More imaging centers are incorporating diffusion-weighted imaging (DWI) into the clinical breast magnetic resonance (MR) examination, with the potential of increasing the specificity of breast MR imaging. This article reviews the role of DWI in the evaluation of breast lesions. The principles, technique, and protocol of DWI are discussed, including the importance of the chosen b value. The interpretation strategy, including quantitative and qualitative analysis is described. Clinical applications of breast DWI are evaluated, such as distinguishing malignant from benign lesions, tumor characterization and monitoring treatment planning, and potential pitfalls such as false-negative and false-positive findings are discussed.
Key points
- •
Magnetic resonance (MR) imaging is becoming a first-line radiological modality in the management of breast cancer.
- •
The main advantages of breast MR imaging are lack of ionizing radiation, high sensitivity, and a high negative predictive value.
- •
Diffusion-weighted MR imaging has been introduced for cancer imaging, and it can be used for differentiating benign and malignant breast lesions.
- •
Diffusion-weighted imaging can help to correctly classify as benign many false-positive cases seen on dynamic contrast-enhanced (DCE)-MR imaging, such as fibroadenomas.
- •
Malignant lesions show restricted diffusion, with high signal intensity on DWI and low apparent diffusion coefficient (ADC) values, mostly related to increased cellularity and decreased extracellular space.
- •
Mucinous cancer shows a very high ADC value because of low tumor cell density and a large amount of mucin around the tumor cells.
- •
The choice of b values directly affects quantitative analysis of the ADC, because the signal intensity at DWI is influenced by the b value.
- •
DWI may be a viable noncontrast alternative method for breast MR imaging screening.
- •
DWI may be able to offer earlier and more precise information on response to neoadjuvant treatment than DCE-MR imaging.
Introduction
Breast magnetic resonance (MR) imaging is the most sensitive imaging modality for the detection of breast cancer, and it has become a valuable breast screening technique in select patient populations. Standard breast MR imaging protocols incorporate evaluation by dynamic contrast-enhanced (DCE) MR imaging, which shows tissue vascularity and vascular permeability. Alterations in tissue vascular properties are characteristic to breast malignancies, enabling their detection by DCE-MR imaging. Although DCE-MR imaging has extremely high sensitivity in the diagnosis of breast cancer, as high as 89% to 100% for invasive cancers, the specificity is moderate (ranging widely from 21% to 100% across 44 studies, with an average of 72%).
Breast MR imaging is an area of intense research, and over the past decade, new MR techniques and interpretation strategies have been developed to increase its specificity and positive predictive value (PPV). Recommended techniques for image acquisition include high spatial and temporal resolution for careful assessment of both morphologic and kinetic features of lesions. In recent publications, using more current approaches, the reported increased specificities ranged between 67% and 92%.
However, despite improvements in DCE-MR imaging acquisition techniques, overlap in morphologic characteristics and kinetic features of some malignant and benign lesions still causes improper classifications. MR imaging false-positive results may be influenced by patient factors such as phase of menstrual cycle, hormone replacement therapy, and patient age. In addition, false-positive results may occur with lesions that share MR features of breast malignancy, such as papilloma, proliferative disease, and fibroadenoma. Thus, there is interest in studying new techniques that can be implemented into the standard breast MR imaging practice to reduce false-positive results and increase specificity, and consequently reduce morbidity, and economic and psychosocial costs associated with unnecessary biopsies.
Diffusion-weighted imaging (DWI) is one such MR imaging technique that is showing promise for improving diagnostic accuracy as an adjunct to DCE-MR imaging. DWI is a short scan available on most commercial MR scanners that does not require any exogenous contrast and can be added to breast MR imaging examinations to provide additional complementary information on tissue microstructural properties. Recent MR imaging technological advances, including echo planar imaging (EPI), high-amplitude gradients, multichannel coils, and parallel imaging, have been instrumental in extending the use and applications of DWI outside the brain. DWI provides a quantitative evaluation, and studies have shown that the apparent diffusion coefficient (ADC) values derived from DWI can facilitate in differentiating benign and malignant breast tumors as well as identifying early response in tumors undergoing preoperative treatment. Furthermore, DWI may provide a useful alternative to gadolinium-enhanced sequences for MR imaging evaluation of patients at risk for nephrogenic systemic fibrosis.
Introduction
Breast magnetic resonance (MR) imaging is the most sensitive imaging modality for the detection of breast cancer, and it has become a valuable breast screening technique in select patient populations. Standard breast MR imaging protocols incorporate evaluation by dynamic contrast-enhanced (DCE) MR imaging, which shows tissue vascularity and vascular permeability. Alterations in tissue vascular properties are characteristic to breast malignancies, enabling their detection by DCE-MR imaging. Although DCE-MR imaging has extremely high sensitivity in the diagnosis of breast cancer, as high as 89% to 100% for invasive cancers, the specificity is moderate (ranging widely from 21% to 100% across 44 studies, with an average of 72%).
Breast MR imaging is an area of intense research, and over the past decade, new MR techniques and interpretation strategies have been developed to increase its specificity and positive predictive value (PPV). Recommended techniques for image acquisition include high spatial and temporal resolution for careful assessment of both morphologic and kinetic features of lesions. In recent publications, using more current approaches, the reported increased specificities ranged between 67% and 92%.
However, despite improvements in DCE-MR imaging acquisition techniques, overlap in morphologic characteristics and kinetic features of some malignant and benign lesions still causes improper classifications. MR imaging false-positive results may be influenced by patient factors such as phase of menstrual cycle, hormone replacement therapy, and patient age. In addition, false-positive results may occur with lesions that share MR features of breast malignancy, such as papilloma, proliferative disease, and fibroadenoma. Thus, there is interest in studying new techniques that can be implemented into the standard breast MR imaging practice to reduce false-positive results and increase specificity, and consequently reduce morbidity, and economic and psychosocial costs associated with unnecessary biopsies.
Diffusion-weighted imaging (DWI) is one such MR imaging technique that is showing promise for improving diagnostic accuracy as an adjunct to DCE-MR imaging. DWI is a short scan available on most commercial MR scanners that does not require any exogenous contrast and can be added to breast MR imaging examinations to provide additional complementary information on tissue microstructural properties. Recent MR imaging technological advances, including echo planar imaging (EPI), high-amplitude gradients, multichannel coils, and parallel imaging, have been instrumental in extending the use and applications of DWI outside the brain. DWI provides a quantitative evaluation, and studies have shown that the apparent diffusion coefficient (ADC) values derived from DWI can facilitate in differentiating benign and malignant breast tumors as well as identifying early response in tumors undergoing preoperative treatment. Furthermore, DWI may provide a useful alternative to gadolinium-enhanced sequences for MR imaging evaluation of patients at risk for nephrogenic systemic fibrosis.
DWI
Principles of DWI
DWI is a noninvasive technique that measures the random motion of free water protons (Brownian motion) and characterizes different tissue properties from conventional MR parameters, such as T1 and T2 relaxation. In contrast to freely diffusing water, the movement of water molecules in biological tissues is restricted by interactions with cell membranes and macromolecules.
In vivo, the degree to which water diffusion is restricted is inversely proportional to the tissue cellularity and the cell membrane integrity. In tissues with a high cell density and intact cell membranes (such as tumor), diffusion is more restricted because the lipophilic cell membranes act as barriers to motion of water molecules. Conversely, in tissues with low cellularity or compromised cellular membranes, water diffusion is less restricted due to the larger extracellular space and ability of diffusing water molecules to move freely across cell membranes.
DWI Acquisition
Stejskal and Tanner described an experimental quantification of water diffusion in vivo that is now the basis of many DWI sequences in clinical use. Their approach was to apply a symmetric pair of diffusion-sensitizing (bi-polar) gradients around the 180° refocusing pulse of a standard T2-weighted spin-echo sequence, thereby attenuating the resulting signal in proportion to water movement. Diffusion can be expressed as the following monoexponential equation:
SDW = SSE ∙ exp (-b ∙ ADC), with b = γ 2 G 2 δ 2 (Δ – δ/3), where SDW is the attenuated spin-echo signal, SSE is the full T2-weighted spin-echo signal without diffusion attenuation, ADC is the apparent diffusion coefficient (mm 2 /s), b is the diffusion sensitization factor (s/mm 2 ), γ is the proton gyromagnetic ratio, G is the gradient strength, δ is the duration of G, and Δ is the time delay between the leading edges of the 2 diffusion-sensitizing gradients. The sequence generates 2 sets of images: the first set, in which the diffusion gradients are turned off (SSE; T2-weighted images), and the second set, with the diffusion gradients turned on (SDW; index diffusion-weighted (DW) images).
The faster the water molecules diffuse, the greater the attenuation and the weaker the corresponding signal intensity (SDW). Thus, signal intensity is usually higher in a region with restricted diffusion, such as tumor, than in a region with fast diffusion, such as free extracellular water in normal breast tissue ( Fig. 1 ). However, visual assessment of index DW images (SDW) is complicated by the fact signal intensity depends on both water diffusion and the T2 relaxation time. Thus, tissues with long T2 relaxation times, such as a fluid-filled cyst, may retain significant signal on DWI and be misinterpreted as having restricted diffusion. This phenomenon is known as the T2 shine-through effect ( Fig. 2 ). However, in this case, the conventional images are typically very clear, showing well-defined nonenhancing T2 bright lesions. The greater the strength of the diffusion sensitization (b value), the lower the impact of the T2 effect on the DW images.
DW images without T2 weighted information can be obtained by creating the exponential image, which is the ratio of the DW image divided by the corresponding unweighted reference image (SDW/SSE) at the same slice position ( Fig. 3 A, B ). Also, the quantitative ADC map can overcome the effects of T2 shine-through. ADC is calculated by:
ADC = −ln(SDW/SSE)/b, for each pixel of the image and is shown as a parametric map, performed on the scanner or work station (see Fig. 3 C). Simple cysts can easily be differentiated from cellular tumors on ADC maps based on differences in water diffusion rates ( Fig. 4 ).
Technical Aspects of DWI
There are challenges to obtaining good-quality breast DWI images and accurate ADC measures. Typical EPI-based DWI sequences can result in image distortion during data acquisition, because of eddy currents, susceptibility effects, and ghosting artifacts. These issues are particularly challenging for breast imaging because of the off-isocenter imaging and significant fat content in the breast. To reduce artifacts and optimize data quality, good shimming and suppression of lipid signal are essential. The optimal technique for fat suppression (eg, spectral attenuated inversion recovery [SPAIR], short-tau inversion recovery [STIR]) may vary between scanners. Parallel imaging techniques also help to reduce susceptibility-based distortions and ghosting artifacts through shortened imaging times. Protocols also must be optimized for adequate signal-to-noise ratio (SNR) by balancing spatial resolution and appropriate diffusion sensitizations or b values.
Influence of b value
The choice of b values may vary according to the protocol and directly affects quantitative analysis of the ADC, because the signal intensity at DWI is influenced by the b value. At lower b values, T2-weighted signal is emphasized (T2 shine-through effect). Lower b values also generate higher ADC values in vivo, because of the contribution of intravoxel incoherent motion effects other than diffusion. Microperfusion, defined as microcirculation within the capillary network, is an important potential influence on diffusion measures. In vivo, the measured ADC value includes the factors of both water diffusion and perfusion effect; hence the term apparent diffusion coefficient, and can be expressed in the equation:
ADC value ≈ D + (f/b), where D is the diffusion coefficient and f is the perfusion factor.
At lower b values (b <400 s/mm 2 for breast tissue), the DWI signal is more influenced by attenuations caused by perfusion or flow, thus increasing the net ADC value. Conversely, higher b values emphasize the contribution from the diffusion coefficient alone and can improve contrast resolution between various diseases and normal breast tissue ( Fig. 5 ). Therefore, high b values (b >500 s/mm 2 ) are recommended for breast DWI.
However, the optimal maximum b value for breast imaging has not yet been established and there is no consensus. The b value has varied between 500 and 1500 s/mm 2 in the literature.
For lesion conspicuity and detection purposes, a very high b value may be preferred. Kuroki and Nasu reported that optimal contrast between cancer and normal mammary tissue was achieved at a b value of 1000 s/mm 2 . However, the use of higher b values may reduce sensitivity for the evaluation of small lesions, such as ductal carcinoma in situ (DCIS), because of more rapid signal decay (ie, lower SNR).
For differentiation between benign and malignant lesions, choice of b value may be less important. Several studies comparing DWI acquired at different b values showed that the mean ADC values of malignant lesions were in general lower than those of benign lesions and normal breast tissue and that diagnostic accuracy was not significantly increased by using higher b values. However, specific optimal ADC cutoffs for lesion characterization are directly influenced by the choice of b value. Thus, the same ADC threshold or cutoff for lesions evaluated with DWI obtained using b values of 1500 s/mm 2 would not be used for lesions evaluated with DWI obtained using b values of 800 s/mm 2 .
In theory, DWI acquisition using multiple b values should provide a more accurate sampling of signal decay for calculation of ADC. However, studies of multiple b value acquisitions in the breast found no improvement in the ability to discriminate benign and malignant lesions over standard 2 b value acquisitions. Given the time constraints of clinical practice, ADC calculation using 2 b values may be considered reasonable and acceptable.
Although standard DW sequences use b = 0 s/mm 2 as the reference for calculating ADC, a nonzero b value reference image may be preferable in vivo to obtain ADC measures free from perfusion and flow contamination. However, a diagnostic advantage was not clearly shown in one study investigating this approach.
Determination of optimal b values for breast imaging and lesion characterization remain areas of active research.
DWI Interpretation
The principle that underlies in vivo DWI is that the motion of the water molecules in the extracellular fluid enables the acquisition of an image that reflects both histologic structure and cellularity. Factors responsible for restriction of movement of extracellular water molecules include high cellularity, intracellular edema, swelling, and extracellular medium with high viscosity (which is observed in the case of abscess and hematoma).
The pathogenesis of the restriction of diffusion in breast cancer is cell proliferation. Tumor growth causes higher cellularity, tissue disorganization, and increased tortuosity of the extracellular space, which result in disruption or restriction of motion of the extracellular water within the tissue. These changes in the diffusion of water result in changes in the signal intensity on the DWI. An inverse relationship between tumor cellularity and ADC measurements has been previously reported. In breast lesions ranging from high cellularity (tumors) to low cellularity (cysts), Hatakenaka and colleagues found that the greater the number of cells per area, the lower the ADC value.
DWI can be interpreted both qualitatively and quantitatively. Qualitatively, areas of restricted diffusion appear as higher signal intensity on the index DW images and lower signal intensity on the ADC map, which may be enhanced using color representation. Quantitative ADC analysis is typically performed by drawing a region of interest (ROI) on the ADC map and calculating the mean value for all pixels within the ROI. Tumor visibility is typically better on DCE-MR imaging because of the reduced spatial resolution of DWI. Therefore, lesions can be identified on the DCE-MR images and the ROI positioned in the lesion at the corresponding location on the DWI series and ADC map ( Fig. 6 ).
Diffusion interpretation must be taken in context with other imaging information to ensure an accurate diagnosis. In general, the clinical implication of ADC value depends on the tissue under investigation; an anomalous increase in ADC can indicate increased edema, cystic changes, and necrosis, whereas an anomalous reduction in ADC might indicate bleeding (hematoma, bleeding cyst) ( Fig. 7 ), infection (abscess), or tumor. For example, the presence of blood or thick content in dilated ducts leads to restriction of diffusion, similar to ductal cancer. However, in the conventional images, ductal content can typically be identified with hyperintense signal on T1 and hypointense on T2 and no enhancement.
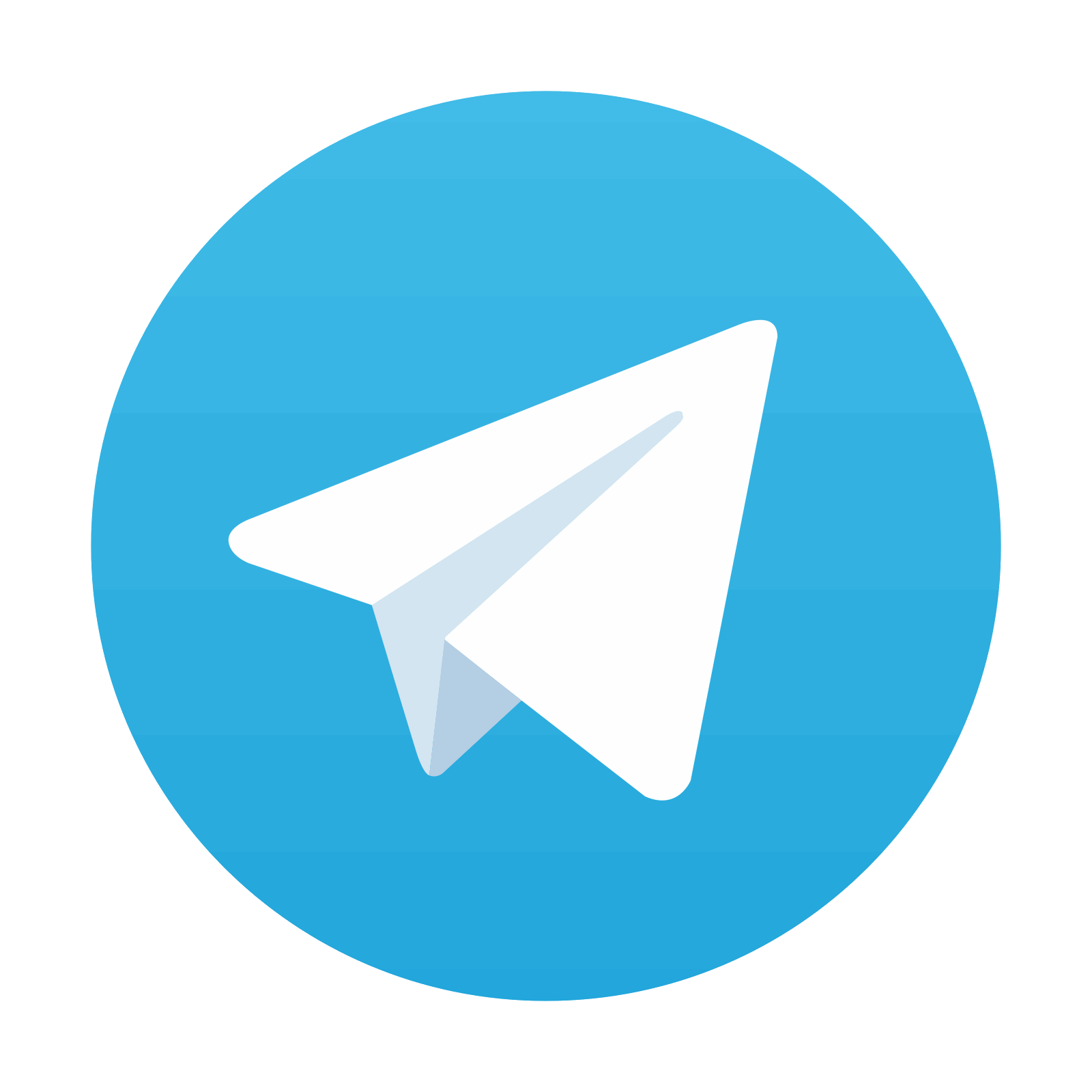
Stay updated, free articles. Join our Telegram channel

Full access? Get Clinical Tree
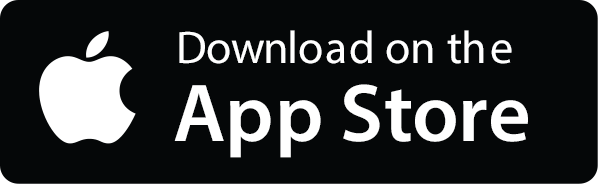
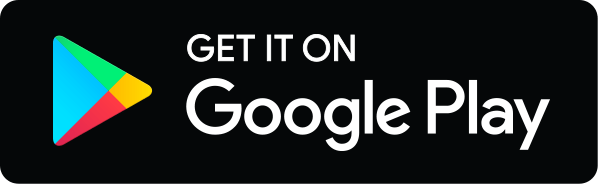
