Brain MR Perfusion Imaging: Cerebral Ischemia
Chia-Shang Jason Liu, MD, PhD
Mircea Cristian Dobre, MD, MS
▪ Background
Stroke is a major health care issue in the United States, affecting 6.4 million noninstitutionalized adults in 2012.1 On average between the years of 2009 and 2010, there were 3.7 million visits to physician offices, hospital outpatient offices, and emergency departments with stroke as the primary diagnosis,2 with the average length of stay within a hospital for the treatment of cerebrovascular disease lasting 6.1 days.3 Stroke was the fourth leading cause of death in 2010, behind heart disease, cancer, and chronic lower respiratory disease.4
While stroke is still one of the leading causes of death in the United States, it has decreased in rank over the years, in part due to the increased awareness of symptoms of stroke by the health care profession as well as the public and the emphasis placed on early and rapid treatment of cerebral ischemia. The brain has little capacity to store energy, and its viability is compromised after 3 minutes of vascular occlusion. The therapeutic window is narrow, and without treatment, penumbra will progress to irreversible infarction in a few hours. Current guidelines in the treatment of stroke advocate the delivery of intravenous recombinant tissue plasminogen activator (rtPA) within 3 hours of last known well time before symptom onset, with earlier delivery of rtPA in patients with NIH Stroke Scale (NIHSS) scores of less than 20 and patients less than 75 years of age having the greatest potential for excellent outcome with treatment.5 Even patients undergoing IV rtPA treatment up to 4.5 hours from last known well time have shown clinical benefits as compared to placebo.6
Current treatment guidelines rely on imaging, in particular noncontrast computed tomography (CT), to exclude the presence of intracranial hemorrhage or other causes of the patient’s symptoms such as the presence of intracranial masses.7 There is strong interest in taking into account the physiology of acute ischemia in the treatment algorithm of acute stroke, with the thought that differentiating the amount of viable brain tissue from infarcted tissue may potentially lead to better patient selection and outcomes (Fig. 12.1). This is especially important in order to decide which patients should receive intravenous or intra-arterial treatments as well as selecting patients for treatment who experience “wakeup strokes,” where the onset of initial stoke symptoms cannot be precisely determined and the patients therefore are technically outside of the treatment window of current treatment guidelines. Magnetic resonance imaging (MRI) utilizing diffusion-weighted imaging (DWI) is currently the most sensitive imaging method for the detection of acute ischemia, with high sensitivity and potential for detection of stroke within hours of onset.8,9,10,11 and 12 DWI does not, however, provide physiologic information such as cerebral blood flow (CBF), cerebral blood volume (CBV), and viability of adjacent brain parenchyma at a particular time point.
▪ Perfusion Metrics and Technical Consideration
Dynamic contrast-enhanced (DCE) CT has been used to characterize brain physiology, with the ability to quantify perfusion parameters such as CBF, CBV, and mean transit time (MTT), parameters that are useful for the characterization of stroke as potentially useful for the prediction of the evolution of stroke.13,14 and 15 Similarly, DCE MRI has also been used to obtain the perfusion parameters.16,17 Details with regard to the technical considerations of CT perfusion as well as dynamic susceptibility contrast (DSC) MRI are explained in detail elsewhere in this book. With both techniques, perfusion metrics such as CBF, CBV, MTT, time to peak of the residual function (Tmax), and delay time to peak of the residual function (DT) can be calculated (Figure 12.2). The time to peak is the time from bolus administration to peak signal intensity.
Arterial spin labeling (ASL) is an MRI technique that can also provide information with regard to brain physiology. In ASL, instead of using an intravenous contrast agent, radiofrequency energy is applied proximal to the tissue to be imaged, with the goal of flipping these spins from their normal alignment with the magnetic field.18 This change in proton spin orientation serves as the label that is carried downstream in flowing blood. Inflow of these inverted spins creates a flow-related signal when the water is extracted from the capillary bed or, in the case of a fistula, can be seen in the venous structures. Multiple images can be acquired prior to complete decay of signal, and quantitative analysis is possible using subtraction from thin-section T1W images.18 The short duration of labeling allows for marked sensitivity to changes in arterial transit time.19
While both CT and MR perfusion offer the ability to measure perfusion metrics, MR perfusion has the advantages of being an imaging technique that does not require ionizing radiation and therefore has decreased associated risks of radiation as compared to CT.20 MR perfusion also has the capability for whole-brain calculation of perfusion metrics, whereas most CT perfusion studies typically only provide limited coverage of the brain, with routine CT perfusion slices centered at the level of the basal ganglia so as to maximize the interrogation of arterial territories and to minimize radiation exposure. Being an MRI technique, MR perfusion has the distinct advantage of being a part of an imaging modality that is currently the most sensitive for acute ischemia, with the evaluation incorporated with other MR sequences such as DWI as part of a stroke evaluation.21 Despite these advantages, CT scanners are more widely available than are MRI scanners for the evaluation of stroke, especially in an emergency setting. CT perfusion metrics are also easier to quantify than are MR perfusion metrics due to the linear relationship between iodinated contrast concentration and CT attenuation, which is not the case for gadolinium contrast concentration and MR signal intensity.7 There are also fewer contraindications for a subject to undergo a CT evaluation than an MRI evaluation, such as the presence of a pacemaker, renal failure requiring dialysis, claustrophobia, or presence of metal fragments including, among others, older ferromagnetic medical implants.
Despite their differences, MR and CT perfusion have been shown to generate similar perfusion metrics. When using a Tmax threshold of greater than 6 seconds, there is good agreement in the predicted area of acute ischemia by MR and CT.22 While the literature supports the use of both imaging modalities and their perfusion metrics in following stroke patients, care needs to be taken in terms of the consistent use of perfusion software, the mathematical model used for the calculation of the perfusion metrics, and the threshold used for the generation of ischemia maps.22
With regard to the characterization of brain perfusion in the setting of acute stroke, ASL and DSC MR perfusion demonstrate similar capabilities to characterize perfusion parameters (Fig. 12.3). A territory of decreased relative CBV or CBV with reduced diffusion identifies infarct. A territory of increased MTT or Tmax indicates slow flow through that area of the brain. Matched Tmax and relative
CBV (or diffusion) regions of abnormality indicate complete infarction, and a Tmax abnormality larger than the rCBV (or diffusion) defect indicates that the ischemic area is larger than the infarction, and the difference represents the penumbra or area at risk that requires intervention. ASL has shown similar ability as DSC MR perfusion in delineating regions of hypoperfusion, with similar conspicuity of the lesions using either technique.23 Hyperemic lesions in acute ischemic stroke are more conspicuous using ASL as compared to DSC MR perfusion.23 Other studies have shown ASL to overestimate perfusion defect volume in patients with small final infarcts.24 In general, ASL hypoperfused area corresponds to increase in transit time on DSC. Penumbra manifests as an ASL hypoperfusion territory larger than restricted area on DWI. Abnormally hypoperfused area on ASL with a normal DWI may indicate an ischemic zone that requires further angiographic studies to assess vascular integrity. To date, however, no multicenter trials have used ASL to risk stratify patients into different treatment arms in the setting of acute stroke, perhaps in part due to the relative novelty of ASL compared to DSC MR perfusion and the lack of ability to implement the technique on conventional clinical MR scanners reliably.
CBV (or diffusion) regions of abnormality indicate complete infarction, and a Tmax abnormality larger than the rCBV (or diffusion) defect indicates that the ischemic area is larger than the infarction, and the difference represents the penumbra or area at risk that requires intervention. ASL has shown similar ability as DSC MR perfusion in delineating regions of hypoperfusion, with similar conspicuity of the lesions using either technique.23 Hyperemic lesions in acute ischemic stroke are more conspicuous using ASL as compared to DSC MR perfusion.23 Other studies have shown ASL to overestimate perfusion defect volume in patients with small final infarcts.24 In general, ASL hypoperfused area corresponds to increase in transit time on DSC. Penumbra manifests as an ASL hypoperfusion territory larger than restricted area on DWI. Abnormally hypoperfused area on ASL with a normal DWI may indicate an ischemic zone that requires further angiographic studies to assess vascular integrity. To date, however, no multicenter trials have used ASL to risk stratify patients into different treatment arms in the setting of acute stroke, perhaps in part due to the relative novelty of ASL compared to DSC MR perfusion and the lack of ability to implement the technique on conventional clinical MR scanners reliably.
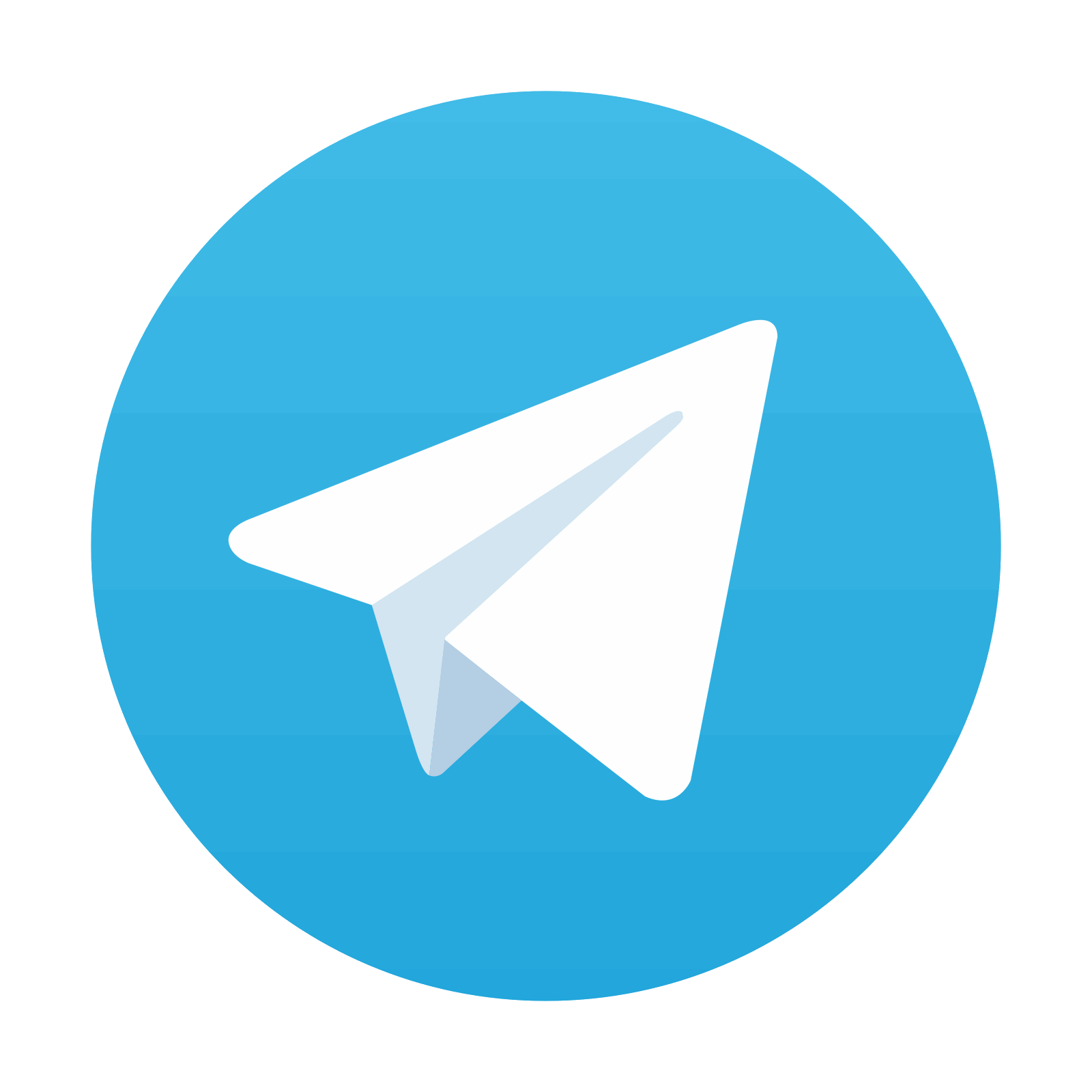
Stay updated, free articles. Join our Telegram channel

Full access? Get Clinical Tree
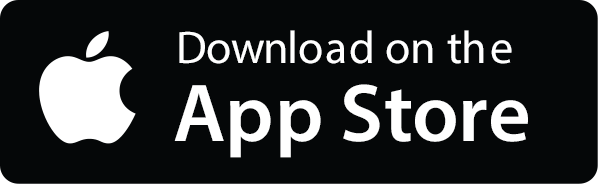
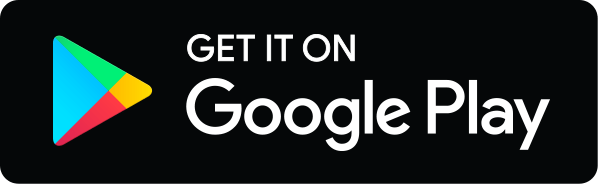