Magnetic resonance (MR) imaging has new applications in the assessment of temporal bone disorders. This article summarizes current MR imaging applications in evaluating adult temporal bone lesions according to their location, beginning from the most common indication, vestibular schwannoma. Inner ear lesions, petrous lesions, and middle ear lesions are discussed, including the role of diffusionweighted imaging in cholesteatomas, external ear lesions, and a few systemic conditions. Although this article emphasizes the role of MR imaging, the diagnostic value of computed tomography scan associated with MR imaging is also stressed. The main indications of temporal bone MR imaging are summarized.
- •
Magnetic resonance imaging is the primary imaging modality for evaluation of the nonosseous components of the adult temporal bone.
- •
Both temporal bones are always imaged to compare sides and the brain/skull base included so that intracranial extension can be evaluated.
- •
Middle ear congenital cholesteatoma presents as a nodular soft tissue mass, without osseous erosions or involvement of Prussak space, and with normal pars flaccida.
- •
A non–echo planar (EP) diffusion-weighted (DW) imaging sequence should be preferred rather than an EP DW imaging sequence because it is less sensitive to susceptibility artifacts and is therefore able to show cholesteatomas as small as 2 mm.
- •
In early stages of necrotizing external otitis, nonspecific soft tissue swelling is present in the external auditory canal with erosion at the petrotympanic fissure and obliteration of the retrocondylar fat pad.
Introduction
Temporal bone imaging is challenging because the normal anatomy includes many small but clinically important structures, and a significant abnormality in this area may be less than 1 mm in size. With isotropic sections, bone algorithm, and three-dimensional (3D) reconstructions, computed tomography (CT) has been the mainstay for evaluating the temporal bone since its inception in the early 1970s, whereas magnetic resonance (MR) imaging is the primary imaging modality for evaluation of the nonosseous components including major blood vessels, fluid spaces (cerebrospinal fluid [CSF], endolymph, perilymph), nerves, muscle, cartilage, brain, salivary glands, and fat. MR imaging has superior soft tissue resolution, multiplanar capability, does not use ionizing radiation, and is the most effective technique for the evaluation of these structures and their disorders. Both of these techniques are complimentary, and it often becomes necessary to use both CT and MR imaging for satisfactory tissue characterization and identification of disorders or confident exclusion of abnormalities. This article reviews current clinical applications of MR imaging techniques appropriate for diagnosing adult temporal bone disorders, and presents appropriate examples.
Technique
MR imaging of the temporal bone is typically performed using a dedicated multichannel head coil and a high field strength MR imaging system. The signal/noise ratio can be improved approximately 3 to 5 times compared with that of a routine head coil by using dedicated phased-array surface coils specifically designed for temporal bone imaging. The disadvantage of using these coils is that the images are not homogeneous in signal intensity, the coils are more cumbersome for the technologist to position, and are not routinely used at the authors’ institutions. Both temporal bones are always imaged to compare sides and the brain/skull base included, so that intracranial extension of a disease process can be evaluated. To address these specific goals, 4 basic MR imaging techniques are used: whole-brain imaging, high-resolution T2/fluid space imaging, precontrast-enhanced and postcontrast-enhanced high-resolution T1-weighted imaging, and vascular (MR angiography) imaging.
Routine Brain Survey
An MR imaging protocol of the temporal bone should start with a brain examination using a T2-weighted, or fluid-attenuated inversion recovery (FLAIR) and whole-brain postgadolinium T1-weighted sequence to exclude associated brain pathologic processes. For example, a patient who presents with symptoms of dizziness could have disorders that result from brainstem demyelination, ischemia, or a neoplasm. Diffusion-weighted (DW) imaging should be added, which helps in differentiation between congenital or acquired cholesteatoma and epidermoid cysts.
High-Resolution T2-Weighted Imaging
Fluid-sensitive high-resolution images are noncontrast enhanced and show the CSF and endolymphatic spaces as regions of high signal intensity. The cisternal cranial nerves can be visualized without contrast using this technique because the nerves are surrounded by fluid of higher signal intensity. Fluid in the otic capsule structures is best visualized using this technique, making it possible to evaluate cochlear or vestibular disorders. This technique has also been used as for screening to rule out vestibular schwannomas.
A heavily T2-weighted sequence, usually a submillimetric 3D turbo spin echo (TSE)/fast spin echo (FSE) T2-weighted sequence, is performed to evaluate the fluid content and signal intensity characteristics in the membranous labyrinth. Postprocessing of the image data can create any specific projection or surface desired. Steady-state T2-weighted gradient echo images using constructive interference techniques also have excellent quality and are not marred by increased magnetic susceptibility artifacts because of their short echo times.
High-Resolution Precontrast-Enhanced and Postcontrast-Enhanced T1-Weighted Images
T1-weighted sequences are performed before and after intravenous administration of gadolinium: a 1-mm (or even submillimeter) axial 3D T1-weighted gradient echo sequence or a thin-slice (2 mm or less) axial and coronal spin echo (SE) T1-weighted sequence can be used. Fat saturation techniques can be applied in 1 direction after contrast administration. This sequence is ideal for identification of subtle changes, like those seen with labyrinthitis/vestibulitis or small vestibular tumors.
T1-weighted gradient echo images can be acquired by using a moderate recovery time (30–50 milliseconds) and flip angle (30°–50°) and by spoiling (destroying residual transverse magnetization). Spoiling is needed to eliminate T2 contrast components from the acquired signal, thus yielding T1-weighted images similar to routine spin echo images but can also show the vessels to advantage. Caution must be exercised, because high signal of vessels may be mistaken for enhancement in a tumor. Routine spin echo T1-weighted images can be used, but the resolution is significantly lower. The lack of any high signal within the canal on this sequence is a reliable indicator that no eighth nerve tumor is present. Some radiologists add fat suppression to eliminate potentially confusing high signal from the fat in the petrous apex.
With the increasing use of 3-T magnets, the axial submillimeter 3D T1-weighted gradient echo sequence is likely to become the sequence of choice because it gives the maximum number of slices through the temporal bone.
Acquisition of a noncontrast T1-weighted sequence is of value to help characterize high-signal regions on contrast studies because, without a noncontrast examination, it may be difficult to differentiate fat or subacute hemorrhage.
MR Angiography
In addition, a dedicated MR angiography acquisition may be of value in specific instances, especially in a case of suspected glomus tumor or in the evaluation of a patient with pulsatile tinnitus. Most commonly, noncontrast 3D time-of-flight sequences are used, which generate the best detail. Postprocessing of these volume data sets can be used to create detailed 3D projections in different planes and perspectives. Flow compensation decreases signal from the moving CSF and thereby increases the contrast/noise ratio between the blood vessels and the CSF in MR angiography; therefore, the soft tissue detail may be poor. Although current MR angiography can provide high-quality images, they cannot substitute for traditional catheter angiography in many cases, which remains the gold standard to exclude small dural arteriovenous fistulas.
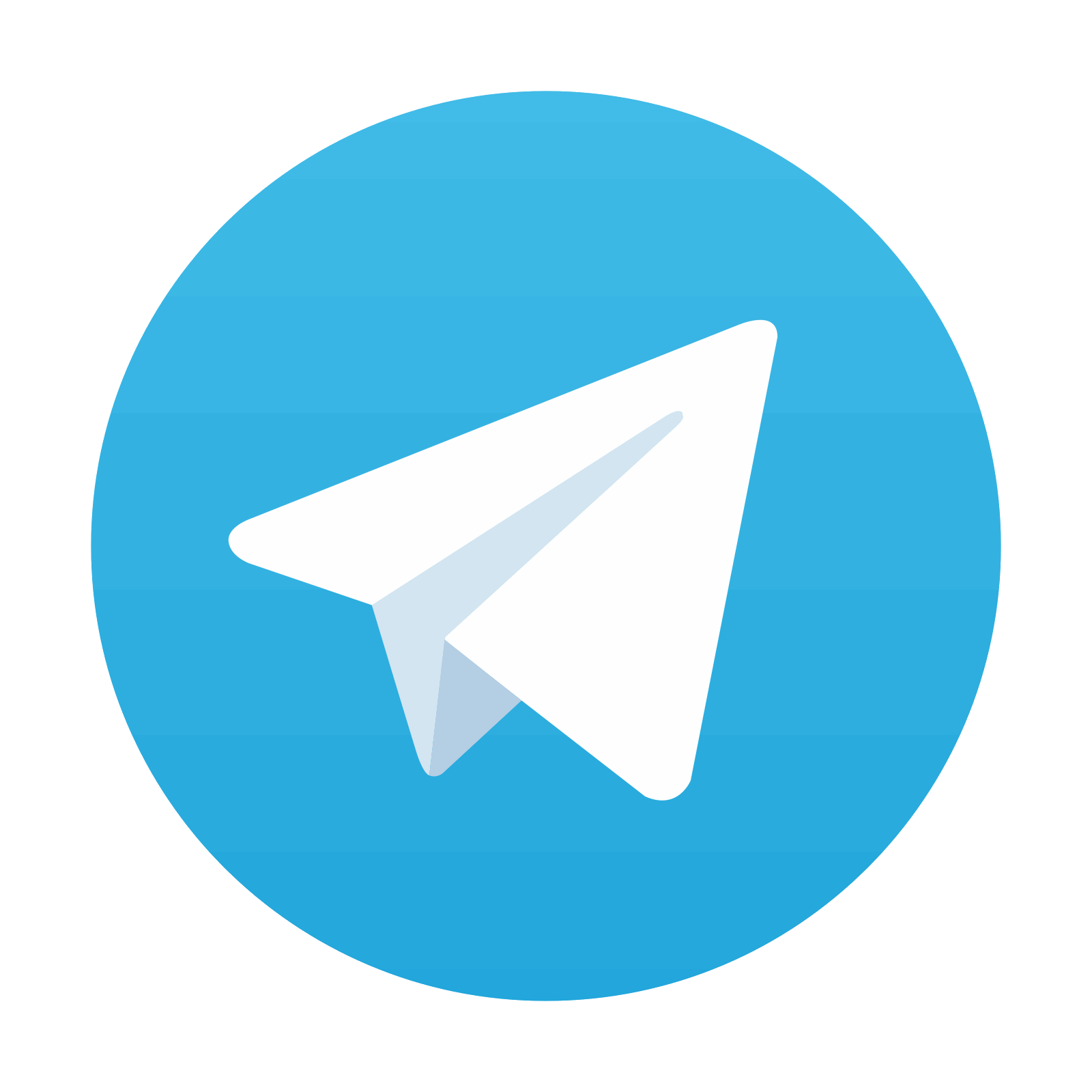
Stay updated, free articles. Join our Telegram channel

Full access? Get Clinical Tree
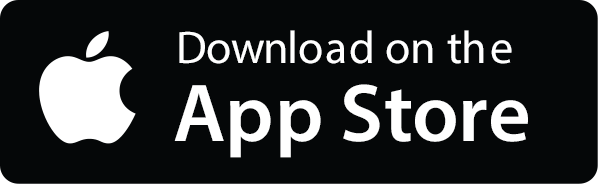
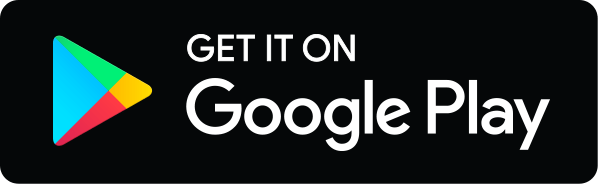