluid (demonstrated as a linear area of high signal-intensity) or gas within the vertebral body on T2-weighted and STIR images (Fig. 5a, b), have been described in association with osteoporotic fractures (Baur et al. 2002). These are considered to represent avascular necrosis or pseudoarthrosis. In particular, the “fluid sign” is seen adjacent to the fractured endplates and the signal is isointense to that of cerebrospinal fluid (Oka et al. 2005).
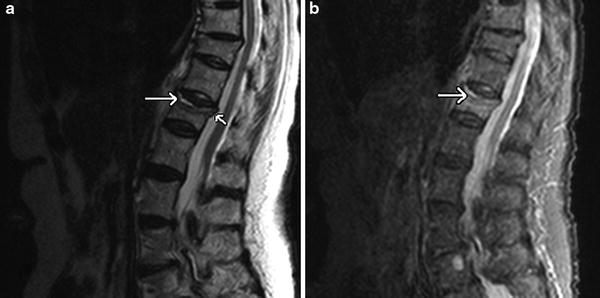
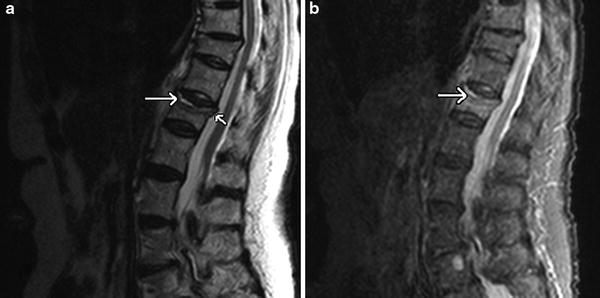
Fig. 5
Fluid sign in osteoporotic fractures. a Linear area of high signal intensity is seen at the anterior endplate (long arrow) on this sagittal T2-weighted image. There is some retropulsion of the posterior superior part of the vertebral body within the spinal canal also noticed at this level (short arrow). b Sagittal STIR sequence demonstrating the “fluid sign”
4.1.3 Low Signal Intensity Band
In acute benign compression fractures, a band like low signal intensity area on T1-weighted and T2-weighted images is commonly seen (Fig. 6a, b). This area is sited adjacent to the collapsed endplate and is encountered in 93 % of acute osteoporotic fractures as opposed to 44 % of metastatic ones (Jung et al. 2003).
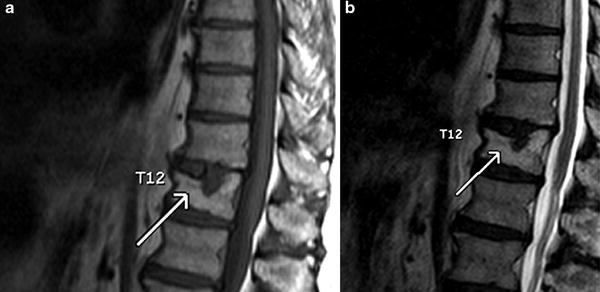
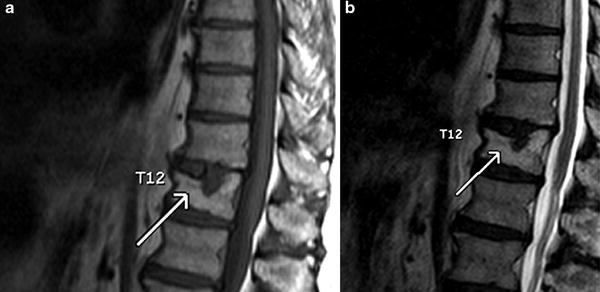
Fig. 6
Low signal intensity band in benign vertebral compression fractures. a Sagittal T1-weighted MR image showing a band-like low signal intensity area adjacent to the collapsed endplate of the T12 vertebral body (arrow). b Sagittal T2-weighted image. There is a subtle low signal intensity band demonstrated at the level of the collapsed superior endplate (arrow)
4.1.4 Retropulsion of a Posterior Bone Fragment
Retropulsion of a posterior bone fragment is believed to be sensitive and specific for osteoporotic fractures. It is detected in 60 % of acute osteoporotic fractures as opposed to 11 % of metastatic collapses (Fig. 7). In cases of metastatic disease, retropulsion might be due to preexisting compression fractures that were secondarily involved by metastatic disease. It is also possible that metastatic disease can sometimes be associated with retropulsion of bone fragments (Kaplan et al. 1987).
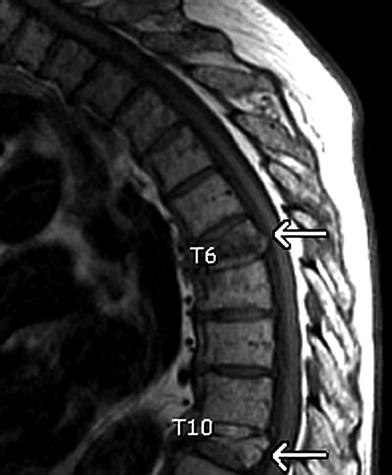
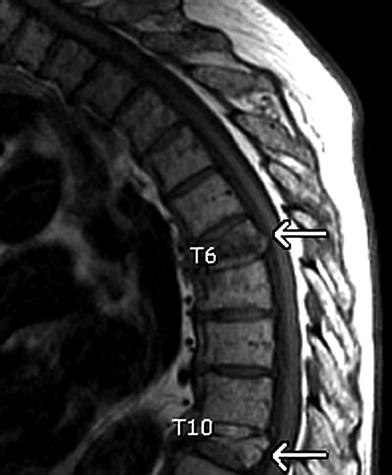
Fig. 7
Osteoporotic vertebral collapse. Retropulsion of a posterior bone fragment is noted at the level of the T6 and T10 vertebral bodies on this sagittal T1-weighted image (arrows)
4.1.5 Pedicle Involvement
Pedicle involvement has been reported as specific for malignancy (Fig. 8a–d). However, it can be found in many patients with osteoporotic fractures, particularly in the early phase. It has been reported that pedicle abnormal signal intensity and contrast enhancement are seen in 64 % of benign compression fractures and in 84.2 % of malignant ones, with no significant difference between groups (Ishiyama et al. 2010). In general, the criteria of pedicle infiltration by tumour include the presence of inhomogeneous diffuse signal-intensity changes or a mass effect.
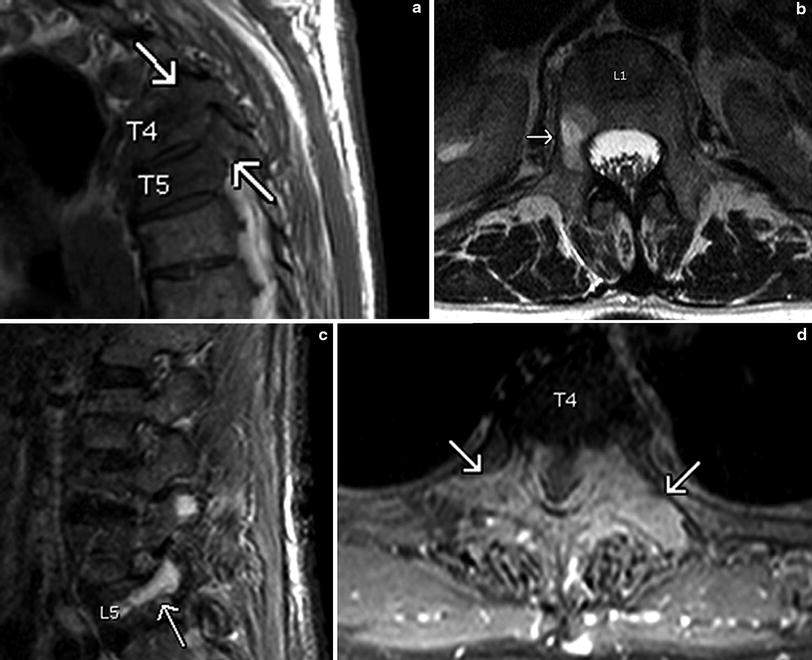
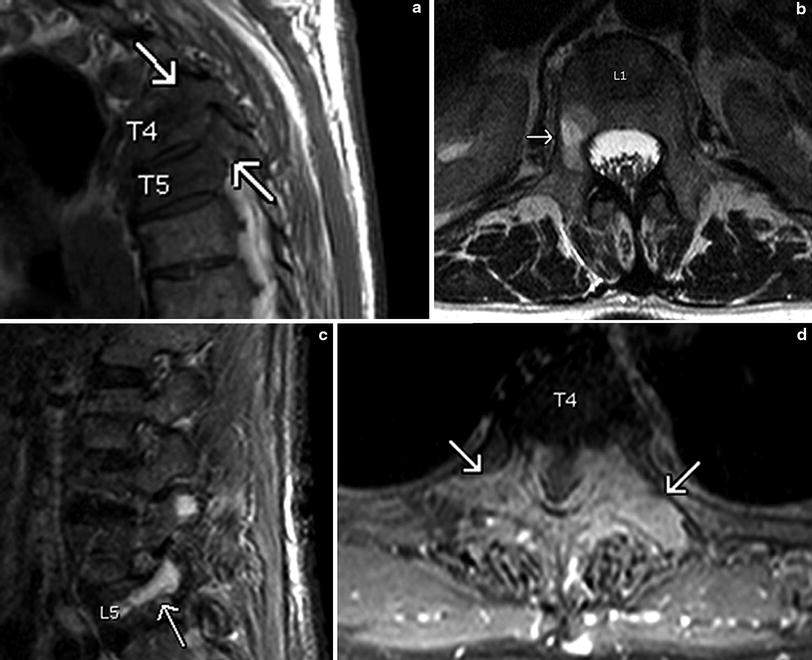
Fig. 8
Pedicle involvement in malignant vertebral compression fractures. a Sagittal T1-weighted image showing diffuse abnormal low signal intensity within the pedicles and the vertebral bodies at the T4 and T5 levels (arrows). b Axial T2-weighted image demonstrating abnormal high signal intensity within the pedicle of the L1 vertebra on the right hand side (arrow). c Sagittal STIR image. Pathologic high signal intensity is noted within the pedicle of the L5 vertebra. d Contrast-enhanced axial T1-weighted image with fat suppression. There is enhancement of the pedicles and the transverse processes (arrows) of the T4 vertebra seen. Enhancement of the spinous process is noticed as well
Nonetheless, it should be stressed that signal-intensity changes in osteoporotic fractures are often focal and inhomogeneous as well. When pedicle involvement is the only sign, a diagnosis of malignant pathologic fracture should not be assumed. Sclerosis of the pedicle is seen during the healing process of microfractures and is considered to represent reactive change. This reflects the signal intensity patterns noted on MRI, which represent infiltration by inflammatory cells, granulation tissue or fibrosis.
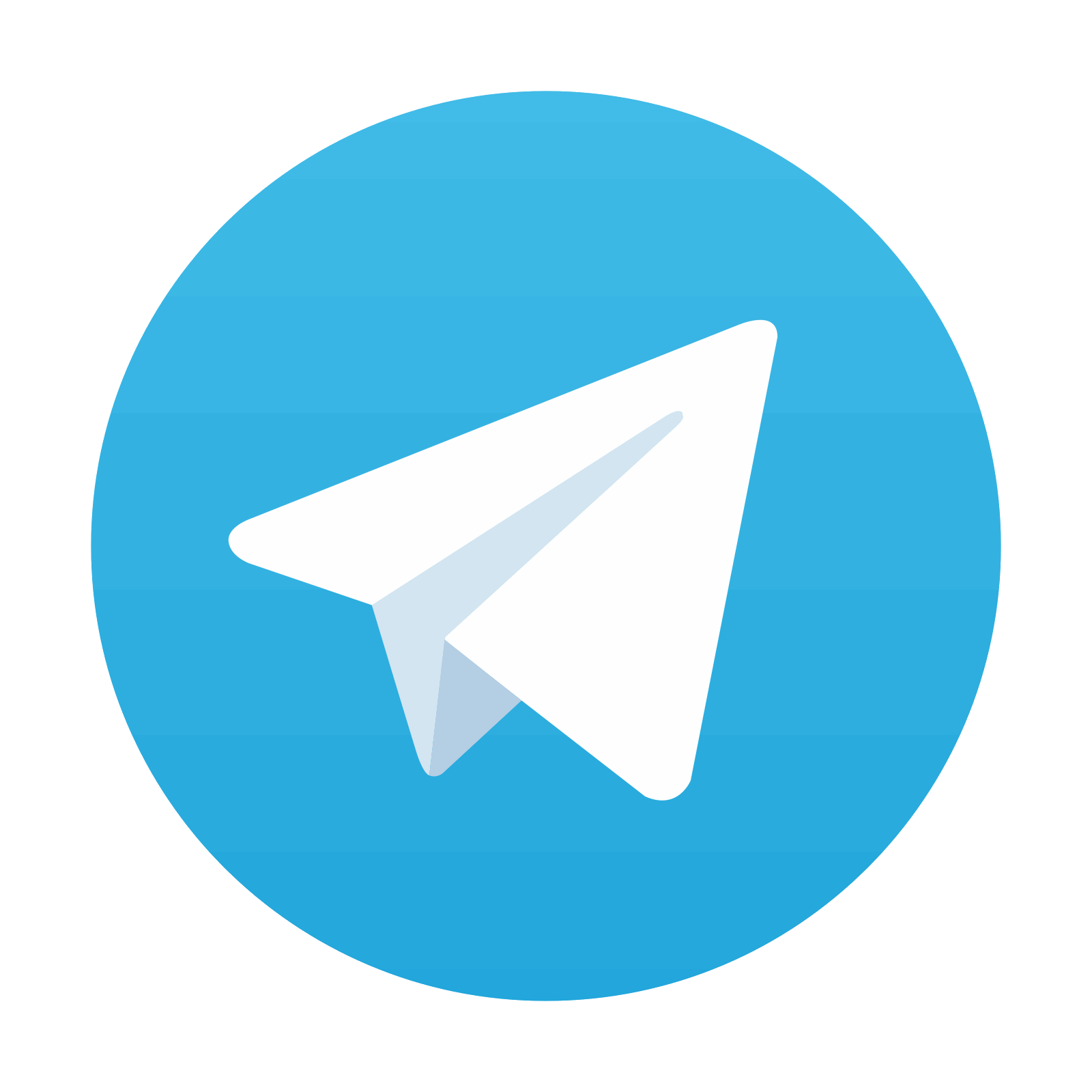
Stay updated, free articles. Join our Telegram channel

Full access? Get Clinical Tree
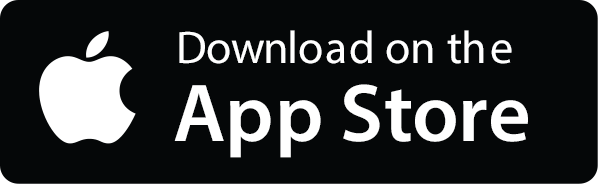
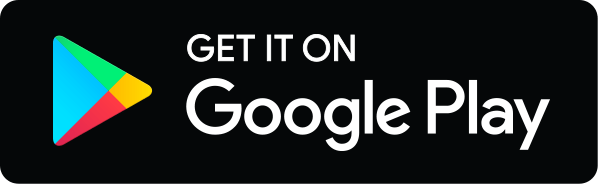