Cardiac IVIM MRI
Intravoxel incoherent motion (IVIM) proposed by Le Bihan et al. in 1986 has been developing for several decades. It has been widely used to assess pathological characteristics of multiple organs, such as the brain, abdominal organs, and breasts, including animal experiments and clinical research. However, only a few studies have investigated cardiac IVIM.
Table 9.1 Summary of cardiac IVIM parameters in normal animals or humans
9.1 Implementation of Cardiac IVIM
The development of echo-planar imaging (EPI) made cardiac IVIM possible by greatly reducing the scan time. Formerly, Pop et al. [1] used a custom fast spin echo (FSE) sequence to scan the total heart, which took approximately 10 h for only one b value (500 s/mm2). Using an EPI sequence, currently one needs just 10 s to complete one slice of a cardiac IVIM scan with nine b values [2, 3]. Nowadays, a spin-echo echo-planar imaging (SE-EPI) sequence is usually used in cardiac IVIM.
Diffusion-weighted imaging (DWI) is very sensitive to motion, including respiratory, cardiac, and gastrointestinal peristaltic movements, which can induce signal loss at a low signal intensity and reduce the signal-to-noise ratio (SNR). Bulk motion is the most challenging motion type for cardiac IVIM. Therefore, strategies are being developed to minimize physiological motion during the acquisition.
The first study of microcirculation in canine heart using IVIM was performed by Callot et al. in 2003 [4]. To remove the influence of cardiac motion, they used a correction long-axis stimulated echo acquisition mode (STEAM) sequence. Encoding and rewinding gradients of the STEAM sequence were placed at exactly the same point of the cardiac cycle, to measure water movement between the same end-diastolic points of two consecutive heartbeats when the position and shape of the heart were the same. STEAM could shorten the acquisition time, enhance spatial resolution and temporal resolution, and improve image quality.
Implementing motion-compensated (MOCO) diffusion-encoding gradient waveforms can mitigate sensitivity to bulk motion. Gamper et al. [5] used flow-compensated diffusion gradients in combination with a reduced field of view to increase the SNR without using the STEAM technique. Aliotta et al. [6] recently demonstrated that the convex optimized diffusion encoding (CODE) DWI can reduce the echo time (TE) and enhance the SNR of the DWI map as well as compensate for bulk motion; they did this by comparing CODE DWI with the conventional MOCO diffusion spin-echo encoding by nulling first- and/or second-order gradient moments (M1, M1 + M2) to mitigate bulk motion [6].
Rapacchi et al. [10] proposed a method to minimize motion- induced signal loss by principal component analysis filtering and temporal maximum intensity projection (PCATMIP) techniques. The PCATMIP algorithm was used with a set of 10 repetitions (acquired with time shifts of 10 ms) for each b value to capture the moment when motion-induced signal loss was minimized. Studies demonstrated that the PCATMIP postprocessing was beneficial for signal recovery, increasing the SNR. This method was also shown to be robust enough to be independent of the RR irregularity and might therefore be the solution of choice for patients with arrhythmic cardiac cycles [2,8–10]. In addition, the IVIM parameters had a good reproducibility with PCATMIP both in-between free breathing and in breath-hold acquisitions [8].
There is a certain degree of water exchange between the intra- and extravascular compartments. Lemke et al. [7] proposed a refinement of the DWI signal model that accounts for the T1 and T2 relaxation of blood and tissue as well as the TE and repetition time (TR) sequence parameters:
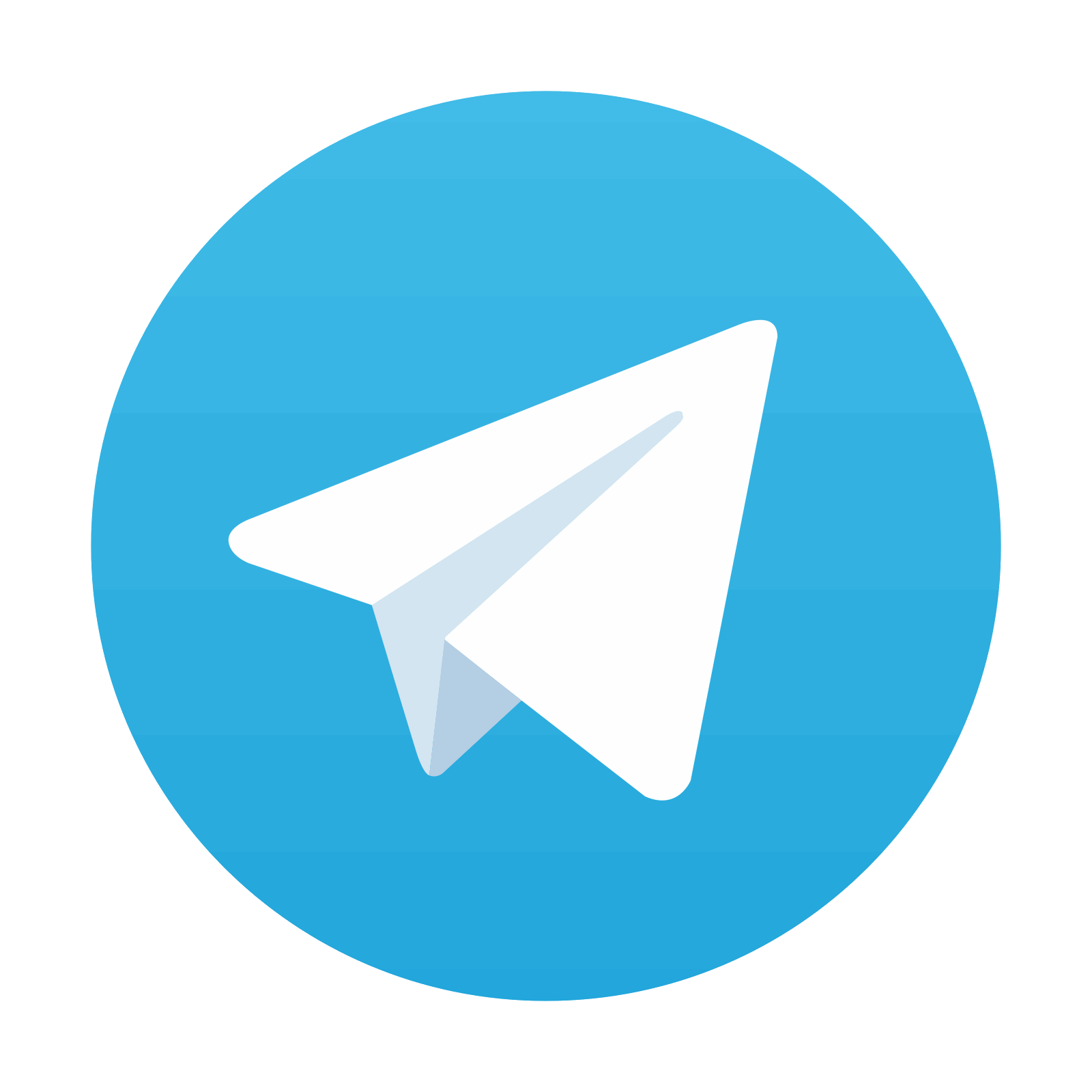
Stay updated, free articles. Join our Telegram channel

Full access? Get Clinical Tree
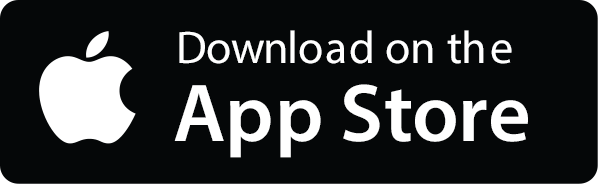
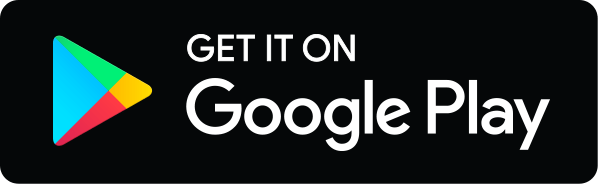