Fig. 40.1
Interactions between bone and cancer cells in paradigmatic examples of osteolytic (breast cancer) and osteoblastic (prostate cancer) bone metastases. In both examples bone metabolism with resorption and formation occurs. The depicted mediators emphasize the predominant pathways.
In most tumors, both serum concentrations of makers of bone formation (p.e. alkaline phosphatase) and resorption (p.e. N-terminal telopeptide) are increased, which reflects bone turnover activation. A trend towards increased bone formation or resorption may explain the clinical presentation as osteoblastic (predominantly bone-forming) or osteolytic lesions (predominantly bone-degrading), respectively [14]. In osteoblastic lesions, as in prostate cancer, new mediators come into play inclining the balance towards bone formation [15]. Prostate cancer cells secrete mainly Wnt family ligands, bone morphogenetic proteins, platelet-derived growth factor (PDGF) and endothelin-1, which are potent osteoblastic activators (Fig. 40.1) [12, 15].
40.3 Clinical Features of Metastatic Bone Disease
40.3.1 Clinical Findings
Metastatic bone disease affects more commonly the axial skeleton (pelvis, spine and ribs) and femurs.
Approximately one third of the bone lesions are asymptomatic [16]. When symptoms are present, pain is the most common (50 %) [17]. In fact, bone metastases are the most common cause of cancer related pain (28–45 %) [18]. Pain due to metastatic bone disease is often dull, persistent, typically worsens at night and does not improve with rest. Pain affecting the axial skeleton tends to be diffuse, while pain affecting the extremities is more localized (similar to the pain secondary to pathological fractures). As the disease progresses, weight-bearing activities intensify pain. Hence, pain which increases with physical activity can be a marker of impending fracture [19].
In addition to pain, bone fracture, skeletal instability, spinal cord compression, hypercalcemia of malignancy and the need for surgery/radiotherapy for the management of symptomatic bone metastases , frequently referred as SREs, are also a common manifestation of metastatic bone disease, more often in patients with lytic disease [6].
In the pre-osteoclastic inhibition era, the highest incidence of SREs was seen in breast cancer (an incidence of 68 % at 24 months), half of which pathologic fractures, an event closely followed from the need of radiation to bone [20]. Among other solid tumors prostate cancer (49 % at 24 months) would follow [20, 21]. With the introduction of bisphosphonates, the risk of developing a first SRE was reduced by 15 % and the risk of subsequent SREs was reduced by 28 %, though still represent an important source of morbidity associated with metastatic bone disease (MBD) [22]. More recently, an extra relative 17 % decrease in the risk of first SRE and 18 % in the risk of subsequent SRE was achieved with denosumab [23].
40.3.2 Laboratory Findings
Alkaline phosphatase (ALP) and N-terminal cross-linked telopeptide of type I collagen (NTX) are commonly elevated in patients with bone metastases . Bone ALP isoenzyme corresponds to approximately 40 % of the total ALP. This isoenzyme is a marker of osteoclasts activity and therefore of bone formation [24]. NTX is a by-product of osteoclast degradation of type I collagen and hence a marker of bone resorption [25]. There are studies that suggest that the elevation of NTX provides relevant prognostic information. Patients with moderate and high NTX levels (50–99 and ≥100 nmol/mmol creatinine, respectively) have a twofold increase in their risk of SRE and bone disease progression compared with patients with low NTX levels. Moreover, high levels and moderate levels of NTX are associated with a four to sixfold and two to fourfold increased risk of death, respectively.
Although informative, neither of these markers markers are currently approved for the diagnosis or as clinical tools to support decision making in clinical practice [24].
Several other biochemical markers of bone metabolism are available, namely C-telopeptide of type I collagen (CTX), pyridinoline cross-linked carboxyterminal telopeptide of type I collagen (ICTP), osteocalcin (OC), osteoprotegerin (OPG), C-terminal propeptide of procollagen type I (PICP), N-terminal propeptide of procollagen type I (PINP) and tartrate-resistant acid phosphatase 5b (TRAcP-5b) [24]. Less data is available on their clinical significance.
40.3.3 Radiologic Assessment
The radiologic assessment of MBD involves different imaging options, which provide complementary information. These include plain radiographs (XR), bone scintigraphy (BS), computerized tomography (CT), magnetic resonance imaging (MRI) and positron emission tomography (PET) scan. Usually, when metastatic bone disease is suspected, BS and XR are the first exams to be requested [26].
The relative activity predominance of osteoblasts or osteoclasts leads to two historical clinical patterns of bone metastases : lytic (characterized by bone degradation) and blastic (characterized by bone formation). In some patients the clinical pattern is mixed (up to 15 % in prostate and lung cancer and up to 30 % in breast cancer) [27, 28]. On the XR, lytic lesions are hypodense and blastic lesions hyperdense. XR is widely available and is relatively inexpensive. However, 30–75 % of normal bone mineralization must be degraded before osteolytic findings in the lumbar vertebrae become apparent on XR, delaying the diagnosis of metastatic lesions for several months [26].
BS screens the complete skeleton for bone metastases . A positive scan is characterized by an uneven distribution of the administered radiotracer (increased or decreased concentration). When compared to the surrounding areas, sections with an increased radiotracer concentration are darker and referred as “hot spots”, while those with decreased concentration are referred as “cold spots”. BS is more sensitive than XR for the diagnosis of metastatic bone disease (62–100 % vs. 44–50 %) [26]. In fact, a 10 % change in bone mineral turnover is enough to be identified by BS. However, BS has lower specificity and therefore an higher false-positive rate [26]. BS findings reflect the osteoblastic activity and skeletal vascularity (not the tumor cells themselves), therefore other bone insults, such as trauma or inflammation, can lead to false positive results. On the other hand, rapidly growing pure osteolytic metastases, when bone turnover is slow, or when the site is avascular can lead to false-negative results [26].
In clinical practice, XR and BS are complementary methods, with XR helping to clarify nonspecific or atypical findings [26].
CT scans and MRI are generally used to better characterize bone disease. CT scan is very sensitive when detecting small cortical erosions and fractures. The reported sensitivity ranges from 71 % to 100 % [26]. CT is more sensitive than BS and XR when detecting lesions in the spine and calvarium [26]. CT is the preferred method to assess cortical bone integrity. Bone MRI has a reported sensitivity of 82–100 % and specificity from 73 % to 100 % for the diagnosis of bone metastases . The resolution power to detect bone marrow disease is higher when compared to CT, however the ability to detect cortical bone destruction is inferior, given that cortical bone is not detectable by MRI. MRI is commonly used to assess pathologic fractures from hip and pelvis. Furthermore, it also allows for an accurate assessment of the bone marrow and the diagnosis of any associated cord compression [29].
Finally, the emergence of PET scan, and particularly of the combination of PET scan with CT (PET/CT) added a new tool to evaluate bone disease. Nevertheless, PET without the CT component is not an ideal method for the diagnosis of osteoblastic lesions [30]. While for most tumors 18F-fluorodeoxyglucose is the radioactive tracer of choice for prostate cancer 11C-choline was more recently established as the preferred radioactive tracer [31].
A meta-analysis [32] combining studies from 1995 to 2010 compared PET (without CT), CT, MRI and BS as the method of diagnosis of bone metastases in several cancer types. The reported sensitivity estimates on per-patient basis for PET, CT, MRI and BS were 89.7 %, 72.9 %, 90.6 % and 86.0 %, respectively, while the specificity estimates for PET, CT, MRI and BS were 96.8 %, 94.8 %, 95.4 % and 81.4 %, respectively. A recent expert consensus statement discussed exhaustively the role of imaging by cancer histologic type and metastases anatomic localization, with an emphasis on the use MRI and PET-PET/CT [33].
Figure 40.2 represents an algorithm for radiologic evaluation of patients with clinical suspicion of bone metastases as proposed by Hamaoka T. et al.
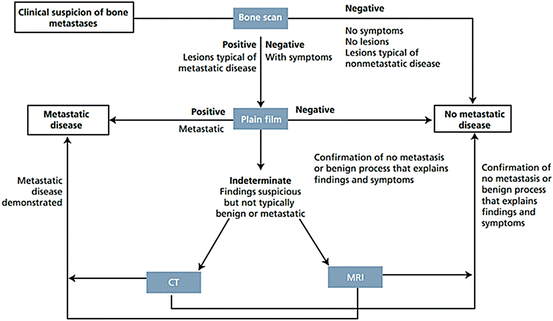
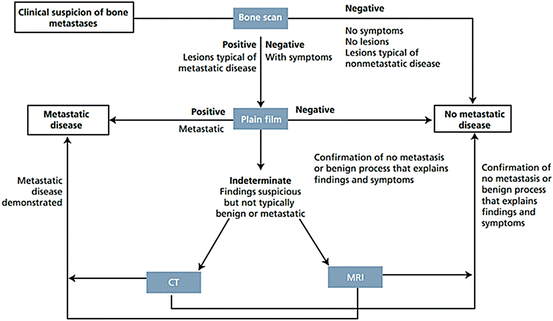
Fig. 40.2
Algorithm for imagiological evaluation of patients with clinical suspicion of bone metastases
40.3.4 Pathological Assessment
A bone lesion biopsy is the standard method to establish the diagnosis of metastatic bone cancer. It allows: (1) the diagnosis of suspected bone lesions without known primary tumor or of symptomatic or radiographically equivocal bone lesions; (2) the differential diagnosis of suspected primary bone tumor s ; (3) the re-examination of biologic features of the tumor to drive treatment choices (e.g. in breast cancer, it allows the re-examination of receptor status).
Nevertheless, a bone biopsy is an invasive procedure and therefore the risk-benefit ratio should be assessed. As an anecdote, in a patient with already confirmed metastatic hormone receptor positive breast cancer the presence of radiological suspicious lesions probably does not require biopsy confirmation of metastatic bone disease.
40.3.5 Longitudinal Assessment of Bone Disease
The longitudinal assessment of bone disease is challenging. The Response Evaluation Criteria in Solid Tumors (RECIST), the most commonly used tumor response criteria for solid tumors, considers most bone lesions (those without soft tissue masses ≥10 mm) as “non-measurable”, which shows how difficult it is to evaluate these lesions [34, 35]. To overcome RECIST limitations, bone-specific (MD Anderson [MDA]) and metabolic-specific (Positron Emission Tomography Response Criteria in Solid Tumors [PERCIST]) response criteria were developed [35], however the uptake of these criteria has been minor. Therefore, the combination of clinical symptoms (including the use of standardized self-reported bone pain scores and quality of life scores to judge symptomatic response to therapy), laboratory findings and imaging data is what usually guides therapy options [36].
40.4 Treatment of Bone Metastases
The treatment goals of metastatic bone disease are symptomatic control, quality of life and survival extension.
40.4.1 Medical Management
The medical management of metastatic bone disease has evolved over the last decade to include therapies directed to the tumor and bone environment.
40.4.1.1 Tumor Directed Therapy
Tumor directed therapies (chemotherapy, hormonal therapy and biologics) are useful for the management of metastatic disease in tumors known to respond to these modalities. Tumor directed therapy should follow the appropriate metastatic treatment guidelines specific for each primary tumor. Cancer medullar involvement and chemotherapy can induce an additive hematologic toxicity.
40.4.1.2 Bone Directed Therapy/Bone Modifying Agents (BMA)
Bisphosphonates (BP) and denosumab are the two classes of drugs approved in this setting.
Type of BMAs Available, Administration and Efficacy
Bisphosphonates are incorporated in the bone matrix and absorbed by osteoclasts during bone remodeling. Inside osteoclasts, BPs inhibit the mevalonate pathway (through the blockage of farnesyl pyrophosphate synthase), which blocks the osteoclast activity and ultimately bone resorption. This leads to a decrease in bone remodeling and in the rate of skeletal related events.
BPs are a class of agents which includes alendronate, ibandronate, neridronate, pamidronate, risedronate and zolendronate. The two BPs most commonly used are pamidronate and zoledronate.
Zolendronate is used as 4 mg IV over 15 min every 3–4 weeks, while pamidronate is used as 90 mg IV over 2 h every month. These two formulations significantly reduce the rate of SREs in patients with bone metastases .
Pamidronate was compared to placebo and demonstrated superiority in terms of reduction in the skeletal morbidity rate (number of SREs divided for the number of patients in 1 year; 2.4 vs. 3.7, P < 0.001) and percentage of patients with SREs at 2 years (51 % vs. 64 %, P < 0.001), but also regarding the increase in the median time to SRE (12.7 vs 7 months, P < 0.001) [20].
The pivotal trial comparing zolendronate to pamidronate was a phase III study involving 1,648 patients with bone metastases from breast cancer and multiple myeloma [37]. After a median follow-up of 25 months, zolendronate and pamidronate had comparable safety profiles, but zolendronate reduced the overall risk of developing an SRE by an additional 16 % (P < 0.001). Favorable results for zolendronate were also obtained for patients with castration-resistant prostate cancer (36 % reduction of SREs risk when compared to placebo), lung (31 % reduction of SREs risk when compared to placebo) and renal cell (58 % reduction of SREs risk when compared to placebo) cancers [38, 39]. A weaker but clinical significant evidence of efficacy was also documented for other solid tumors, as thyroid and bladder cancer [38].
Oral formulations of BPs, as ibandronate (50 mg tablet daily) and clodronate (1,600–3,200 mg capsules daily), are also available. These formulations have a comparable safety profile and for some patients are viewed as having a more convenient mode of administration. For patients with bone metastases from breast cancer , ibandronate demonstrated a reduction in SRE when compared with placebo [40]. Nevertheless, oral ibandronate could not demonstrate non-inferiority in preventing SRE when compared to IV zolendronate [41]. For patients with metastatic prostate cancer, clodronate also demonstrated efficacy in reducing SRE when compared to placebo [40]. When compared to zolendronate, clodronate had a similar action in the reduction of the SRE rate [42]. However, the zolendronate group had an improved bone progression free survival (31 vs. 22 months, p = 0.04). No overall survival differences were found. Despite these limitation, oral formulations of BP can be discussed with the patient if a strong preference is present or if difficulties with intravenous administration of drugs occur.
Denosumab is a fully human monoclonal antibody with high affinity for RANKL. The interaction between denosumab and RANKL decreases the availability of RANKL, and therefore blocks its natural interaction with the osteoclast precursor surface receptor RANK, precluding osteoclast formation and bone resorption.
Denosumab (120 mg SubQ every 4 weeks) effectively reduces the rate of SREs in patients with bone metastases . Denosumab was compared to zolendronate in a phase III trial involving 2,046 patients with bone metastases from breast cancer [43]. Denosumab was superior in delaying time to first on-study SRE (HR 0.82; 95 % CI, 0.71–0.95; P = 0.01 for superiority) and time to first and subsequent (multiple) on-study SREs (rate ratio, 0.77; 95 % CI, 0.66–0.89; P = 0.001). A similar safety profile was documented. Denosumab has also demonstrated favorable results when compared to zolendronate in patients with castration-resistant prostate cancer (18 % reduction on time to first SRE; statistically significant for superiority) [44]. Of note, hypocalcemia was more frequent in prostate cancer patients (13 % vs. 6 % in the zoledronic acid group) [44]. For patients with other types of solid tumors and multiple myeloma denosumab was non-inferior to zolendronate [45].
A recent meta-analysis [46] concluded that denosumab is superior to zolendronate in the prevention of bone complications from bone metastases , namely in time to first on-study skeletal-related event (hazard ratio [HR] 0.83; 95 % CI, 0.76–0.90, P < 0.001), time to multiple skeletal-related events (HR 0.83; 95 % CI, 0.76–0.90, P < 0.001) and pain worsening (HR 0.92; 95 % CI, 0.86–0.99, P = 0.026). However, no effect on survival was found. Furthermore, the cost of denosumab is significantly higher than that of zoledronic acid, particularly when generic BPs are available in Europe and in the US.
Recent guidelines from the American Society of Clinical Oncology (ASCO) on the role of bone-modifying agents in metastatic breast cancer (one of the most widely studied type of patients in this setting) consider denosumab, zolendronate and pamidronate as equally valid options in the setting of bone metastization [47].
Side Effects
An uncommon but serious side effect from parenteral bone-modifying agents is osteonecrosis of the jaw (ONJ). ONJ is defined as the persistence of exposed bone in the oral cavity despite adequate treatment for 8 weeks, without local evidence of malignancy and no prior radiotherapy to the affected region [48]. Despite being a serious side effect it is relatively uncommon, occurring in up to 2 % of the patients receiving zolendronate or denosumab [49]. Prolonged therapy increases the risk of ONJ, with a documented median time to ONJ of 15 months for patients receiving either zolendronate or denosumab [50]. Identified risk factors for ONJ include invasive dental procedures (extractions or implants), trauma, poor dental hygiene, and therapy with antiangiogenic agents and probably corticosteroids. Every invasive dental procedure should be done several months before treatment with bone modifying agents, and BPs discontinued for 3 months before and after elective invasive dental surgeries. Patients should be encouraged to maintain good oral hygiene and clinicians should assess in every visit jaw/tooth pain or exposed bone on clinical examination. A conservative management is recommended with limited debridement, antibiotics and oral rinses (as chlorhexidine) [48].
Other shared side effects from BMAs include [51]:
1.
Hypocalcemia. Patients should be encouraged to take supplemental calcium and vitamin D and serum calcium, magnesium and phosphate monitored during therapy.
2.
Acute phase response. This reaction is characterized by fever and flu-like symptoms occurring in the first 3 days after therapy and shortly resolving. Paracetamol or NSAIDs improve symptoms. It generally does not recur after first or second administration.
BPs have specific side effects [51]:
1.
Nephrotoxicity. Zolendronate induces tubular dysfunction, while pamidronate damages the glomeruli. Patients should maintain adequate hydration and clinicians need to monitor renal function during therapy. A dose reduction is recommended for patients with creatinine clearance <60 mL/min and BPs are contra-indicated for those with creatinine clearance <30 mL/min.
2.
Ocular toxicity. Conjunctivitis, uveitis, scleritis and orbital inflammation were documented.
3.
Joints or muscular pain.
4.
5.
Conflicting evidence associates BPs with a slightly increased risk of developing atrial fibrillation and stroke [54].
Treatment Duration and Schedule
The optimal duration of BP treatment in patients with metastatic bone disease is controversial [47]. Pivotal trials with bisphosphonates showed a reduction of SREs for treatments up to 2 years, while those with denosumab for up to 3 years. A recent ASCO consensus statement recommended treatment with BMA to be continued until evidence of substantial decline in patient’s general performance status is noted [47]. An international consensus panel recommended that the decision to maintain treatment beyond 2 years should be assessed in a case-specific manner [55]. The consequences of stopping bone-modifying agents after one or more adverse skeletal-related events are not clearly defined [47]. There is some concern that discontinuation of nonbisphosphonate antiresorptive agents can lead to a bone turnover rebound, however scarce data in patients with osteoporosis showed no excess risk of fracture after treatment discontinuation [56, 57].
With the objective to decrease treatment toxicity and hospital visits, OPTIMIZE-2 and ZOOM trials tested and demonstrated that a less frequent administration of zoledronic acid (every 12 weeks instead of every 4 weeks) after approximately 1 year of every 4 weeks therapy was a non-inferior option [58, 59]. However, a more irregular control of bone markers was found. The implications of this finding are unknown. More recently, CALGB 70604 tested the non-inferiority of an upfront de-escalated regimen of ZA (every 12 weeks vs. every 4 weeks) in 1822 patients with breast (833), prostate (674), myeloma (270) and other (45) tumors. Overall, at 24 months, the proportions of SRE were 29.5 % vs. 28.6 % for every 4 and every 12 weeks, respectively (P = 0.79) [60]. An ongoing trial is testing a similar de-escalated regimen for denosumab (NCT02051218). Finally, a recent meta-analysis including data from 1026 patients recruited for 6 studies of BMA (4 trials with BP and 2 with denosumab; excluding CALGB 70604) found no difference in the rate of on-study SRE between every 4 weeks and every 12 weeks (de-escalated) regimens of BMA [61]. Of note, current international guidelines still do not support the use of de-escalated regimens.
Other strategies to reduce exposure to BMA were tested, namely treatment scheduling according to bone resorption markers [62]. However, this approach did not demonstrate non-inferiority to current practice.
40.4.1.3 Radiation Therapy
In the setting of metastatic bone disease, radiotherapy (RT) is used to relieve pain, prevent and treat bone fractures and spinal cord compression and to consolidate treatment after surgical management [63, 64]. In the majority of the cases, RT is combined (concomitantly or sequentially) with other treatment modalities, namely surgery [63, 64], other anti-neoplastic therapies or bisphosphonates [65].
There are different hypofractionation schemes (e.g. 30 Gy in 10 fractions/daily, 20 Gy in 5 fractions/daily or 8 Gy in a single fraction) that can be used in this context with palliative intention [63, 64, 66, 67]. The choice of the fractionation scheme is mainly determined by patient characteristics (e.g. performance status, co-morbidities, compliance to immobilization for radiation session, setup accuracy and socio-economic support), tumor/metastases characteristics (e.g. type of primary tumor and its radio-sensitivity, staging, localization, weigh-bearing vs non-weight bearing sites, size, osteoblastic vs osteoclastic, growth rate, presence of soft tissue involvement and risk of complications), symptoms characteristics and previous treatments (medical or surgical with or without insertion of medical devices). This section will review the use of RT for different indications in the setting of bone metastases.
Pain Control
For the palliation of pain associated with bone metastases , RT can induce pain relieve (any degree of pain control) in 60–80 % of cases and a complete pain resolution in 15–30 % of the cases within 3–4 weeks of treatment [63, 64]. Pain control with RT can be attempted in the following scenarios: localized non-complicated painful bone metastases, localized painful bone metastases with neuropathic pain and diffuse bone metastases.
Localized Non-complicated Painful Bone Metastases
A 2007 systematic review from Chow et al. (updated in 2012) [68] showed similar results between a single fraction versus multiple fractions schemes in terms of pain control (overall pain response rates of 60 % vs. 61 % for multiple schemes with a pooled odds ratio of 0.98 [95 % CI 0.95–1.02]; and pain complete response rates of 23 vs. 24 % for multiple schemes with a pooled odds ratio of 0.97 [95 % IC 0.89–1.06]). Of note, variable definitions of pain response rate were used across the trials that contributed to this review, and for the purpose of the meta-analysis, both overall and complete responses were considered as used and reported in each trial.
When compared to non-single fractioning schemes, several other studies report not only similar efficacy, but better cost-effectiveness and easy implementation [66, 67, 69, 70].
Therefore, for patients with localized non-complicated bone pain, an international consensus suggests the use of external beam radiotherapy (EBRT) in an 8 Gy single fraction scheme instead of other hypofractioning schemes [67]. However, current implementation of this strategy is still low, with single fraction schemes still being mainly used in patient with low life span, low performance status, low compliance and transportation issues [69].
Noteworthy, single fraction schemes needed re-treatment more frequently (20 % vs. 8 % for multiple fraction schemes) with a 2.6-fold higher likelihood for re-irradiation (95 % CI 1.92–3.47; P < 0.001). In these studies re-treatment was indicated at discretion of the treating physician, which could have possibly introduced study bias [64, 66]. Regarding the optimal dose of single fraction schemes, a systematic review from Dennis et al. [71] concluded that 8 Gy were superior to 4 Gy. In the intention-to-treat (ITT) analysis, the 8 Gy dose was superior to the 4 Gy dose regarding pain control overall response rate (ORR; 21–81 % vs. 23–47 %) and complete response rate (CRR; 9–52 % vs. 15–18 %). When patient’s assessment (PA) analysis was applied, the 8 Gy dose was also superior to the 4 Gy dose in terms of pain control overall response rate (ORR; 31–93 % vs. 44–47 %) and complete response rate (CRR; 14–57 % vs. 15–26 %).
If re-irradiantion is needed, it is recommended a minimum of 4 weeks interval between RT treatments [63, 64, 67]. A meta-analysis by Huisman et al. documented pain relief response rates after re-irradiation in 58 % of the patients (95 % CI 0.49–0.67). The quality of this study was however poor given the high heterogeneity between studies (I2=63.3 %) and the high drop-out rates (14–35 %) [72].
The phase III trial RTOG 0433/NCIC CTG SC 20 compare the overall pain response rate (complete response and partial response) at two months after the first fraction of re-irradiation. In this non-inferiority trial, 850 patients were randomized (1:1) to receive a single-fraction radiotherapy (8 Gy) on day 1 or a multiple-fraction scheme (to a total of 20 Gy) over 5 days or over 8 days in the case of spine and/or whole pelvis re-irradiation (prior radiotherapy needed to be administered in multiple fractions). Unfortunately, this trial experienced significant limitations in the intention-to-treat analysis (around 30 % of patients in both arm were not assessable at 2 months). In the intention-to-treat population, 28 % of the patients allocated to 8 Gy treatment and 32 % allocated to the 20 Gy treatment had an overall pain response (p = 0.21; response difference of 4.0 % [upper limit of the 95 % CI 9.2, less than the pre-specified non-inferiority margin of 10 %]). Similar results were found in the per-protocol population. Despite the study limitations, 8 Gy in a single fraction seems to be as good as 20 Gy in multiple fractions. Moreover, trade-offs between efficacy and toxicity profiles should be taken into account [73].
Localized Painful Bone Metastases with Neuropathic Pain
Patients can also experience neuropathic bone pain with significant impact in QOL. The optimized RT fractionation for this type of pain remains unclear. There are two possible mechanistic pathways for this pain entity: mechanical pressure vs. humoral insult by cytokines or host cell pathological reaction (e.g. osteoclasts). These hypothesis impact the best clinical approach, namely if it should be used a higher RT dose (to shrink tumor) or lower dose (as an “anti-inflammatory” treatment) [74]. Scarce data is available on this issue and only the TROG 96.05 approached it.
The TROG 96.05 trial [74] compared a non-single scheme of 20 Gy in 5 fractions to a 8 Gy scheme in a single fraction for neuropathic bone pain. A trend toward best overall response and complete response for the non-single scheme of 20 Gy in 5 fractions was noted. The 8 Gy single fraction had an ORR (ITT) of 53 % (95 % CI 45–62 %), while the non-single scheme of 20 Gy in 5 fractions had an ORR of 61 % (95 % CI 53–70 %; P = 0.18). As for CRR, The 8 Gy single fraction had a 26 % (18–34 %) response vs. 27 % (19–35 %; P = 0.89) response in the non-single scheme of 20 Gy in 5 fractions. Furthermore, the estimated median time for treatment failure (TTF) showed also a trend toward the non-single scheme arm (TTF of 2.4 vs. 3.7 months for the single fraction arm; HR 1.35; 95 % CI 0.99–1.85; log-rank P = 0.056). Moreover, patients receiving a single fraction 8 Gy scheme had a higher consumption of analgesics and hospital related admissions costs [75]. Thus, most physicians opt for a non-single fraction scheme for neuropathic painful bone metastases.
Until further studies are available, the current evidence favours a non-single fraction RT for neuropathic pain. Single fraction RT can be reserved for less fit patients.
Approach to Diffuse Bone Metastases : Radiotherapy vs. Radionuclides
In cases of diffuse painful bone metastases affecting large anatomical areas and with poor response to systemic medical treatments two treatment options are available: hemi-body/wide-field irradiation or intravenous radiopharmaceuticals.
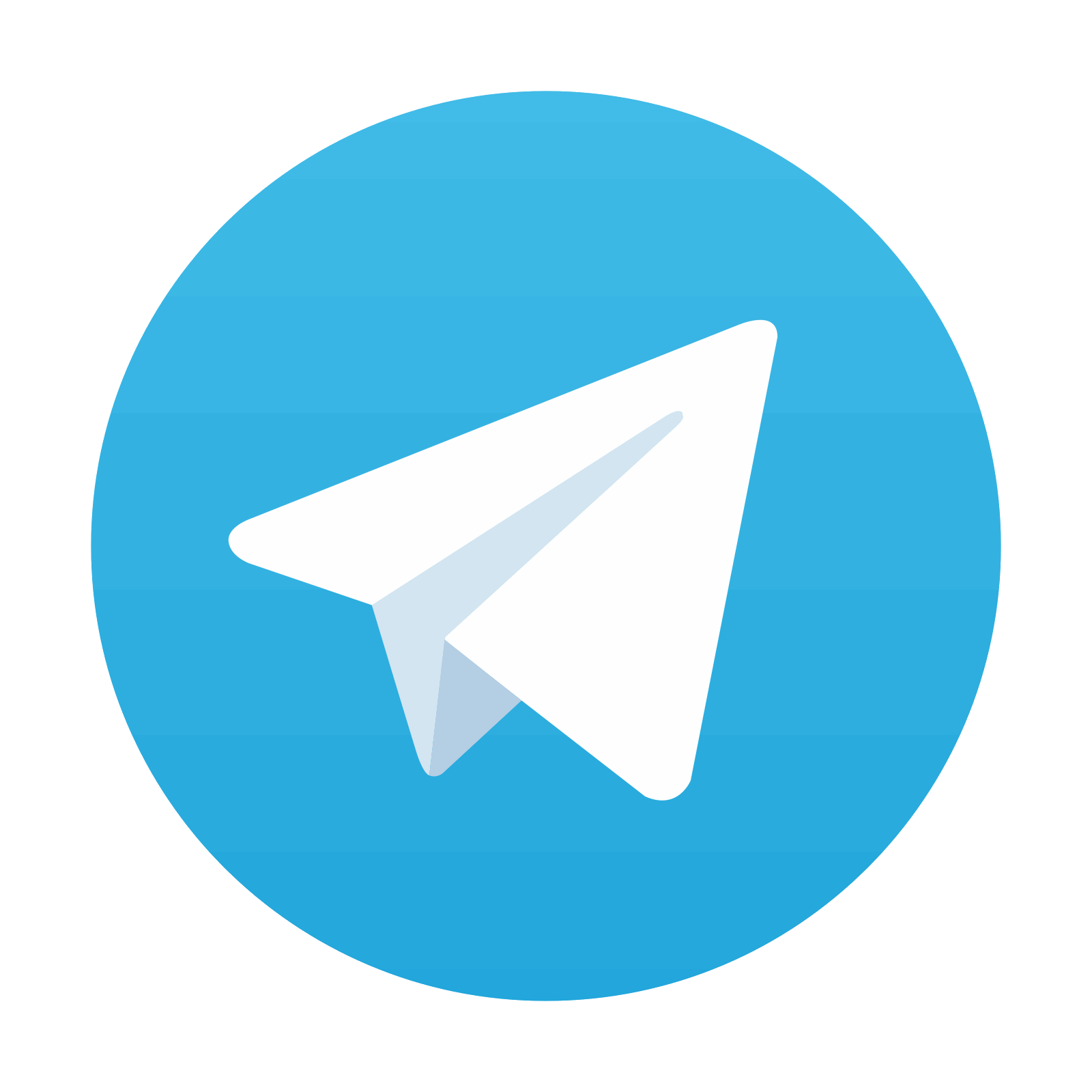
Stay updated, free articles. Join our Telegram channel

Full access? Get Clinical Tree
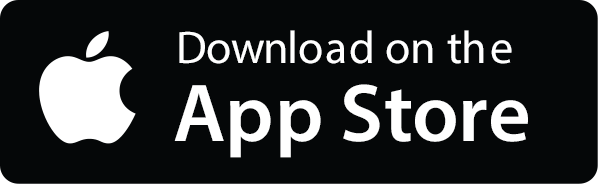
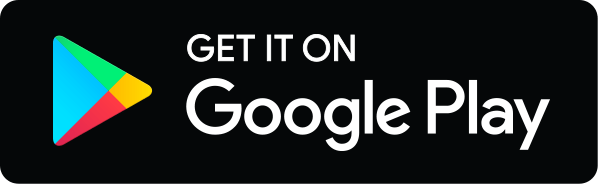
