Bone Scintigraphy
Hossein Jadvar
LEARNING OBJECTIVES
1. Understand the diagnostic utility of bone scintigraphy in the imaging evaluation of the skeleton in various relevant clinical conditions.
2. Understand the limitations of bone scintigraphy in the imaging evaluation of the skeleton.
3. Discuss non-skeletal imaging utility of bone scintigraphy.
INTRODUCTION
Nuclear medicine has played a major role in the imaging evaluation and treatment of skeletal system. Bone is a crystalline lattice that is comprised of calcium, phosphate, and hydroxyl ions. Bone-seeking radiopharmaceuticals are typically calcium, phosphate, or hydroxyl analogs. The most common radiotracers for imaging are technetium-labeled phosphate analogs including methylene diphosphonate for single-photon planar or single-photon emission computed tomography (SPECT) scintigraphy. Fluorine is a hydroxyl analog and can be used as 18F-sodium fluoride (18F-NaF) with positron emission tomography (PET).
Bone scintigraphy is used for imaging evaluation of infection (e.g., osteomyelitis), noninfectious inflammation (e.g., arthritis), trauma, metabolic bone disease, benign and malignant neoplasms, and specific conditions in children. The scan may include dynamic image data acquisition and involve the whole skeleton or only a specific region of the body. Bone scintigraphy is most effective when correlated with other relevant imaging modalities such as radiography, computed tomography (CT), and magnetic resonance imaging (MRI), which often assists with improving specificity. Procure standards and practice guidelines with either single-photon or positron imaging have been published (1,2,3).
The uptake of the bone-seeking radiotracers is dependent on blood flow and chemisorption to bone matrix. The skeleton is imaged about 3 h following intravenous administration of the single-photon radiotracer. The delay in imaging improves the target-to-background uptake ratio. With 18F-NaF PET, the tracer uptake is based on chemisorption with an exchange of 18F– ion for OH– ion on the surface of the hydroxyapatite matrix of the bone, forming fluoroapatite and migration of the 18F– ion into the bone crystalline matrix. Due to high first-pass extraction from plasma and rapid renal clearance, imaging with PET can be performed in a shorter time (typically at 45 min to 1 h after intravenous tracer administration) in comparison to 99mTc-based radiotracers.
NORMAL BIODISTRIBUTION
The normal bone scan shows relatively homogenous tracer distribution in the axial and appendicular skeleton with most activity in the axial skeleton (Fig. 8.1). The calvarial activity may be somewhat patchy. In children, the epiphyseal plates show relatively symmetric and intense tracer accumulation, which diminishes as the child grows into adulthood. There may be increased foci of activity, which may develop with the aging process including degenerative changes (e.g., osteophytosis and arthropathy), and bursitis at tendon insertions. An optimal quality bone scan shows little soft tissue activity with a high bone-to-background activity ratio. Renal and bladder urine activities are reflective of physiologic route of tracer excretion. Unless there are asymmetries due to patient positioning, observed asymmetries in osseous activity may be a sign of abnormality and should be further examined with spot planar imaging, SPECT(/CT), other correlative imaging, and clinical information. The bone-to-background activity ratio (sensitivity) and specificity are generally higher with 18F-NaF PET/CT than planar or whole-body SPECT/CT bone scintigraphy (4).
CLINICAL APPLICATIONS
There is a wide range of clinical applications for bone scintigraphy. These include but are not limited to the following indications:
Bone pain with normal radiograph.
Infection (e.g., osteomyelitis vs cellulitis).
Trauma (e.g., stress fracture, non-accidental injury in children).
Determination of bone viability.
Evaluation of prostheses (infection vs loosening).
Evaluation of metabolic bone disease.
Staging and treatment monitoring of cancer.
Benign bone lesions
A potential cause for painful bone without a definite radiographic abnormality is osteoid osteoma, which can show high focal activity at the site of the lesion’s vascular nidus. Fibrous dysplasia is
another benign bone condition that can present as a single lesion or multiple lesions (polyostotic fibrous dysplasia) with typically increase radiotracer accumulation (Fig. 8.2). Correlation with clinical and other imaging information can enhance specificity for differential diagnosis (5).
another benign bone condition that can present as a single lesion or multiple lesions (polyostotic fibrous dysplasia) with typically increase radiotracer accumulation (Fig. 8.2). Correlation with clinical and other imaging information can enhance specificity for differential diagnosis (5).
Infection
The common clinical indications for bone scintigraphy are detection, differentiation (osteomyelitis vs. cellulitis or both), and determination of the extent of infection. Three-phase bone scintigraphy (first phase—flow, second phase—blood pool or tissue, and third phase—delayed or metabolic) can be useful in the differential diagnosis of osteomyelitis and cellulitis. In osteomyelitis, all three phases of bone scintigraphy will be abnormal (hyperperfusion, hyperemia, and hypermetabolic), but in cellulitis, the abnormality is early and compartmentalizes to the soft tissue (Fig. 8.3). Additional imaging with bone marrow scanning and radiolabeled leukocyte scan may be needed for improved specificity (6,7,8).
Trauma
Bone fractures may be evident on bone scan within 24 h after the injury. However, in the elderly and patients with diminished bone mineral density, increased tracer accumulation at the site of fracture may be delayed. Non-complicated healing will result in a decline in tracer uptake within a few years. Complicated (e.g., infected) non-healing (e.g., continued trauma) fractures may display high tracer accumulation chronically. Correlative clinical and imaging information can help distinguish pathologic from non-pathologic fractures.
Bone vascularity and viability
Bone scintigraphy can be used to determine blood supply to and viability of bone grafts (e.g., after surgical implantation) and specific sites in the skeleton. Aseptic necrosis is caused by avascular necrosis of bone due to a variety of causes (e.g., trauma and steroids). There is initially decreased tracer activity due to diminished vascularity followed by repair phase, which shows increased activity. Correlation with clinical history and other imaging modalities (particularly MRI) can be helpful for accurate
diagnostic assessment. After radiation therapy, there can be a decline in tracer accumulation in view of diminished vascularity and viable cellularity. Another condition related to vasomotor instability is reflex sympathetic dystrophy syndrome that can be a consequence of a variety of etiologies (e.g., trauma and neurologic abnormality) and associated with pain, swelling, and tenderness. Three-phase bone scintigraphy shows increased perfusion to the affected limb with increased juxtaarticular activity (10,11,12) (Fig. 8.5).
diagnostic assessment. After radiation therapy, there can be a decline in tracer accumulation in view of diminished vascularity and viable cellularity. Another condition related to vasomotor instability is reflex sympathetic dystrophy syndrome that can be a consequence of a variety of etiologies (e.g., trauma and neurologic abnormality) and associated with pain, swelling, and tenderness. Three-phase bone scintigraphy shows increased perfusion to the affected limb with increased juxtaarticular activity (10,11,12) (Fig. 8.5).
Prosthesis evaluation
A common clinical request for bone scintigraphy is the discrimination of prosthesis loosening from infection, which requires different clinical management. With regard to hip prosthesis, persistent activity at the caudal tip of prosthesis and the trochanteric region may signal prosthesis loosening (Fig. 8.6). However, in the case of prosthesis infection, there can be generalized hyperactivity around the prosthesis. Bone scintigraphy is also sensitive but nonspecific for differentiating among various conditions that cause painful knee prostheses. If further imaging evaluation is needed, radiolabeled leukocyte scan, 68Ga-citrate scintigraphy, or PET with 18F-fluorodeoxyglucose (18F-FDG) can be contributory (13,14,15,16,17).
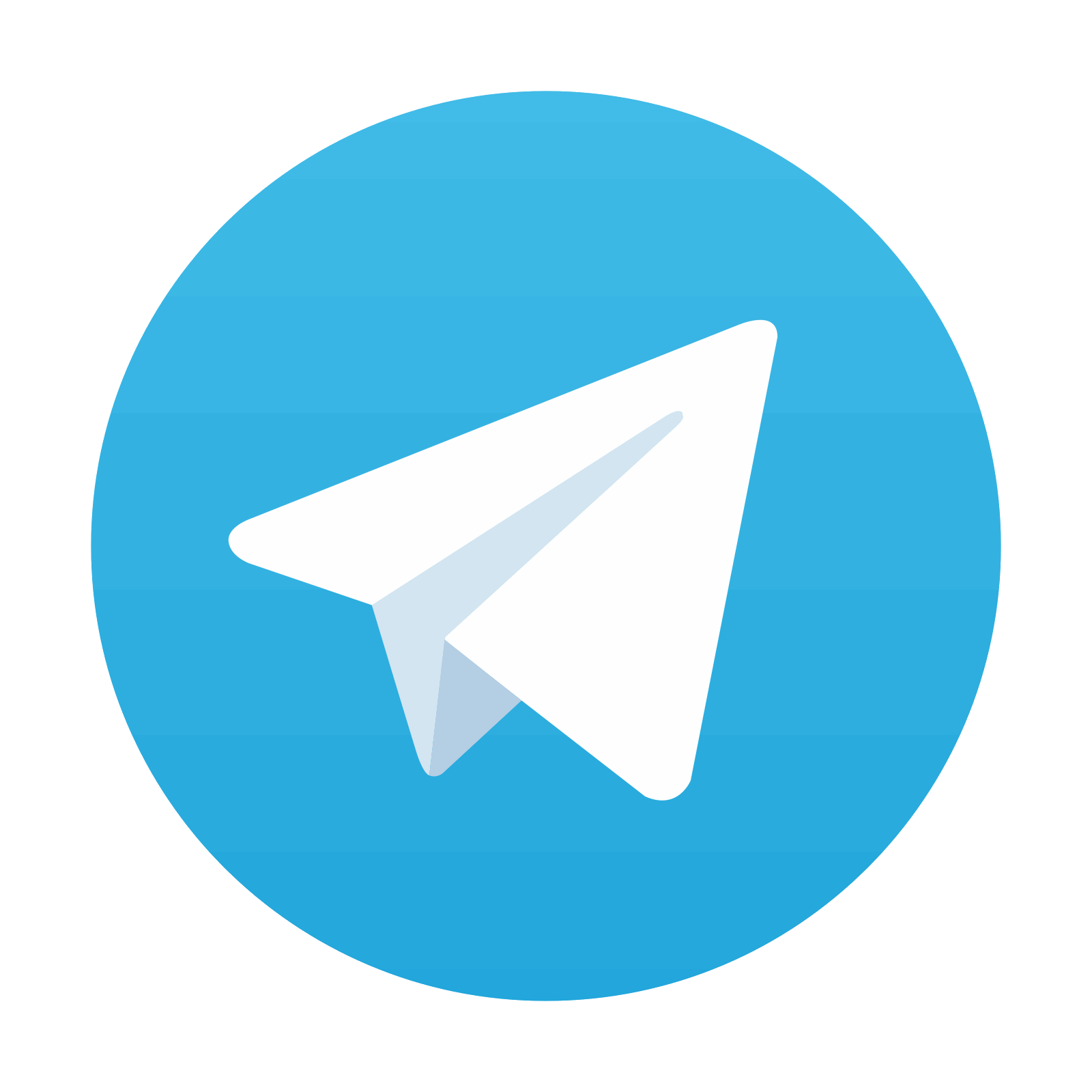
Stay updated, free articles. Join our Telegram channel

Full access? Get Clinical Tree
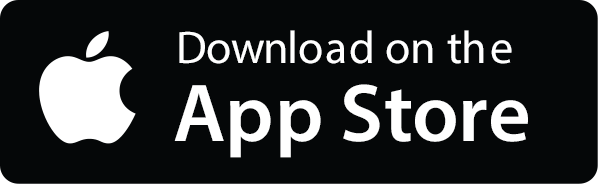
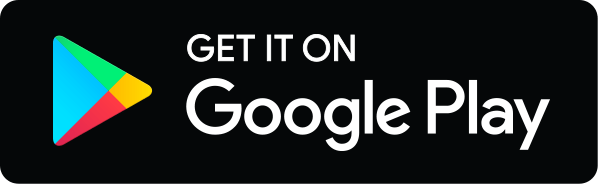