Congenital
1. Tethered cord syndromes
2. Spina bifida
3. Dural ectasia
4. Spinal stenosis
Metabolic and inflammatory
1. Diabetic peripheral neuropathy
2. Hyperthyroidism, hypothyroidism
3. Ankylosing spondylitis
4. Paget’s disease
5. Sarcoidosis
6. Arachnoiditis
Traumatic
1. Traumatic disc disruption
2. Complex regional pain syndrome (CRPS) types I and II
3. Postsurgical nerve entrapment:
(a) Post-laminotomy syndromes
(b) Post-carpal tunnel/tarsal tunnel release-related CRPS-II
(c) CRPS-II of the ulnar nerve postsurgical transposition
(d) Chronic piriformis syndrome (sciatic neuralgia)
(e) Brachial plexus avulsion
(f) Electrical injury
4. Traumatic amputation
Neoplastic
1. Chemotherapy-related peripheral neuropathy
2. Peripheral nervous system malignancies
3. Intraspinal malignancies
Infectious
1. Herpes zoster
2. Epidural abscess
3. HIV-related peripheral neuropathy
(a) Primary
(b) Secondary, related to antiretroviral chemotherapy
4. Lyme’s disease
In each of these scenarios, some pathological mechanism for peripheral or central nerve injury has created an ongoing, self-sustaining pain process. This involves processes imbedded within the central nervous system such as “central sensitization” and “windup,” which can be substantially influenced by normal sensory signals resulting in painful sensations, dysesthesias, and paresthesias. The involvement of the sympathetic nervous system in response to these phenomena adds another layer of complexity and pain pathology, as alterations in global and local sympathetic tone can amplify the pain experience.
Typical manifestations of neuropathic extremity pain include burning, throbbing, aching, and boring pains accompanied by allodynia, dysesthesias, hyperalgesia, and temperature or blood flow alterations. These can result in abnormalities of perspiration and piloerection, and episodic muscle cramping and twitching [1, 2], ipsilateral blockade of the sympathetic ganglia of the affected extremity (for neuropathic extremity pain), or bilateral blockade of the sympathetic ganglion of the affected intervertebral disc level (for neuropathic axial pain) will often result in pain relief and a normalization of vascular abnormalities. This includes enhanced blood flow to distal sensory neurons (with an increased sensitivity to light touch) and to muscle groups (with a relaxation of cramping and spasm). Those patients who respond well to sympathetic blockade tend to respond well to intraspinal neurostimulation, and many of these same features can be seen clinically.
Neuroanatomy and Neurostimulation
Nociceptive and neuropathic pain is carried to the central nervous system (CNS) from the periphery via sensory neurons, directly or indirectly. Beginning in small, microscopic (“unnamed”) nerves, sensory information is transmitted to larger nerves and then to spinal nerves. These sensory neurons are incorporated into spinal nerves directly into the CNS by way of the dorsal root ganglia (DRG) and the dorsal root entry zone (DREZ) and, from there, into the dorsal horn to be incorporated into elements of the central nervous system. Sensory neurons can also enter the CNS indirectly by way of the autonomic nervous system (sympathetic or parasympathetic elements). At the level of the DRG, the spinal nerves are separated into ventral motor and dorsal sensory components before entering the spinal cord. The majority of sensory neuronal systems enter the spinal cord via the dorsal root entry zone (DREZ) and are distributed within the dorsal horn to ascending pathways in the dorsal columns (ipsilateral) and tracts of the ventrolateral quadrants (ventral spinothalamic tract and lateral spinothalamic tract) in both ipsilateral and contralateral manners via numerous interneuronal synapses [3, 4].
It is extremely important to understand that different ascending tracts within the spinal cord carry qualitatively different aspects of sensory information; this can have a bearing on the appropriate targeting for intraspinal neurostimulation. The dorsal columns carry the sensations of vibration, touch, proprioception, and pain to the thalamus; the lateral spinothalamic tract (part of the ventrolateral quadrants) carries sensations of pain and temperature, while the ventral spinothalamic tract carries sensations of visceral pain and temperature. Because of the profound neuroplasticity often associated with neuropathic pain, it is entirely possible that different qualitative pain sensations can be modulated with neurostimulation along tracts not traditionally associated with neuropathic pain. If this were not the case, it might very well be that neurostimulation of the dorsal columns alone would have minimal effect on neuropathic pain [4].
Since neuropathic pain frequently incorporates elements of both touch and temperature, it is often necessary to provide neurostimulation to both the dorsal columns and the ventral and lateral spinothalamic tracts in order to achieve optimal therapy. However, neurostimulator leads must be placed dorsally (e.g., dorsal columns and dorsal root entry zone), in order to avoid the motor stimulation that occurs with more ventral placements, and this substantially impedes access to the ventral and lateral spinothalamic tracts. The lateral spinothalamic tract is located too deeply within the spinal cord to be influenced directly by neurostimulation fields on the surface of the cord. The ventral spinothalamic tract is more superficial, and it can be influenced with neurostimulation. However, precise lead placement in this area can be quite difficult, dangerous, and complicated by motor recruitment. Thus, traditional dorsal column lead placement alone may miss some of the opportunities to adequately and more thoroughly affect the modulation of neuropathic sensory information traversing all three spinothalamic tracts.
Directly stimulating the DRG, or combining dorsal stimulation of the traversing and exiting spinal nerves (at the level of the neuroforamina), with/without DREZ stimulation, added to the more traditional approach of dorsal column stimulation (DCS), takes advantage of the neuroanatomy by incorporating additional spinal tracts in the total neuromodulation scheme of neuropathic pain. By appropriately targeting the DRG, individual spinal nerves, or the DREZ, electrical neuromodulation of fibers can be achieved before they are anatomically distributed and reorganized within the dorsal horn to the various spinothalamic tracts. This can greatly augment the efficacy of neuromodulation achieved with DCS alone and can be referred to as multi–target stimulation.
It is thus extremely important for the implanter to have a thorough understanding of cranial, spinal, and peripheral neuroanatomy to appropriately take advantage of the wide variety of neuronal targets that are available for efficacious neurostimulation.
Lower amplitudes of stimulation than that needed for the dorsal columns are required for stimulation of the nerve roots and the DRG, because the CSF layer is quite thin at these locations. Thus, contact separation from the targeted tissues is quite small, and significantly less energy is required for activating the nerve fibers at these locations [5, 6]. The amplitudes involved to generate a “therapeutic window” for pleasant and efficacious neurostimulation in the general region of nerve roots and the DRG are extremely small. (This phenomenon is present in the periphery as well, where the distance of separation from the electrical contact to the targeted nerve, and local tissue electrical impedance, determines the amount of energy (amplitude of stimulation) required to obtain clinically relevant neuromodulation.) With the presence of a disease state creating neuropathic pain, these same neuronal pathways may actually be much more sensitive to stimulation as a result of “primary hypersensitivity”. Thus, these exquisitely sensitive neuronal pathways may be recruited with even lower levels of amplitude than their adjacent, somatotopically arranged neighbors, and thereby provide more focal targeting of specific anatomical areas. This could have significant clinical ramifications for selective neurostimulation of peripheral nerves, spinal nerves, the DRG, traversing nerves, nerve rootlets and the DREZ.
The optimal contact locations that enable field exposure to a sufficiently wide range of sensory fibers suitable for stimulation tend to cluster in the dorsal aspects of the spinal canal and are the preferred region for therapeutic neurostimulation. To avoid motor stimulation when nerve root or ganglion stimulation is desired, the individual lead electrodes need to be placed quite dorsal to the targeted structure. At this location, the therapeutic effects of both sensory and subsensory stimulation can be obtained with greater precision and success.
Electrical neurostimulation is a surface phenomenon, and the depth of penetration into neural tissue is remarkably small (less than 0.25–0.5 mm) compared to the spread of the stimulating electrical field across the surface of the targeted structure [6]. The more superficial the individual neuron is to the surface of the neural structure being targeted, the greater will be its sensitivity to electrical stimulation. In view of this, it is important to take advantage of the neuroanatomical differentiation between motor and sensory fibers in the spinal nerve roots in the area of the dorsal root ganglion and between the DREZ and the dorsal columns in the cord itself. Precise anatomic placement of the electrical field is essential, and the tools to be used include amplitude, frequency, pulse width, and contact configuration of cathodes and anodes. Appropriate attention to the use of all of these tools is necessary to create a therapeutic electrical field.
Further features of the neuroanatomy of the dorsal columns also bear consideration in the clinical application of electrical fields. They include the concepts of somatotopic organization, surface area, fiber types, sizes, and speeds of conduction, and thickness of the dorsal CSF layer.
Somatotopic Organization
There is considerable somatotopic organization within the dorsal columns [7], and fibers consistent with a particular dermatome tend to ascend within an organized scheme. After entrance into the dorsal horn, fibers synapse with interneurons and are distributed to the various ascending tracts. Within the dorsal columns, fibers associated with individual dermatomes appear in the ventral areas, deep within the gray matter of the fasciculus gracilis (thoracic, lumbar, and sacral spine) or the more lateral fasciculus cuneatus (in the cervical spine). From there, these fibers ascend toward the thalamus and migrate dorsally within the lateral aspects of the fasciculus to gradually develop a “dorsal exposure at the lateral border with the DREZ (Fig. 43.1)” [7].
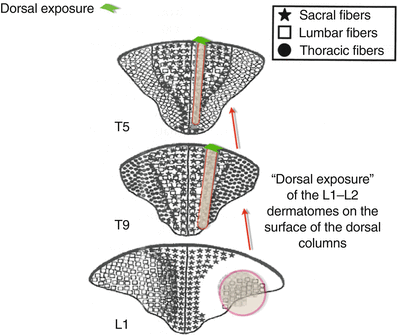
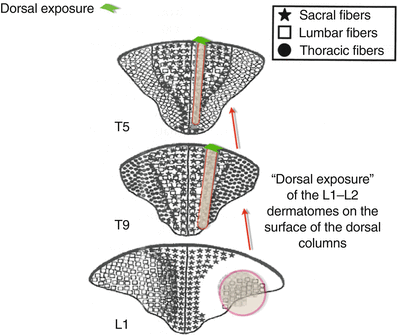
Fig. 43.1
Somatotopic representation of the dorsal columns. Note that after appearing in the deep tissues of the dorsal columns at lower spinal levels, the L1 and L2 dermatomes gradually develop a “dorsal exposure” at more cephalad spinal levels. This creates a superficial “rim” of somatotopic tissue for those specific dermatomes, which can respond to applied electrical fields. This rim of “dorsal exposure” migrates medially from the lateral edges of the dorsal columns along the borders of the dorsal root entry zone DREZ. They then “migrate” medially, as they ascend toward the foramen magnum, becoming thinner as the fibers decrease in size (Adapted from Smith and Deacon [7], with permission)
The dorsal exposure on the surface of the dorsal columns is extremely important, as the electrical field does not penetrate greater than 0.25–0.50 mm within the gray matter of the cord. Thus, until a particular dermatome has a “dorsal exposure,” it cannot be affected by epidural neurostimulation. The electrical impedance of the CSF is so much greater than the conductivity of the gray matter of the cord that electrical current will more readily be shunted (following the “path of least resistance”) into the CSF, away from penetration of gray matter. This causes the electrical field to extend into the area of the dorsal roots (producing “flank” stimulation), and motor activation can occur as stimulation amplitude is further increased.
As shown in Fig. 43.2, this somatotopic organization of dermatomes across the surface of the dorsal columns creates a “grain,” much like what one would see within a plank of wood. Clearly, the geometry of the electrical field needs to be parallel to the “grain” to induce the greatest electrical influence over the parallel fibers ascending to the thalamus. Interestingly enough, the majority of neurostimulator leads have contacts with the long axis parallel to the “grain” of the dorsal columns.
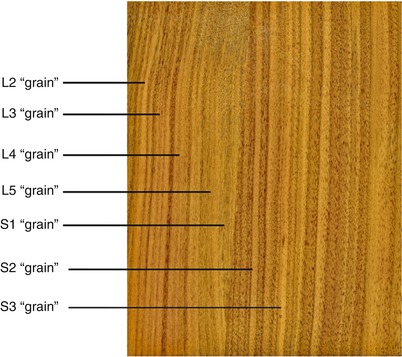
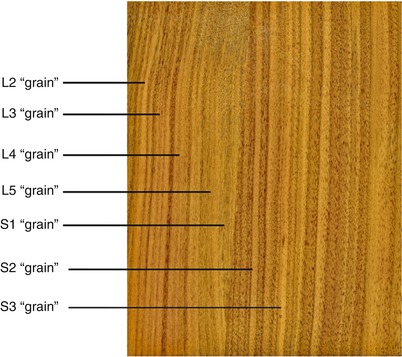
Fig. 43.2
Somatotopic representation of the dorsal columns. The striations of the dermatomes across the surface of the dorsal columns, moving lateral to medial, may well be a bit similar to the grain of wood as seen in this image of a walnut panel. Very little in nature is perfectly parallel, and individual variation between subjects appears to be the “norm.” This concept helps to account for the high degree of variation between patients in programming neurostimulator leads over the Dorsal Columns, the DREZ, the DRG and even in the periphery
This somatotopic organization in the dorsal columns also helps to explain the ability to recruit fibers to create therapeutic paresthesias in the lower extremities from distal sites of stimulation such as the cervical spine (fasciculus gracilis). This is demonstrated by the ground-breaking work by Barolat et al. [8], shown in Fig. 43.3.
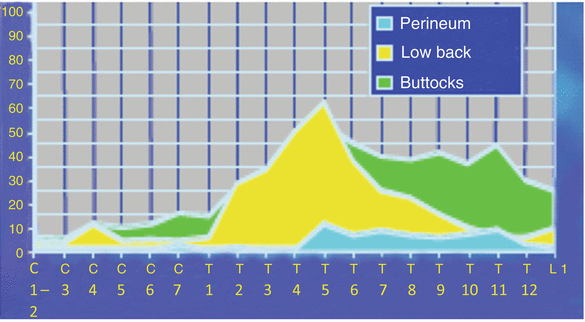
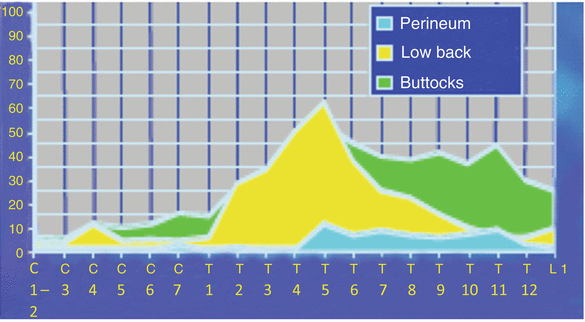
Fig. 43.3
Distribution of paresthesia from the dorsal columns. The ability to recruit stimulation of the lower extremities from cervical levels is well demonstrated in these figures. Barolat et al. noted that roughly 30 % of their subjects could obtain foot stimulation at C7 and nearly 60 % of their subjects could obtain stimulation of the low back at T5 under the right conditions (From Barolat et al. [8], with permission)
Somatotopic sensory organization is seen at all levels in the hierarchy of the nervous system, from the homunculus of the sensory cortex [9] to the ascending tracts in the cord [7], to the DRG [10–12], and within the peripheral nerves [13, 14]. Slipman et al. [15] carefully demonstrated with highly selective, fluoroscopically guided neural stimulation of cervical dorsal root ganglia the wide range of overlapping dermatomal stimulation associated with each DRG. This is a consequence of sensory input from multiple sensory nerve roots into adjacent and nearby dorsal root ganglia and enables a significantly robust interpretation of the sensory environment by the CNS. Thus, the whole concept of “two-point discrimination” is really a subtle and somewhat complex integration of sensory stimuli within the thalamus and higher centers. Obviously, this phenomenon is present throughout the peripheral nervous system, and while the classic dermatome maps are helpful, they are not to be interpreted in absolute terms. While there does appear to be a somatotopic distribution of dermatomal fibers within the DRG at any given level, for neurostimulation to be truly “focal” to a specific anatomic location, it is necessary to be “local” [16]. A conceptual schema of this is shown in Fig. 43.4.
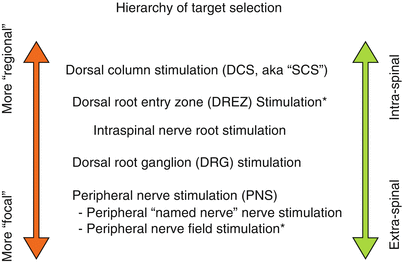
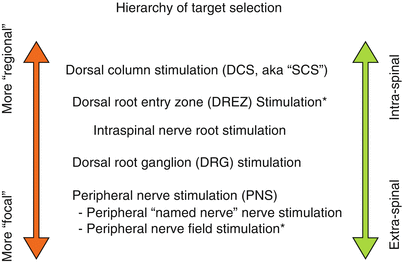
Fig. 43.4
Hierarchy of target selection in neurostimulation. As one moves from intraspinal to peripheral, the targets for neurostimulation change in complexity and specificity. There are always exceptions, especially with tremendous individual variation in patients. Two general exceptions are notable: (a) occasionally somewhat “focal” stimulation can be obtained with small electrical fields placed over rootlets at the DREZ and (b) peripheral nerve field stimulation (PNFS) is considerably broader than “named-nerve” peripheral nerve stimulation (PNS), as a result of the overlap of small branches from various separate “named-nerves”
Surface Area
The spinal cord varies in its cross-sectional area as it ascends from the conus medullaris to the foramen magnum. There are several remarkably important areas to be considered: the lumbosacral enlargement, the thoracic cord, the cervical enlargement, and the cervical cord. The lumbosacral and cervical enlargements are created by the immense number of additional neurons associated with motor, sensory, and autonomic functions within the lower and upper extremities, respectively, entering the spinal cord. This additional neuronal tissue enlarges the cross-sectional area of the cord over a portion of its distance, and thus, the total surface area of the dorsal columns is also increased. With this increase in the total surface area of the dorsal columns, a statistically greater opportunity exists for stimulating neurons within the dermatomal “grain.”
Of particular interest is the fact that within the thoracic cord, above the lumbosacral enlargement, the cross-sectional area diminishes and the ability to stimulate sacral elements almost diminishes considerably. It is reasonable to conclude that the width of the “grain” for the sacral dermatomes becomes quite small for most patients and is thus potentially quite difficult to recruit from surface stimulation. The opportunity to stimulate sacral and lower extremity dermatomes reappears at the cervical enlargement and continues some distance toward the foramen magnum [17]. It is almost as though fiber tracts “hidden” from an applied electrical field of stimulation within the fold of the dorsal median sulcus have blossomed back out onto the surface where they are again susceptible to stimulation. In many patients, there appears to be a slight contraction of the surface area of the dorsal columns within the cervical cord cephalad to the cervical enlargement, but in general, the surface area of the dorsal columns within the cervical cord is much greater than seen in the thoracic cord below the cervical enlargement. The cross-sectional geometry of the thoracic cord is virtually circular, whereas the cross-sectional geometry of the cervical cord resembles that of a lima bean, having a broader dorsal surface. With the cervical enlargement, the fasciculus cuneatus is formed lateral to the fasciculus gracilis, between which is the dorsal intermediate sulcus, and carries a “dorsal exposure” for dermatomes representing the upper extremities.
Fiber Types, Sizes, and Speeds of Conduction
There are different fiber types and sizes of fibers populating the dorsal columns. Both myelinated and unmyelinated fibers are found within the superficial layers of the dorsal columns, and they vary in size. Feirabend et al. [18] have shown by histological studies that Aβ-fibers having larger fiber diameters are found to recur more frequently along the lateral edges of the dorsal columns and near the center. Roughly 85 % of all fibers in the superficial dorsal columns are smaller than 7 μm, and only 1 % is larger than 10 μm, but these larger fibers occur with much greater frequency in the more lateral portions of the dorsal columns [18]. Aβ-fibers from a laterally placed dermatomal “grain” become smaller and migrate medially as they ascend toward the thalamus.
Of course, not all of the fibers are of a uniform size, nor are all the fibers myelinated. This has important consequence in neurostimulation because smaller fibers require greater amplitudes of stimulation intensity to trigger a response than larger fibers. This is demonstrated by a typical strength-duration curve shown in Fig. 43.5. Longer pulse width values promote the activation of smaller diameter fibers relative to larger diameter fibers, as found in other neurostimulation applications [19]. Thus, in dorsal column stimulation, longer pulse widths tend to increase the number of fibers activated, recruiting more of the smaller fibers toward the midline in the dorsal columns, where more sacral fibers are to be found, and producing a “sacral shift” in the perceived stimulatory pattern (Fig. 43.6) [20].
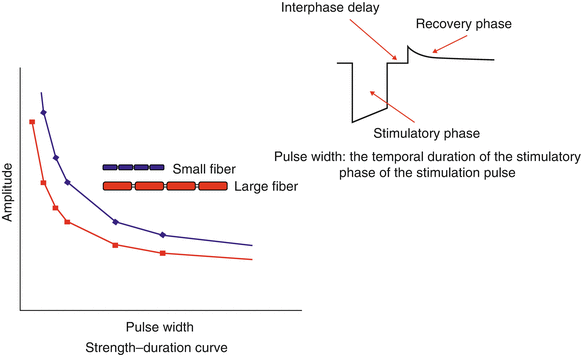
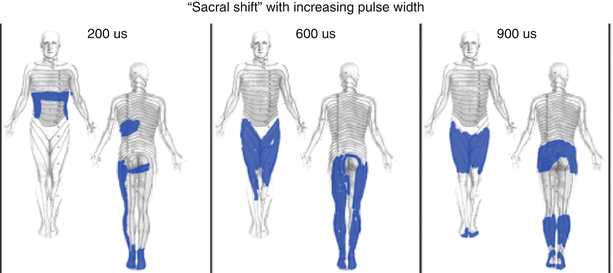
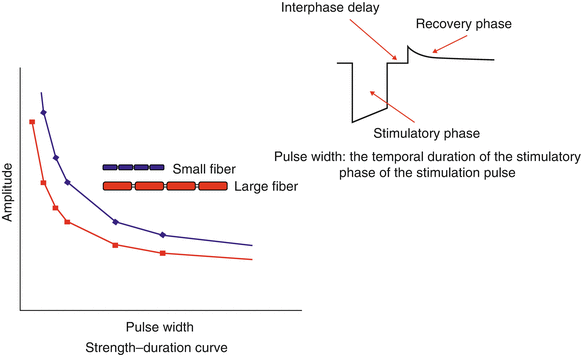
Fig. 43.5
Strength-duration curve. Larger diameter neuronal fibers are activated by applied electrical fields more easily than smaller fibers. Fiber sizes tend to be smaller nearer the midline of the dorsal columns. Increasing the pulse width of the stimulatory phase of the applied electrical field recruits more of these smaller diameter fibers and increases the area of perceived stimulation. Inset: A typical stimulation pulse for a neurostimulator contact
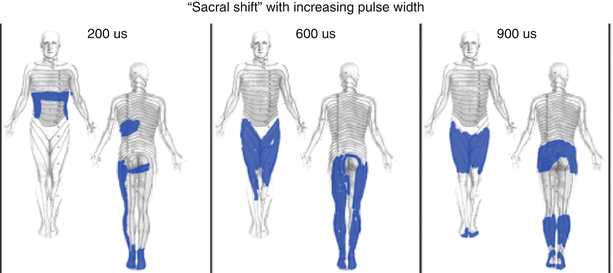
Fig. 43.6
Clinical example of the “sacral shift.” The clinical effect of increasing pulse width in the stimulation pulse is often to increase the incorporation of smaller, more medial fibers (typically in greater abundance in the midline of the dorsal columns: sacral dermatomes primarily) (From Yearwood et al. [20], with permission)
Also of note is the fact that the sensations created by neurostimulation of the dorsal columns traveling in myelinated fibers will reach the thalamus prior to those traveling in unmyelinated fibers. This is true whether the number of fibers recruited by any stimulatory pulse is sufficient to create a perceptible sensation within the thalamus, or remain as an imperceptible or “subliminal” influence upon the global sensory processing within the thalamus. Such a situation could perhaps block painful and erratic neuropathic signals from traversing the same individual neurons. With higher frequencies of stimulation, a greater number of fibers could potentially be slowly driven into a relative refractory phase, effectively rendering these fibers unavailable for pathologic signal transmission.
Finally, the absolute and relative refractory periods of the targeted neurons are in the time range of milliseconds, whereas stimulation duration and recovery phases of a typical neurostimulation system are in the time range of microseconds. This means that a typical neurostimulation system can deliver a number of stimulatory pulses of different contact configurations much more quickly than the CNS can process the individual signals. The typical speed of cinema is roughly 24 frames/s. This enables the CNS to appreciate perceptions of smooth motions from rapidly displayed individual “still-shot” frames. Thus, it is not surprising that the neurostimulation systems presently available are able to provide considerable sophistication in perceived and subliminal signals to interrupt the interpretation and CNS response of painful stimuli resulting from neuropathic processes. A typical rate of 40 stimulatory pulses/s is certainly above the CNS processing speed, as noted by the sensation of constant stimulation by most patients and this frequency. As in cinema, however, it remains to be seen if even higher rates of stimulation (400–800 Hz) provide a sense of “high definition (HD)” to the CNS that could have therapeutic benefit.
Thickness of the Dorsal CSF Layer
The thickness of the CSF layer between the implanted epidural contacts and the surface of the dorsal columns or other neuronal structures varies considerably within each individual patient based on posture and location within the spinal canal [21]. With increasing thickness of the CSF (dCSF) between individual contacts and neuronal surfaces, there is increasing influence of the electrical impedance of the CSF, requiring increased intensity of stimulation to achieve paresthesias. However, with increased distance from the contact, coupled with increased amplitude, the size of the electrical field across the surface of the targeted neuronal structures becomes larger and optimal target stimulation is easily compromised. In transitioning from prone to supine, there is a dorsal movement of the spinal cord within the dural and the dCSF can decrease remarkably. Unless the amplitude of the stimulation is decreased concomitantly, the patient may feel too intense a stimulation, and be uncomfortable, or the patient may experience too wide a field of stimulation, with collateral paresthesias that are similarly uncomfortable. Alterations in the local dCSF are also seen with transitioning from laying to sitting, sitting to standing, extending and flexing the spine, and lateral bending or twisting. All these considerations are important during the programming of the implanted device to assure optimal therapeutic benefit to the patient.
In summary, the efficacy of neurostimulation is dependent upon two fundamental concepts: appropriate placement of the electrode array (lead) in order to electrically modulate the bioelectric phenomena of the targeted neuronal tissue and the appropriate programming of the electrical neurostimulator system to control the style and character of the neuromodulation of the neuronal surfaces.
Lead Placement
Careful lead placement near the appropriate neuronal target(s) is essential in order to achieve the greatest likelihood of therapeutic stimulation within the superficial layers of the neuronal structure: peripheral nerve, DRG, dorsal roots, DREZ, dorsal columns, superficial motor cortex stimulation (MCS), or deep brain stimulation (DBS). It is crucial to remember the principle that the ultimate target of all of our neurostimulation efforts is the thalamus and that portion of the CNS involved in the perception of painful signals, and the response to pain.
Electrical Field Shaping (Programming)
Contact configuration and amplitude determine the size and shape of the stimulatory field. Pulse width can exert an influence on the total number of fibers stimulated by a specific stimulatory pulse by causing the stimulatory field to “linger” over the neuronal target, recruiting a greater number of fibers. Frequency can alter the repetition of activation and at higher rates (>1,000 Hz) can theoretically create a situation in which many fibers in the superficial layers of the dorsal columns can become relatively refractory.
Therapeutic Goals
The goal of therapy in electrical neurostimulation is to alter the pain state of the patient in such a way as to enhance the capability of achieving success with functional rehabilitation. Neuropathic pain in the extremities is often accompanied by abnormal muscle tone, usually episodic in nature, as well as autonomic dysfunction: decreased blood flow to connective tissue, muscles, and neuronal tissues; temperature abnormalities; and perspiration dysfunction. To achieve improvement in physical function, these autonomic features must be addressed along with the underlying pain complaints, to improve the metabolic state of the affected extremity. For this reason, it is often not sufficient for the patient to “feel stimulation” in the affected area and yet still have the autonomic dysfunction.
It is quite important to realize that the role of electrical neurostimulation is not limited to “pain relief” per se but should serve as an important tool in the rehabilitation of the patient. As such, it becomes a part of the overall plan of therapy for the patient to be integrated with functional rehabilitation, oral medication management, behavioral therapy, and conservative spinal injection techniques.
Clinical Examples: Treatment of Radicular Pain Syndromes
The term “radiculitis” is from the Latin radix – root – and is defined as the inflammation or irritation of the nerve root between the spinal cord and the exit of the nerve root from the canal [22]. As such, any irritation of a lumbar nerve root would be expected to create sensations (often painful) that “radiate” from the axial spine to the periphery. But note that the emphases of the definition are on the nerve “radix – root”– and not on the concept of radiating (from Latin radiare – “to emit rays”). Thus, painful sensations perceived along the axial distribution of the spine can result from a radiculitis or a radiculopathy of the nerve roots. That can be a significant clinical challenge. It can also provide a significant advantage for the clinical application of neurostimulation.
As noted by Malik and Benzon [23], the clinical syndrome of radicular pain in a predictable dermatomal pattern, characterized by subjective reports of sensory disturbance (paresthesia, dysesthesias, numbness, hyperalgesia, allodynia, etc.), and typical objective signs of weakness, decreased reflexes, and positive dural tension signs is referred to by a variety of terms: radiculopathy, radiculitis, and radicular syndrome. However, as they point out, the term radiculopathy inappropriately implies the presence of objective signs of pathological nerve root damage, including loss of sensation, muscle weakness, and diminished reflexes. But all of these objective signs can occur without objective evidence of anatomical or pathological nerve damage. In a similar fashion, radiculitis inappropriately implies an inflammatory process as the sole etiology for the causation of the radicular signs and symptoms. The term radicular pain syndrome appears to be most appropriate and correctly suggests a constellation of clinical signs and symptoms of variable etiology secondary to pathology or dysfunction of the sensory nerve roots or the dorsal root ganglia (DRG).
Thus, a radicular pain syndrome can be axial in distribution, or it can be manifested as radiating into an extremity, or a distribution of pain can occur in both the spinal axis and in the extremities concurrently.
Cervical Radicular Pain Syndrome
Post-laminotomy syndrome of the cervical spine s/p anterior cervical discectomy and fusion (ACDF), also known as failed neck surgery syndrome, often manifests itself with continued cervicogenic headache (CHA) [24–26], primary discogenic pain above and below the level of the fusion, axial neck pain, and upper extremity radiculitis/radiculopathy. Little is known about the neuropathic nature of primary discogenic pain in the cervical spine, but it has been well studied in the lumbar spine and has been found to have a nociceptive etiology with heavy autonomic nervous system transmission of sensory signals [27]. Thus, perceived pain within the axial spine, centrally and laterally, may have a neuropathic-like component susceptible to neurostimulation therapy; it is not necessarily always due to nociceptive facet arthropathy. Because it is neuropathic in nature, neurostimulation efforts can be of substantial benefit in treating this form of pain. Additionally, chemical radiculitis secondary to a disrupted cervical disc annulus can provoke tremendous neuropathic discomfort of the nerve roots, also suitably treated with neurostimulation. (This pattern of pain pathology is frequently seen with patients having a relatively normal MRI appearance of the cervical disc, but to exhibit significant leakage of radiocontrast material injected during provocative discography into the nucleus of the disc.)
In the example shown below (Fig. 43.7
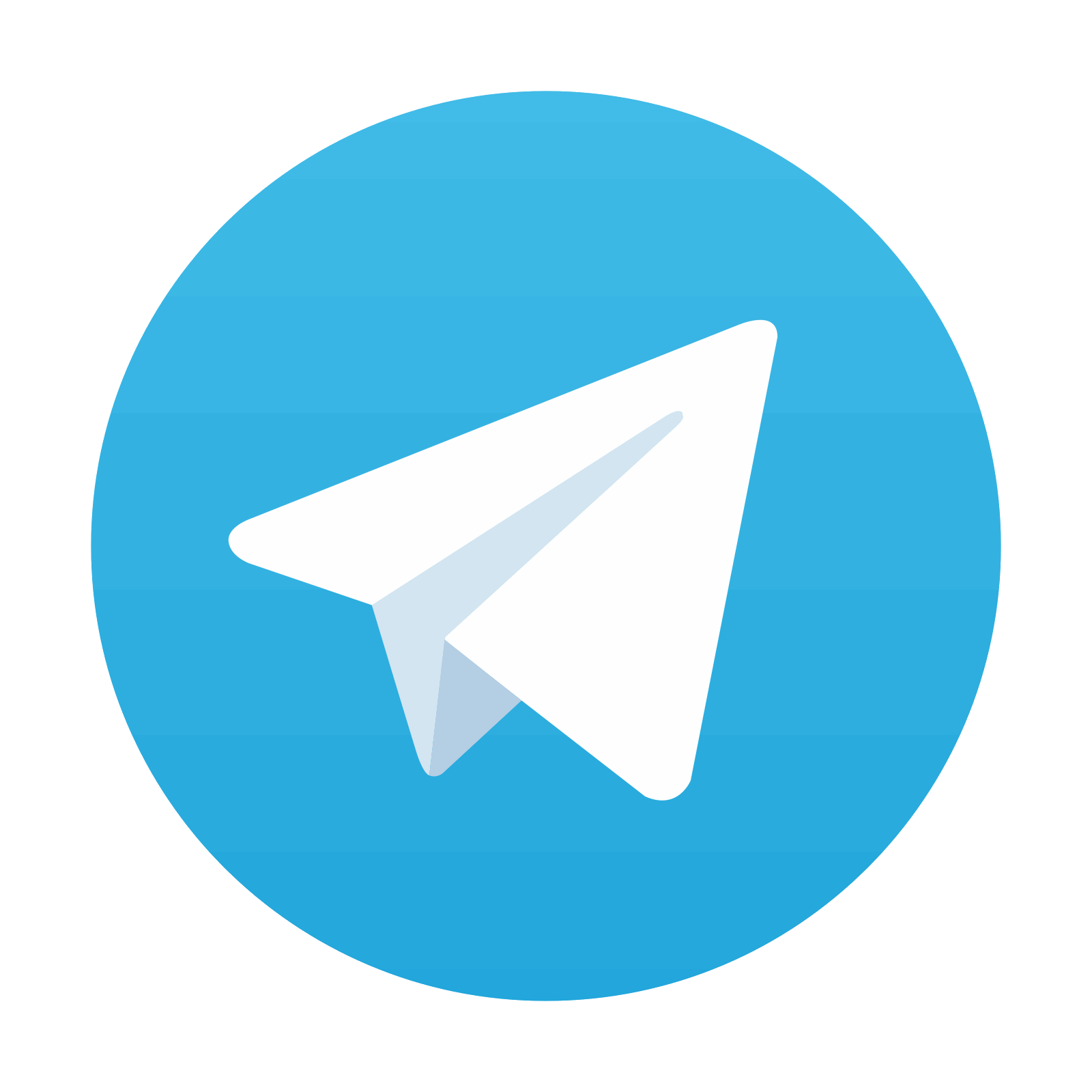
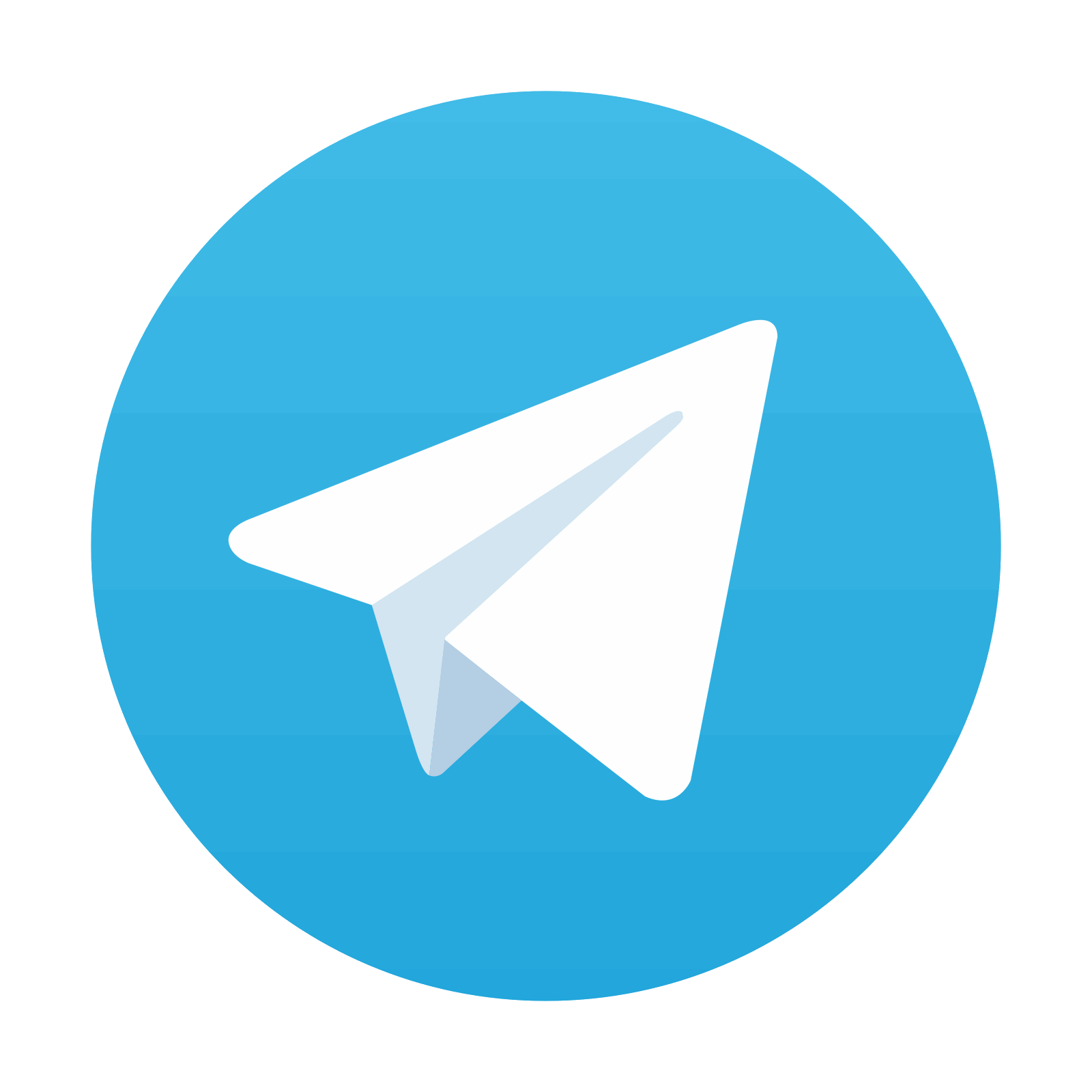
Stay updated, free articles. Join our Telegram channel

Full access? Get Clinical Tree
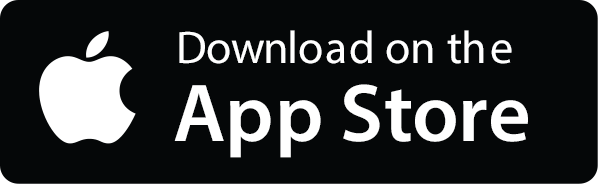
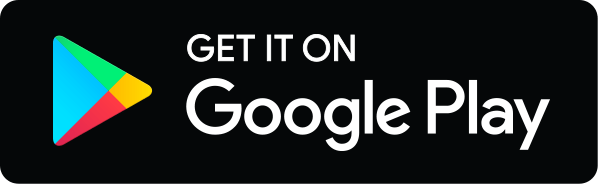