Avinash U Sonawane, V. Anuradha, Anand Pinjarkar X-ray radiation for diagnosis is widely used application of ionizing radiation in medicine, in terms of both the number of existing institutes/facilities and the number of persons who are exposed for clinical investigations. As per the global data published by United Nations Scientific Committee on the Effects of Atomic Radiation (UNSCEAR), 2020/21, about 4.2 billion medical radiological examinations were performed annually, leading to individual annual average dose of 0.57 mSv. Medical X-ray exposures account for 99% of the contribution from all artificial radiation sources. This is not surprising as X-ray-based diagnostic radiology equipment encompass a wide gamut of practices ranging from the general-purpose radiography (fixed or mobile), fluoroscopy, computed tomography (CT), cath lab, C-arm, intraoral periapical X-ray, orthopantomography and cone beam CT used in dental departments and other diagnostic equipment such as mammography and bone mineral densitometer (or dual-energy X-ray absorptiometry). All these equipment are internationally termed as “medical radiological equipment”. The CT scan equipment, in particular, has become a ubiquitous diagnostic modality in a hospital, owing to its versatility and practicality. It is also commonly used in emergency departments for accident victims for quick triage. CT is also useful for cancer treatment planning with use of positron emission tomography–CT for detailed information about cancer and CT simulation for delineating target volume and sensitive organs for radiation therapy. In CT scans, series of X-ray images are taken around the body, and combining these images by computer processing creates cross-sectional images. CT is based on variable absorption of X-rays by different tissues, and the principle of working is similar to radiography or fluoroscopy equipment. However, the imaging in CT technology is very different from that of radiography or fluoroscopy. A CT imaging system produces cross-sectional images or “slices” of anatomy, which are then effectively used for accurate diagnosis of disease. CT systems are capable of “spiral” or “helical” scanning as well as conventional “axial” or “sequential” scanning. Many CT systems are capable of imaging multiple slices simultaneously, which allows imaging of large volumes of anatomy in relatively less time. Over the years, there has been great advancements in the CT technology, such as improvements in speed and image quality. The image reconstruction techniques have contributed significantly in reduction of radiation dose to the patient as compared with erstwhile CT scanners. With all its undeniable benefits in terms of timely diagnosis, the CT scan equipment stands as the one diagnostic modality with the potential to deliver substantial radiation exposures to the patient. The scattered radiation from the patient’s body could expose the radiological medical practitioners (RMPs)1/medical radiation technologists (MRTs)/other medical personnel (e.g. anesthetists) working in close proximity of the equipment. The radiation could also expose the general public in the vicinity of equipment room. X-rays are ionizing radiation, which deposits its energy in the tissues and bones in the human body. For the patient undergoing diagnosis, the X-rays emitted directly from the equipment (termed as primary radiation) result in differential absorption and are reflected in an X-ray image. There are two types of adverse health effects of ionizing radiation: the deterministic effects and the stochastic effects. Deterministic effects relate to direct killing of cells by radiation resulting into damage to organism and include (at higher doses) skin erythema, epilation and ulceration/necrosis. Stochastic effects relate to genetic damage of cells and modifications in germ cells, leading to mutation resulting into carcinogenesis and hereditary effects. The probability of occurrence of stochastic effects increases with dose, while severity of deterministic effects increases with dose. The radiological risk associated with various types of radiology equipment varies significantly. At the low-risk end are dental exposures (excluding cone beam computed tomography) and bone densitometry studies. At the high-risk end are CT scans and image-guided interventional procedures where radiation injury may occur. As with CT and most other diagnostic equipment, there exists primarily the consequence of stochastic effects. Deterministic effects are not prevalent in this modalities. The exception is cath lab, wherein certain radiological procedures require continuous X-ray exposures to the affected parts of body for appropriate diagnosis/treatment. This has, in certain instances, resulted in development of radiogenic effects in patient, i.e. skin erythema, deep cell squamation, etc. There is a possibility that RMP/MRT and general public in the vicinity get exposed to the scattered radiation from the patient body, which is, however, substantially lesser than the primary radiation. In addition, there is a small amount of radiation leakage from the CT equipment, which contribute to the exposure of RMP/MRT and general public in the vicinity. The cohort of people receiving these exposures are different. Hence, these are classified as follows: All the aforementioned exposures should be kept minimum without compromising in acquiring the desired clinical information. For achieving this, the team of persons performing radiological procedure should be qualified, trained and competent. The following paragraph gives a brief primer of the radiological quantities and units used to explain radiation dose, which is important to be able to interpret and assess the extent of radiation protection to the three cohorts mentioned earlier. The values of tissue weighting factors recommended in International Commission on Radiation Protection (ICRP) Report No. 103 are given as follows: Source: http://www.icrp.org/publication.asp?id=ICRP%20Publication%20103. For example, if lung and brain are exposed to radiation separately and equivalent dose to the organs is 100 mSv each, then the effective dose is (100 mSv × 0.12) + (100 mSv × 0.01) = 13 mSv. Therefore, the risk of harmful effects from this radiation would be equal to 13 mSv dose delivered uniformly throughout the whole body. Atomic Energy Regulatory Board (AERB) is the national regulatory body in India, which exercises regulatory control over all the medical radiological equipment, from radiation safety view point. Accordingly, safety licence from AERB is mandatory for operations of any radiological equipment. Licence is issued under the provisions of the Atomic Energy (Radiation Protection) Rules, 2004, promulgated under the Atomic Energy Act, 1962. For issuance of licenses, AERB has deployed efficient and transparent web-based licensing system named as electronic Licensing of Radiation Applications (eLORA). AERB stipulates requirements to be met to ensure radiation safety in operation of X-ray equipment in its Safety Code entitled, “Radiation Safety in Manufacture, Supply and Use of Medical Diagnostic X-Ray Equipment”, AERB/RF/SC-3 (Rev-2) 2016. For obtaining AERB licence, the mandatory requirements are, appropriate layout and shielding of the X-ray room, qualified personnel, personnel monitoring, personnel protective equipment (such as protective barrier, aprons, gloves, thyroid shield, gonad shield, protective goggles). Also, the X-ray equipment shall be type approved (i.e. design approved) by AERB. Procuring the AERB-type approved equipment ensures built-in safety features and intended operational performance as per the prescribed quality assurance protocols. AERB has stipulated effective dose limits to the radiation workers and general public as follows: Medical exposures are delivered to individuals (patients) undergoing diagnostic (X-ray or nuclear medicine) examinations, radiological interventional procedures or radiation therapy. The exposure is intentional and for the direct benefit of the patient. It is important to note that specific dose limits are not applicable to medical exposures (i.e. radiation exposure to patients). However, they are governed by principles of radiation protection, i.e. (1) justification, (2) optimization and (3) dose reference levels. International Commission on Radiation Protection (ICRP) in its Report No. 103 gives emphasis on the justification of the medical exposure and on the optimization of protection. ICRP recommends principle of justification at three levels:
1.15: Clinical application of radiation dose optimization and image optimization in radiology
Introduction
Radiation protection
Terms explaining radiation dose
Tissue
Tissue Weighting Factor
Bone marrow (red)
0.12
Breast
0.12
Colon
0.12
Stomach
0.12
Lung
0.12
Gonads
0.08
Thyroid
0.04
Liver
0.04
Oesophagus
0.04
Bladder
0.04
Brain
0.01
Bone surface
0.01
Salivary glands
0.01
Skin
0.01
Remainder tissues: adrenals, gall bladder, extrathoracic region, kidney, heart, lymphatic nodes, muscles, oral mucosa, pancreas, small intestine, spleen, thymus, prostate (in male), uterus/cervix (in female)
0.12
Total
1.00
Regulatory body in India governing radiation safety
Medical exposures
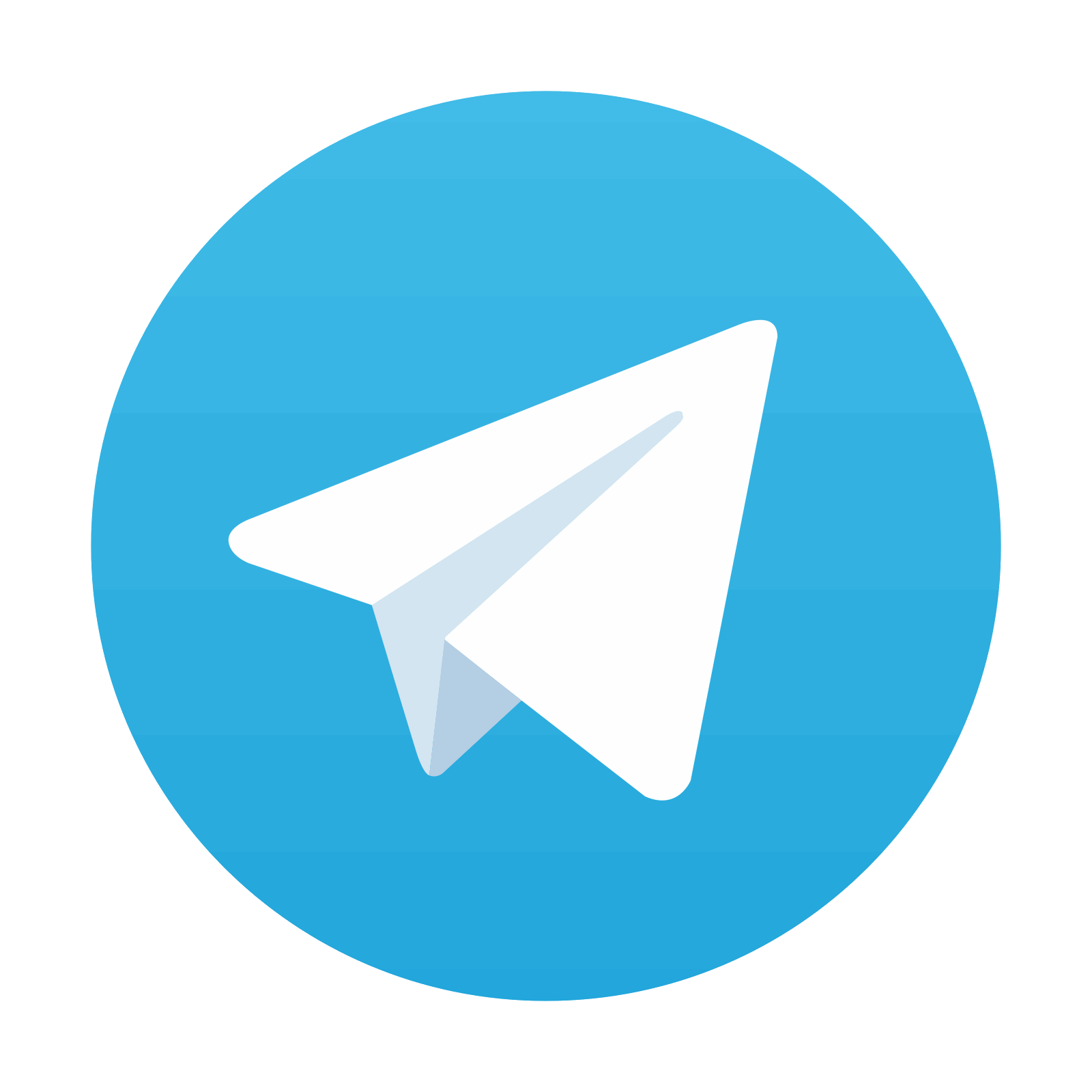
Stay updated, free articles. Join our Telegram channel

Full access? Get Clinical Tree
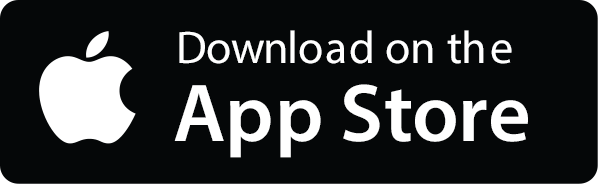
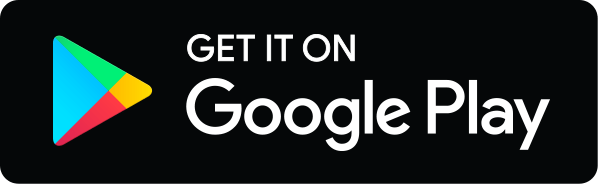