Thyroid Imaging and Therapy
Erik S. Mittra
Hong Song
LEARNING OBJECTIVES
1. Understand the role of nuclear radiology in the evaluation and treatment of patients with hyperthyroidism.
2. Understand the role of nuclear radiology in the evaluation and treatment of patients with thyroid cancer.
INTRODUCTION
Radioiodine therapy of hyperthyroidism is the origin of the field of Nuclear Medicine with the first treatments in 1941 (1), followed by the official publications documenting their efficacy in 1946 (2,3). Since then, of course, radioiodine imaging has also come to play an integral role both independent of and as an adjunct to radioiodine therapy both for hyperthyroidism and thyroid cancer. The difference between the latter two indications cannot be overemphasized, and is often a source of confusion for those new to the field. While it is true that the diagnostic and therapeutic radiopharmaceuticals I-123 and I-131, respectively, are the same for both hyperthyroidism and thyroid cancer, every other aspect of their use is different including the pathology, diagnostic algorithms, treatment algorithms, preparation for and types of scans, the doses used for therapy and how to determine the dose, and the radiation precautions associated with each therapy. As such, the role of radioiodine in hyperthyroidism and thyroid cancer will be described separately in this chapter.
By way of brief background, the thyroid is a small endocrine gland located in the low-anterior neck, overlying the thyroid cartilage, and is responsible for the production of the thyroid hormones triiodothyronine (T3) and thyroxine (T4), as well as the hormone calcitonin. These hormones influence metabolic rate and protein synthesis, and calcium homeostasis, respectively. The production of these hormones is in turn controlled by thyroid-stimulating hormone (TSH) production from the anterior pituitary gland and thyrotropin-releasing hormone (TRH) by the hypothalamus (4).
HYPERTHYROIDISM
Background
Hyperthyroidism is a syndrome caused by higher than normal levels of serum thyroid hormone (T4 and/or T3). Usually, this causes suppression of TSH, which then becomes a reliable marker of the disease. There are many causes of hyperthyroidism, but broadly, it is either due to overstimulation of the thyroid epithelium causing overproduction of hormones, or else destruction of the thyroid follicles and epithelium resulting in release of stored thyroid hormones, termed “thyroiditis.” The most common cause of overproduction is either diffuse toxic goiter (Graves’ disease) or nodular toxic goiter, and these tend to be a permanent issue in most patients. Less common causes include hyperfunctioning thyroid tumors, hashitoxicosis, secondary and tertiary hyperthyroidism, struma ovarii, or other tumors causing overproduction of TSH or TRH. There are many etiologies of thyroiditis including subacute, mechanical destruction of the thyroid, medications such as amiodarone, related to pregnancy, silent, and others (5). The broad divide between overproduction versus destruction as a cause of hyperthyroidism is important for imaging and therapy with radioiodine because overproduction results in increased uptake of radioiodine and therefore can be used to treat it, whereas destruction results in low uptake of radioiodine and therefore cannot be used to treat it (6).
General diagnostic and treatment algorithm
Once hyperthyroidism is biochemically confirmed, the next step is typically a radioiodine uptake and scan (6). A neck ultrasound (with or without fine needle aspiration [FNA]) may also be done if there are palpable nodules or if the radioiodine scan demonstrates hyper- or especially hypofunctioning nodules. If the cause of the hyperthyroidism is due to thyroiditis, typically only symptomatic management is needed, as the disease is self-limited. If the cause is due to overproduction of thyroid hormone, typically the first step is medical management with propylthiouracil (PTU) or methimazole (MMI). However, these medications can be difficult to manage and have significant potential side effects including agranulocytosis, liver failure, and birth defects (7), so they typically cannot be used in the long term. Definitive treatment options are either surgical removal of part or all of the thyroid gland or radioiodine ablation of the thyroid. Both have various pros and cons (8) which should be carefully discussed with the patient prior to a decision for therapy.
Role of nuclear imaging
As mentioned, the main indication for a radioiodine thyroid uptake and scan is for further evaluation of biochemically confirmed hyperthyroidism (9) (Figs. 4.1,4.2,4.3,4.4,4.5,4.6). Less commonly, it
can be used to evaluate the functional status of a nodule seen on ultrasound even in the face of normal labs. The rationale being that hypofunctioning nodules are more likely to be cancerous than hyperfunctioning ones and so merit biopsy (10) Nowadays, ultrasound features rather than radioiodine uptake often makes this determination although it remains a consideration.
can be used to evaluate the functional status of a nodule seen on ultrasound even in the face of normal labs. The rationale being that hypofunctioning nodules are more likely to be cancerous than hyperfunctioning ones and so merit biopsy (10) Nowadays, ultrasound features rather than radioiodine uptake often makes this determination although it remains a consideration.
Preparation for an uptake and scan involves being off of thyroid medications (PTU or MMI) for at least 5 days and ensuring that no exogenous iodine (most commonly from a diagnostic computed tomography (CT) with intravenous-iodinated contrast) has been administered in the 6 weeks prior to therapy (11). For the same reason, it is ideal if the patient does not have an iodinerich meal (i.e., seafood or seaweed) in the 48 h prior to imaging. A true low-iodine diet, however, is typically not necessary.
The procedure is composed of two distinct parts: an uptake measurement (non-imaging using a thyroid probe) and a scan (imaging using a gamma camera). Both parts can be done using a single administration of I-123. The uptake can also be done separately using a smaller (half) dose of I-123. After oral administration of the pill, the patient returns 24 h afterward for the uptake measurement. And optional 4-h measurement is also done at some centers and can be useful for patients with high turnover to know whether the uptake is increasing or decreasing over time. There are several ways to do the uptake measurement. A common way is as follows. The thyroid probe is set a fixed distance away from the patient’s neck to record the uptake. The patient’s background counts are also measured by the thyroid probe pointed toward the mid-thigh. An equivalent standard dose of I-123 is then placed in a lucite scattering neck phantom and measured by the thyroid probe, and lastly the room background is measured. The patient’s neck counts minus their thigh counts is divided by the standard counts minus the room background (11).
Normal radioiodine uptake is 6% to 18% at 4 h and 10% to 30% at 24 h (12).
Normal radioiodine uptake is 6% to 18% at 4 h and 10% to 30% at 24 h (12).
![]() FIG. 4.1 • I-123 scan showing physiologic uptake in the bilateral thyroid lobes. The 24-h radioiodine uptake (RAIU) is 16.6% (normal 10%-30%). |
![]() FIG. 4.2 • I-123 scan showing a solitary hyperfunctioning nodule in the inferior right thyroid lobe, with relative suppression of the remaining normal thyroid gland. The 24-h RAIU is 39.3%. |
![]() FIG. 4.3 • I-123 scan showing multiple hyperfunctioning nodules, with relative suppression of the remaining normal thyroid gland. The 24-h RAIU is 35.9%. |
![]() FIG. 4.4 • I-123 scan showing hyperfunctioning nodules in the mid-right and upper-left thyroid lobes as well as hypofunctioning nodule in the mid-left thyroid lobe. The 24-h RAIU is 41.4%. |
![]() FIG. 4.5 • I-123 scan showing diffusely increased uptake in the bilateral thyroid lobes with visualization of the pyramidal lobe as well as the thyroid isthmus. The 24-h RAIU is 74.0%. |
The scan using a gamma camera is typically done with a pinhole collimator, focused on the neck. If there is low uptake, a Technetium-99m marker is useful to mark the top of the thyroid cartilage as well as the sternal notch, as the anatomy is otherwise unclear. If the patient has a palpable nodule, or known nodules >1 cm by ultrasound, it is also useful to try and mark where these are located while the patient is being imaged, so a direct correlation of nodule uptake can be made. In practice, this can be challenging unless the nodule is superficial, large, or the patient is thin.
Interpretation of the scan and uptake is relatively straightforward. Uptake values below the normal range suggest thyroiditis as the gland has released its stored thyroid hormone, which subsequently suppresses the TSH and the gland. In this case, the scan will show limited or no uptake in the thyroid. Uptake values higher than the normal range suggest either Graves’ disease, disease or toxic nodular goiter as the gland is active despite a low TSH. In this case, the scan will show diffuse uptake for Graves’, often with visualization of the thyroid isthmus and pyramidal lobes. For toxic nodule(s), the scan will typically show high uptake in the nodule(s) with variable suppression of the surrounding thyroid gland. Because of the latter issue, the uptake value may actually be in the normal range for nodular goiter if the uptake in the nodule is balanced by the low uptake in the suppressed gland. If the scan and uptake results are clear, then obtaining thyroid function tests at the time of the scan has limited added value. If, however, the uptake and scan is normal, then obtaining concurrent thyroid function tests is useful to know if the thyroid labs are also normal or abnormal at the time of the study (9).
Radioiodine therapy
A hyperthyroidism patient would be amenable to therapy with I-131 if the following conditions are met: (1) The radioiodine uptake at 24 h is greater than 30%, (2) the scan shows either diffusely increased uptake or solitary or multiple hyperfunctioning nodules, and (3) there are no hypofunctioning nodules seen which have not already been evaluated by ultrasound and/or biopsy to confirm they are not malignant. Other factors to consider are to ensure the patient has had a thorough discussion with their endocrinologist regarding alternative treatment options, a negative urine or serum pregnancy test within 48 h of the planned therapy, and appropriate counseling about radiation precautions including avoiding pregnancy. If the uptake is high normal, but the patient is otherwise a good candidate for radioiodine therapy and had proper preparation, then it may be beneficial to have the patient undergo a low iodine for 1 or 2 weeks and return for an uptake measurement. The idea is to further stimulate the thyroid gland and increase the uptake value above normal. This is beneficial to enhance the efficacy of the therapy.
![]() FIG. 4.6 • I-123 scan showing almost no uptake in the bilateral thyroid lobes. The gland is barely visible above background. The 24-h RAIU is 1.1%. |
The dose of I-131 for therapy of hyperthyroidism can either be chosen empirically or by calculation (13). For Grave’s disease, empiric doses are typically in the range of 10 to 15 mCi and for hyperfunctioning nodule(s) in the range of 10 to 20 mCi as the latter can be more radioresistant. Alternatively, the dose can be calculated to deliver a specific radiation dose to the gland or nodule(s). For Grave’s disease, the formula is 50 to 200 µCi/g of tissue, divided by the radioiodine uptake. For hyperfunctioning nodules, the formula is 150 to 200 µCi/g of tissue, divided by the radioiodine uptake. That is, the dose is directly related to the gland size and indirectly related to the uptake. To use the formula, then, the gland size must be known. Here, again, there are two methods. The most accurate is to use the measurements from a recent thyroid ultrasound. In this case, the formula for gland size is that of an oval (length × width × height/2). When an ultrasound is not available, a physical exam can be used to estimate the gland size. In this case, one must know that the average total gland size in an adult is 20 g (10 g/side). A gland that can easily be palpated but not seen is approximately 40 g in size. A gland that can easily be seen is 60 g. And a large goiter may be 80 to 100 g in size. This method is prone to error in larger patients in whom a large portion of the gland is hidden behind overlying soft tissue.
The goal of the therapy is not necessarily to achieve an euthyroid state, but rather to destroy the gland completely and render the patient hypothyroid. When that happens, they will require thyroid hormone replacement for the remainder of their life. Even with this approach, up to 20% of patients may require a second treatment to be fully effective (8). Lower doses are correlated to greater treatment failure (13) but this should be balanced by not giving high-radiation doses to everyone, which may increase the risk of side effects (see “Side effects and radiation precautions”) as well as their life time radiation burden.
The timeline of therapy should be clarified with patients as many believe the I-131 will be immediately effective. Once the I-131 is localized to the thyroid gland, the emitted beta radiation will cause mostly single-stranded deoxyribonucleic acid (DNA) damage which ultimately cannot be effectively repaired by the cell, causing it to die (14). This process can take many weeks or months to have an effect on the whole thyroid gland. During this time, the patient’s thyroid function should be checked periodically and symptomatic patients should continue their PTU or MMI and other medications such as beta-blockers for palpitations. Over time, the patient will slowly transition from hyperthyroid to euthyroid to hypothyroid (13).
Side effects and radiation precautions
Side effects of radioiodine treatment can be divided into those which are short and long term (14). Short-term side effects typically begin and also resolve within the first several days after the therapy. They include nausea (rarely strong enough to cause emesis), sialadenitis, swelling of the thyroid gland, change in taste, and generalized malaise. Ensuring the patient is well hydrated and controlling symptoms with non steroidal anti-inflammatory medications and/or antiemetics can mitigate these effects. Sialagogues such as sour candies or lemon have been advocated to reduce the risk of sialadenitis, but the timing of their use is not well understood (15). Several studies show that starting sialagogues immediately after I-131 administration (and continuing for several days) can reduce the uptake of I-131 in the salivary gland (16,17). However, one study showed that starting within 24 h of the I-131 administration can actually increase uptake in the salivary gland (so called “rebound effect”) resulting in greater side effects (18).
Long-term side effects of radioiodine therapy include prolonged or permanent damage to the salivary glands causing persistent dry mouth. Infrequently, this can present itself several months after the therapy even in patients who did not initially experience sialadenitis. Again, the use of sialagogues has been shown to reduce these effects. Also, rarely, alteration in taste can be more prolonged or rarely permanent. There is a theoretical risk of developing a second malignancy from the radiation, but this is more of a concern at the higher doses used for thyroid cancer, not with the low doses used for hyperthyroidism.
Radiation precautions associated with radioisotope therapies of any type are a source of great confusion for physicians and patients alike and there is variability based on geography, institution, and organization (19). The US Nuclear Regulatory Commission (NRC) provides guidance on this but their interpretation can vary. The reason for this is most likely because the effects of such low-level radiation exposure are not well known and mostly safe. With any radioisotope therapy, the two types of radiation to consider is emitted radiation and cleared radiation (primarily from the urine). The NRC guidelines for release of radioactive patients from the hospital are three fold, (1) if the administered dose is less than 33 mCi, (2) if the emitted radiation is less than 7 mR/h at 1 m from the patient, and (3) if the total radiation exposure to a caregiver or family member is less than 500 mrem. Given the doses discussed for hyperthyroidism, the first two criteria are always met and so the patient does not need to be admitted. Having said that, it is probably advisable for the patient to keep some distance from others (especially children less than 11 years of age, or pregnant women) for the first several days after therapy to allow for the majority of the clearance to happen. The cleared radiation is of slightly greater concern primarily to avoid contamination at home. To that end, good bathroom hygiene for the first week after therapy is advised. This includes avoiding spills (men should sit when urinating), flushing (with the lid down) 2 to 3 times after using the toilet, cleaning up any spills, washing hands thoroughly after using the bathroom, and taking daily showers to minimize the radiation in sweat.
THYROID CANCER
Background
Thyroid cancer incidence has steadily been increasing over time, although the mortality has been relatively stable except for advanced-stage disease (20,21). Possibilities for this discrepancy include increased detection of subclinical thyroid cancer such as microcarcinomas (less than 1 cm in size), increased radiation exposure to the general population, as well as other endocrinological, and environmental factors such as increasing obesity. There are several pathologic subtypes of thyroid cancer, although the most common are the differentiated thyroid cancers including papillary and follicular, together accounting for greater than 90% of thyroid cancers. This is fortuitous both because these cancers have a favorable prognosis and because they are amenable to radioiodine therapy. Other subtypes such as medullary thyroid cancer, anaplastic thyroid cancer, and other poorly differentiated thyroid cancers have a worse prognosis and do not take up radioiodine. The remainder of this chapter only pertains to differentiated thyroid cancers.
The most commonly used staging classification for differentiated thyroid cancer is the American Joint Committee on Cancer Tumor Node Metastasis (AJCC/TNM) staging system was revised in October 2016 as the eighth edition. The primary change in the new edition was the downstaging of a significant number of patients into lower stages, reflecting their low risk of thyroid cancer-related death (22,23).
A variety of mutations can also affect differentiated thyroid cancer. The ones of greatest importance for papillary thyroid cancer include RET, BRAF, tyrosine kinase, and TP53. But their importance for prognosis remains incompletely understood. For instance, one study found that the BRAFV600E mutation predicted a worse prognosis (24), while another did not (25). The RAS mutations or PAX8/PPAR gamma rearrangements are more related to development of follicular thyroid cancer.
Treatment overview
Thyroid cancers do not typically cause symptoms and are often found incidentally during a physical exam or by imaging of the neck done for other reasons. However, a suspicious nodule is first identified, the next step is ultrasound-guided FNA followed by a core biopsy. Once differentiated thyroid cancer is confirmed by pathology, the next step is typically total thyroidectomy, although this does vary based on size of the primary tumor (26). Papillary microcarcinomas (those smaller than 1 cm) may undergo
lobectomy or active surveillance (27,28). Based on the pre surgical imaging, a central or lateral neck dissection is also done at the time of the thyroidectomy to sample and remove any metastatic lymph nodes. This surgery is complex given the proximity of the thyroid gland to important vasculature and nerves (e.g., recurrent laryngeal nerve) structures in this area. As such, it is not uncommon for some normal thyroid tissue to be left behind either inadvertently or on purpose.
lobectomy or active surveillance (27,28). Based on the pre surgical imaging, a central or lateral neck dissection is also done at the time of the thyroidectomy to sample and remove any metastatic lymph nodes. This surgery is complex given the proximity of the thyroid gland to important vasculature and nerves (e.g., recurrent laryngeal nerve) structures in this area. As such, it is not uncommon for some normal thyroid tissue to be left behind either inadvertently or on purpose.
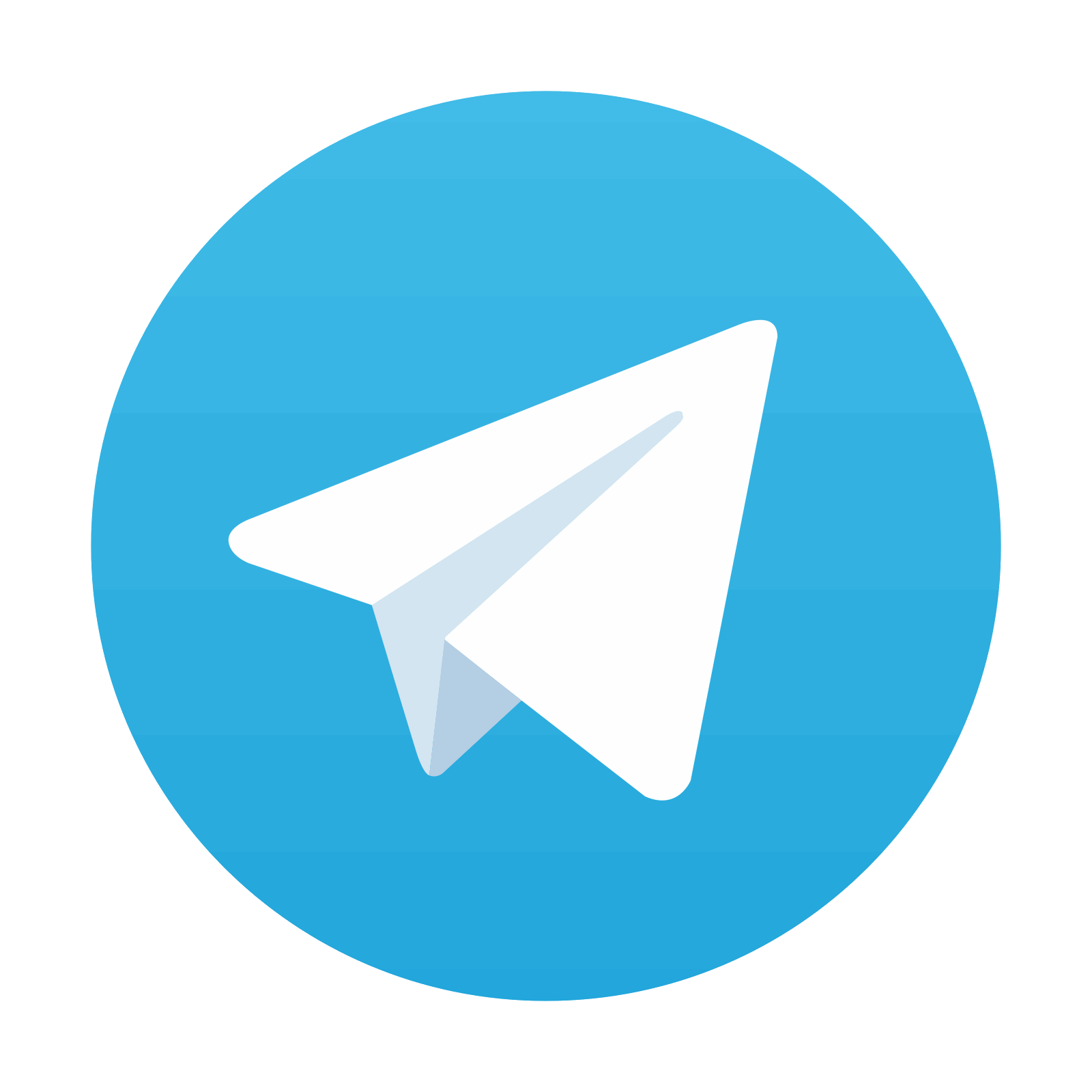
Stay updated, free articles. Join our Telegram channel

Full access? Get Clinical Tree
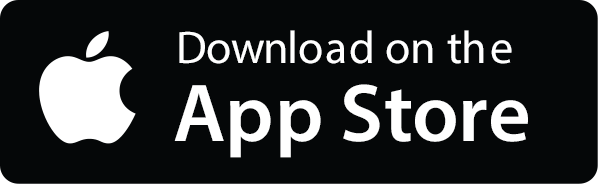
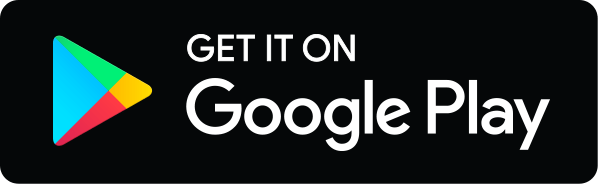