PET/MR imaging is in routine clinical use and is at least as effective as PET/CT for oncologic and neurologic studies with advantages with certain PET radiopharmaceuticals and applications. In addition, whole body PET/MR imaging substantially reduces radiation dosages compared with PET/CT which is particularly relevant to pediatric and young adult population. For cancer imaging, assessment of hepatic, pelvic, and soft-tissue malignancies may benefit from PET/MR imaging. For neurologic imaging, volumetric brain MR imaging can detect regional volume loss relevant to cognitive impairment and epilepsy. In addition, the single-bed position acquisition enables dynamic brain PET imaging without extending the total study length which has the potential to enhance the diagnostic information from PET.
Key points
- •
PET/MR imaging for whole-body oncologic imaging performs at least as well as PET/CT and may have advantages for certain malignancies.
- •
Pelvic, liver, and soft-tissue malignancies are additional indications benefitting from the advantages offered by simultaneous PET/MR imaging.
- •
PET/MR imaging is well suited to brain imaging and has the potential to grow substantially for evaluation of cognitive impairment and neuro-oncology.
- •
PET/MR imaging can reduce radiation exposure compared with PET/CT and reduce the burden of diagnostic imaging for some oncologic and neurologic applications.
Introduction
Positron emission tomography (PET) and magnetic resonance imaging (MRI) are advanced clinical imaging modalities that in combination can provide structural, morphologic, functional, and molecular information about disease and physiologic processes in a single imaging session. Several simultaneous PET/MR imaging scanners are FDA-cleared and in clinical use although they are not as widely used as PET/CT scanners. Systems capable of acquiring PET and MR imaging data at the same time can provide unique advantages for both clinical and investigational applications. This article reviews current clinical applications of PET/MR imaging for oncologic and neurologic imaging including advantages and limitations compared with PET/CT and separately acquired PET and MR imaging.
A current challenge in clinical PET/MR imaging is establishing applications where PET/MR imaging is clearly superior to other imaging modalities, particularly PET/CT and separately acquired PET and MR imaging. Much of the available data on clinical PET/MR imaging reflect the lower bounds of utility compared with standard of care and established imaging techniques such as PET/CT. Some of the published results rely on sequences, radiopharmaceuticals, or processing techniques that are not universally available limiting their applicability in general practice settings.
Practical Considerations, Strengths, and Challenges
Instrumentation
One of the key advances that enabled simultaneous PET/MR imaging was the development of mutually compatible PET detectors, MR coils, and MR gradients. The PET insert in current systems does not distort static magnetic field (B0), radiofrequency field (B1), or linear field gradients, and the MR image quality is similar to MR-only systems. PET subsystem cannot use certain crystal materials such as gadolinium orthosilicate that affect the magnetic field. The PET insert reduces the diameter of the bore which can cause difficulties for obese or claustrophobic patients. Surface coils and scanner hardware between the PET insert and patient attenuate the gamma photons, but the reduction in the overall sensitivity of the PET subsystem is practically inconsequential.
Attenuation Correction
Attenuation of photons arising from positron annihilation results in reduced PET signal detection, particularly in deep tissues. Attenuation correction (AC) methods are used to produce PET images that visually and quantitatively reflect the true distribution of the PET radiopharmaceutical. Attenuation maps derived from CT are widely accepted as the clinical standard of PET AC. Unlike CT, MR imaging signal is not directly related to the photon-attenuating properties of tissues and cannot be used directly to estimate 511-keV photon attenuation. Instead, MR imaging-based AC (MRAC) uses MR imaging sequences to characterize tissue composition which can be used alone or in combination with an atlas for segmentation of the body into various tissues such as fat, soft tissue, lung, air, and bone for which the attenuation coefficients are known. MRAC maps can introduce errors in standardized uptake value (SUV), particularly within or adjacent to cortical bone if bone is not represented in the attenuation-correction map. The magnitude of these errors is typically on the order of 10% to 20%, is clinically insignificant for most applications, and generally does not affect diagnosis even for osseous lesions. During clinical interpretation of PET/MR imaging studies, the MRAC map should be reviewed to insure that tissue segmentation was performed correctly.
Continued progress and improvements in MRAC are increasing the quantitative accuracy of PET/MR imaging. Light-weight surface coils minimize attenuation that is not accounted for by the scanner, unlike embedded coils and other fixed hardware. , Ultrashort and zero echo time sequences can characterize osseous structures and assess tissue components in areas of high magnetic field inhomogeneity such as lungs or gas-filled bowel and are used to improve segmentation methods. , Segmentation can also be improved using a deep learning model or other machine learning approaches. PET/MR imaging can surpass the quantification accuracy of AC compared with legacy PET scanners or PET/CT in areas where motion results in misalignment between transmission and emission images by exploiting simultaneous acquisition and motion tracking. Time-of-flight systems allow joint estimation of activity and attenuation based on PET data which can overcome challenges in MRAC, and when combined with MR-based systems mentioned previously, they improve the robustness and accuracy of PET quantification in organs and structures such as lung or bones or near-metallic hardware that conventional MRAC had been deficient. Using these techniques, Rezaei and colleagues recently reported a residual quantification error below 1% in brain PET/MR imaging.
Workflow
Simultaneous acquisition of PET and MR imaging can provide patient convenience compared with two separate sessions. , In applications where only one PET bed position is needed (eg, brain or cardiac imaging), standard of care PET and MR imaging can be acquired very efficiently using a simultaneous PET/MR scanner with no or minimal change to well-established imaging protocols. In other applications such as whole-body oncologic imaging, a one-stop scan requires tailored protocols that can acquire PET and MR imaging images with adequate diagnostic quality within a certain time constraint such as 60 minutes of total imaging. Inclusion of dedicated sequence for pulmonary imaging such as respiratory-triggered, periodically rotated overlapping parallel T2-weighted imaging which can approach the diagnostic accuracy of low-dose CT for pulmonary nodules reduces the need for a separate chest CT in most instances.
The z-axis coverage of the PET subsystem is fixed (eg, 25 cm or 25.8 cm in current iteration of integrated PET/MR imaging system). Acquisition of skull to mid-thigh or whole-body PET requires scanning at multiple table positions (typically between 4–6). The minimal PET acquisition time is relatively similar between different table positions. In contrast, the z-axis coverage and acquisition time vary significantly depending on the MR imaging sequence.
The Dixon sequence with volume coil is used for segmentation-based MRAC and provides phase, opposed-phase, water, and fat images which can be used for basic anatomic correlation. Using coronal acquisition covering two stations in z-axis and adding fat saturation at every other table position can be used to provide images with high soft-tissue contrast efficiently. However, additional sequences such as volumetric T2-weighted images or whole-body diffusion-weighted imaging (DWI) can increase MR acquisition time significantly. The protocol requires compromises to fit the timing and order of acquisition of different table positions for simultaneous MR imaging and PET, and the overall clinical question should be considered in designing the protocol.
Improved Anatomical Registration
An advantage of PET/MR imaging is improved coregistration of PET with anatomic images through simultaneous acquisition of both modalities. Changes in location of structures between anatomic (ie, CT or MR imaging) and PET image acquisition can introduce diagnostic errors such as misinterpretation of activity or mis-localization of a lesion. With simultaneous acquisition, bulk motion from patient repositioning is minimized, improving anatomic coregistration compared with sequential acquisition with PET/CT or separately acquire PET and MR imaging studies. ,
PET and MR sequences are affected by movements of the diaphragm and rib cage resulting in nonrigid motion and elastic deformation of adjacent structures during respiration, as well as periodic or irregular movements in other structures such as cardiac contractions and bowel peristalsis. Depending on the MR sequence, these effects manifest differently, and certain sequences require breath hold or gating or the use of navigator pulses to minimize distortions or other artifacts. Movements during PET acquisition result in blurring of the PET images and quantification errors due to lower recovery coefficient or mismatch between MRAC maps and PET. , When multiple MR sequences (eg, free breathing and single-shot breath hold sequences) are acquired during PET acquisition at each table position, or if a particular MR sequence is performed separately before or after PET acquisition (as frequently is the case for postcontrast whole-body T1W images), the position of anatomic structures on MR images may not match the position of time-averaged associated activity on PET. Combination of free-breathing PET data with breath-hold MR imaging sequences not only lead to registration errors due to nonrigid deformations in the liver and other upper abdominal organs but may also cause attenuation-correction errors. With improved spatial resolution of clinical PET images, small changes in position and blurring of activity in areas of motion are becoming more noticeable. Various techniques for detection and monitoring motions are currently available and can be paired with correction algorithms in PET after processing. Gating is useful for periodic motions such as respiration or cardiac cycle and, in an application such as cardiac PET/MR imaging, improves spatial resolution, contrast-to-noise, and quantification accuracy compared with nongated PET as depicted in Fig. 1 .
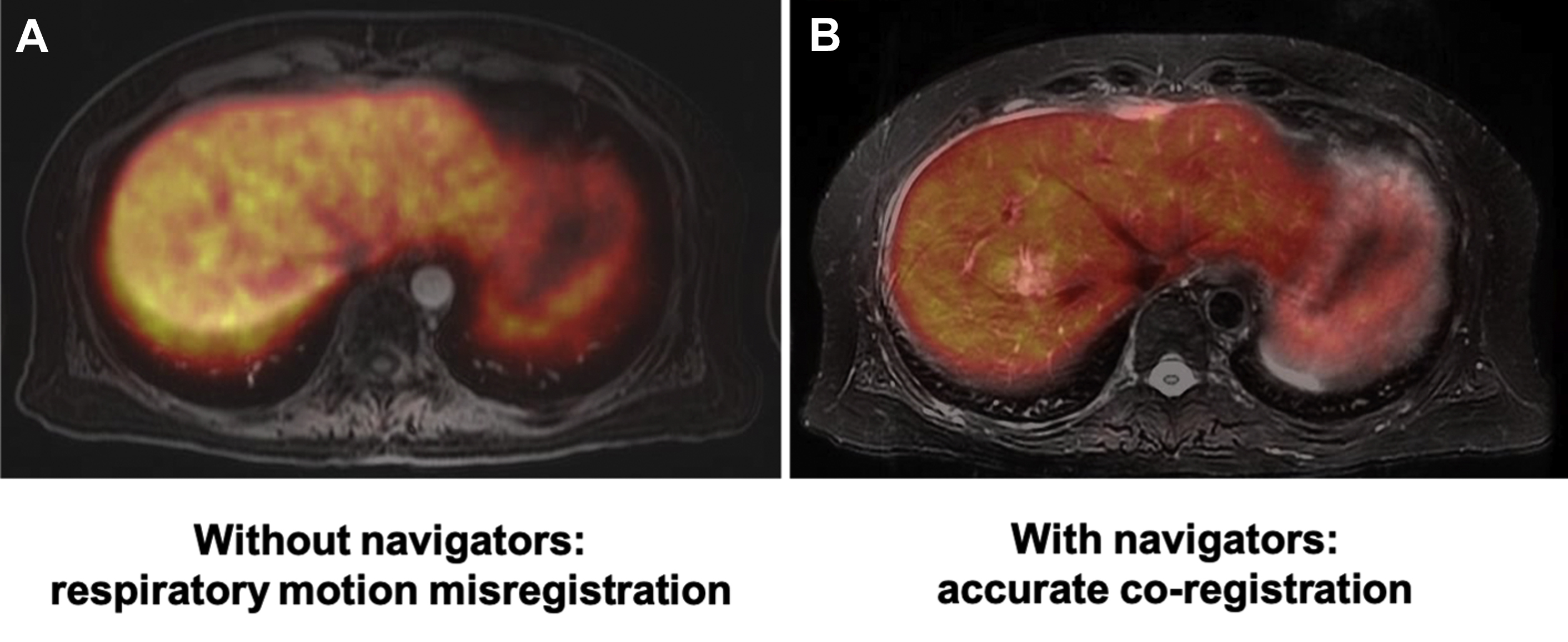
Reducing Radiation Exposure
The effective exposure from low-dose CT can be as high as radiation exposure from the PET agent, and elimination of CT reduces exposure by as much as 50%. Moreover, optimized workflow efficiency allows for a focused MR imaging to be performed in the same session to avoid additional exposure from a separate dedicated contrast-enhanced CT or multiphase CT. An additional 40%-50% reduction of radiation exposure (depending on BMI) compared to conventional PET/CT is feasible because of higher sensitivity of detector rings in modern hybrid PET/MR systems by reducing the injected dose. With MR acquisition protocols that require longer time per bed, the injected activity can be reduced even further. Overall, with careful optimization of the protocol, PET/MR imaging can result in 70% to 80% less radiation than standard PET/CT, , which is particularly relevant in pediatric and young adult populations because of risk of secondary malignancies later in life associated with radiation exposure. ,
Imaging Protocols
Simultaneous focused PET/MR imaging requires minimal change to the optimized standard of care imaging protocol if a single table position provides sufficient coverage for the field of view of PET and MR imaging. Depending on the length and type of study, the PET radiopharmaceutical can be administered before or after the patient is placed in the scanner. In most clinical applications, the PET acquisition time is shorter than that of MR imaging, and ideally the timing should be set up that PET acquisition coincide with an MR sequence that provides AC information as well as sequences that provide the highest anatomic details and most relevant diagnostic information (eg, volumetric postcontrast T1WI for brain tumors, delayed postcontrast T1WI for cardiac sarcoidosis) to maximize registration accuracy.
Whole Body PET/MRI Protocols
Protocols for multistation PET/MR imaging require optimization and often compromise to satisfy certain constraints regarding the order of acquisition, timing at each bed position, and total duration of examination. For typical doses of fludeoxyglucose (FDG) and many other clinically used radiopharmaceuticals, 2 to 5 minutes of PET scan time at each table position is sufficient. Longer acquisition in chest and abdomen can improve detection rate for small pulmonary or hepatic metastases and may be necessary for certain approaches to mitigate motion artifact. A Dixon-based MR imaging sequence for AC and up to three diagnostic MR imaging pulse sequences which typically includes T2WI fit during PET imaging acquisition. A T2-weighted single-shot fast-spin echo sequence in the axial and/or coronal plane is often used in whole-body PET/MR imaging because of its good soft-tissue contrast and anatomic detail, relatively fast acquisition time, and relative insensitivity to motion. An example of typical sequences used for whole-body PET/MR imaging is shown in Fig. 2 . Depending on the body part, additional sequences (eg, respiratory triggered periodically rotated overlapping parallel T2WI of the lungs) may be acquired either simultaneously with PET or before or after PET acquisition. As the diagnostic performance of PET/MR imaging heavily depends on MR sequences, there is ongoing interest and research in developing standardized protocols for PET/MR and incorporation of multiparametric MR imaging tailored to specific clinical applications.
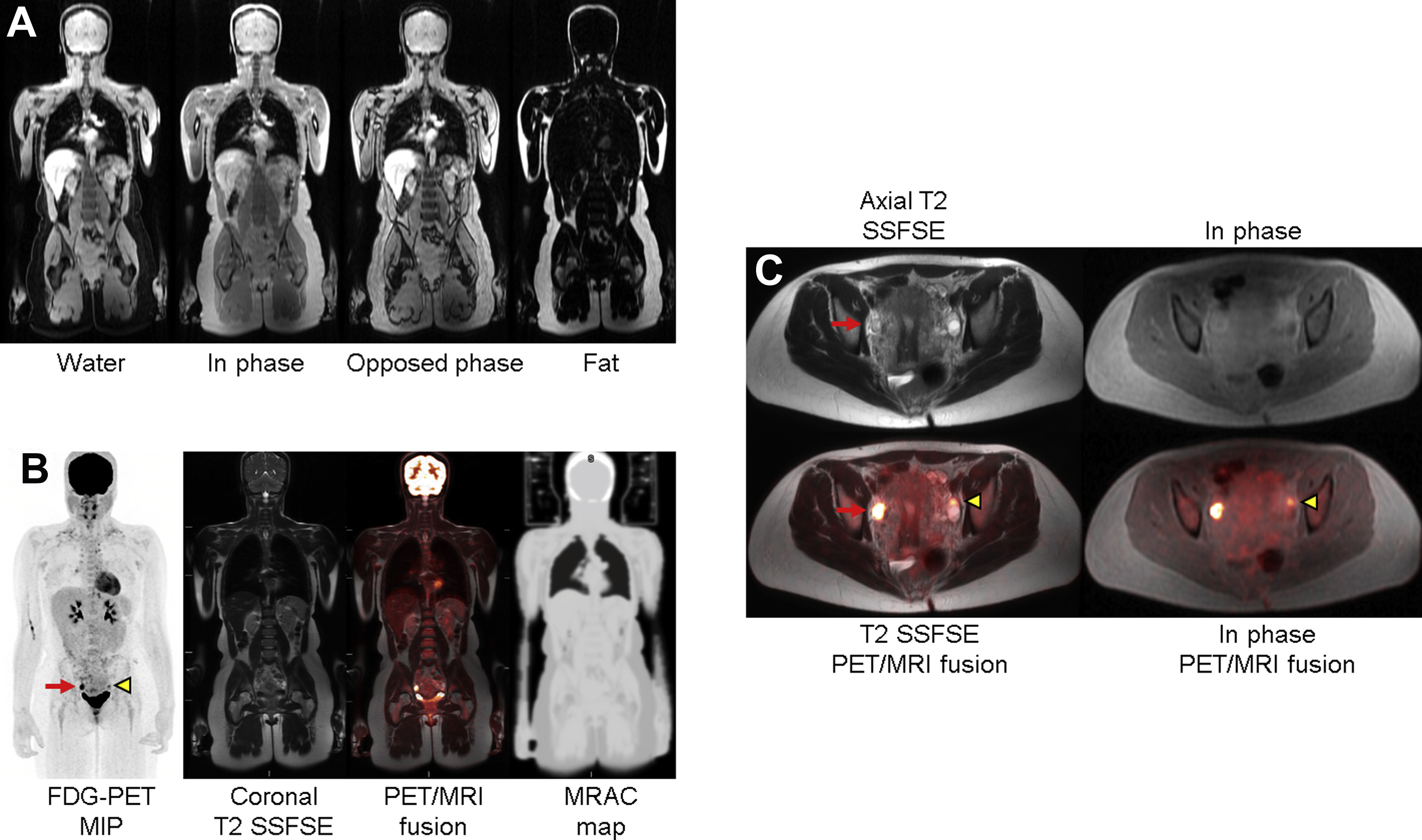
Intravenous Contrast
Administration of contrast is primarily helpful in focused PET/MR imaging of a specific body part. When both focused and whole-body PET/MR imaging are performed in the same session, splitting the extracellular contrast agents into two injections may be necessary. Gadolinium-enhanced T1W imaging can achieve adequate lesion conspicuity and detection rate and good anatomic details in a more time-efficient manner than T2W and DWI. However, the experience on redundancy between PET and contrast-enhanced MR imaging in many clinical scenarios remains limited. With hepatobiliary contrast agents such as gadoxetate disodium or gadobenate dimeglumine, skull-base to thigh PET can be acquired in the interval between initial dynamic postcontrast images of the liver and delayed imaging. Gadoxetate has high uptake by hepatocytes followed by biliary excretion by the canalicular multispecific organic anion transporter, providing excellent contrast between focal lesions and normal hepatic parenchyma on delayed images.
Oncologic Applications of PET/MR imaging
In oncologic applications, beyond improving diagnostic accuracy and facilitating workflow and cost-effectiveness as a one-stop shop for staging or restaging several malignancies, a multiparametric PET/MR imaging can provide biomarkers for disease activity that contribute to precision medicine. A review of more than 100 published articles between 2012 and 2018 supports the utility of PET/MR imaging and superiority to other modalities including PET/CT in several malignancies. The primary disadvantage of PET/MR imaging compared with PET/CT is in assessment of primary pulmonary malignancies and small lung metastases. In many oncologic applications, the diagnostic performance of FDG PET/MR imaging is at least equivalent to PET/CT. Mayerhoefer and colleagues recently reported a significantly higher accuracy for PET/MR imaging than for PET/CT in a mixed population of cancer patients (97.3% vs 83.9%, P < .001) primarily because of better sensitivity for liver and brain metastases, impacting management in 8% of their patients. However, the impact would be smaller if metastases to brain from non-small-cell lung cancer are excluded as these patients routinely get a brain MR imaging as a part of their workup. Another observational study of more than 1000 patients found that although PET/MR imaging revealed additional information compared with PET/CT in more than a quarter of patients, malignant findings were detected only in 5.3% of the studies and lead to a change in staging in about half of those patients (2.9%). At the same time, PET/MR imaging missed malignant lesions (compared with PET/CT) in 2.9% of studies, but the staging was affected in less than a fifth of those studies. PET/MR imaging reduces the need for additional workup in 11% of patients by characterizing indeterminate lesions on PET/CT but resulted in additional studies in 26% of patients, of which less than a third were malignant. Considering limited availability of PET/MR imaging systems and concerns about cost and patient tolerability, there is interest in improving triage of patients based on histopathology and clinical stage.
Head and Neck
FDG PET/MR can have excellent diagnostic performance in staging head and neck malignancies , and has certain advantages to other available imagining modalities for initial evaluation and assessment of response to therapy. , T-staging is improved by addition of high-resolution dedicated head and neck MR sequences. Excellent soft-tissue contrast of MR imaging enables more accurate delineation of certain cancers such as nasopharyngeal carcinoma than contrast-enhanced CT. T2WI is useful for detection of necrotic or cystic lymph node metastasis. Diffusion weighted imaging provides complementary information to FDG PET and can identify lesions in lymphoepithelial tissues or other areas of high background physiologic activity. MR imaging has excellent sensitivity for bone marrow involvement. Ultrashort echo-time (UTE) or zero echo-time (ZTE) sequences can identify cortical bone erosions reducing the need for a separate CT. Addition of PET to MR imaging increases sensitivity and negative predictive value in detecting local recurrence and metastases. In one small series, PET/MR imaging had higher accuracy than MR imaging alone in diagnosing the primary lesion at initial presentation (75% vs 50%) and local recurrence (57% vs 72%).
The utility of FDG PET/CT (often in combination with contrast enhanced CT) in locally advanced head and neck malignancies is well established. A few studies that have directly compared the diagnostic utility of PET/MR imaging with PET/CT have confirmed similar if not superior accuracy for PET/MR imaging for locoregional assessment , and comparable accuracy for evaluation of distant metastases or synchronous cancers. MR can be used for anatomic correlation of PET findings in the oral cavity or other areas with severe streaking artifacts on CT because of dental hardware. PET/MR has shown to provide accurate staging without the use of intravenous contrast which is an important consideration for patients who are unable to receive intravenous contrast because of allergy or poor renal function. An example of FDG PET/MR imaging for head and neck cancer staging is shown in Fig. 3 .
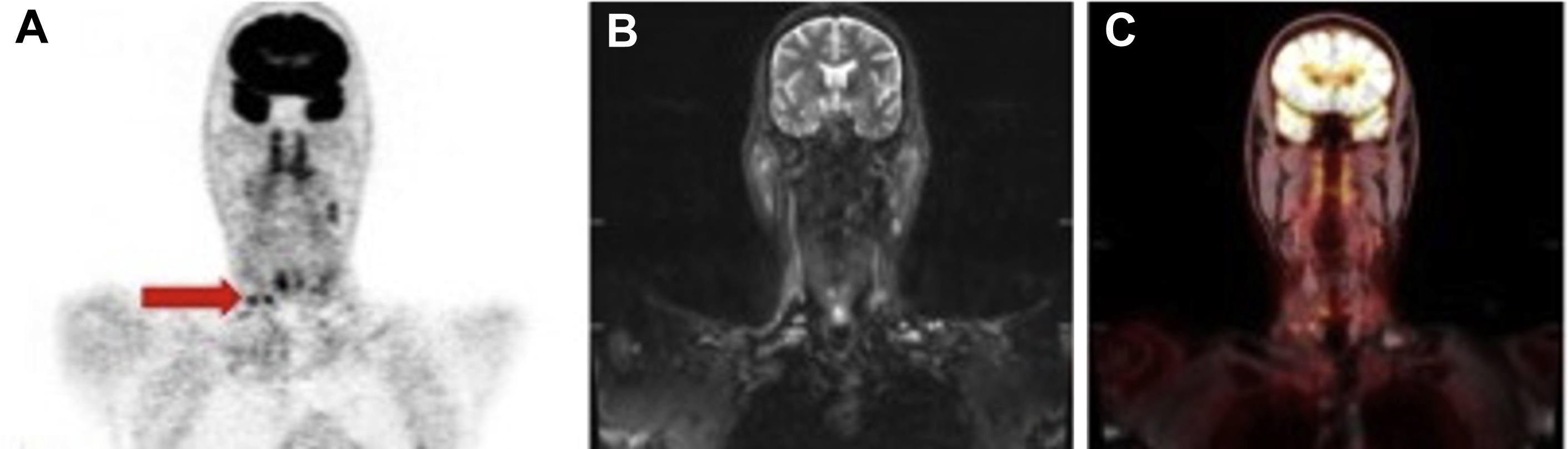
PET/CT may be a better option for patients with hypopharyngeal or laryngeal primary cancers as CT is less degraded by motion artifact. In patients with known or suspected pulmonary metastases, PET/CT appears to be superior for assessment of small pulmonary nodules, particularly if combined with a focused chest CT. However, pulmonary nodules that are missed on PET/MR imaging are generally too small to be accurately characterized by PET, and their clinical significance remains doubtful given the relative frequency of benign incidental small pulmonary nodules.
Gastrointestinal Malignancies
Physiologic FDG uptake in the gastrointestinal tract is variable, and without accurate anatomic correlation, metabolic characterization of gastrointestinal lesions can be challenging. Single-shot spin echo sequences can depict anatomic details and provide high lesion conspicuity in gastric cancer which can improve diagnostic accuracy compared with PET/CT. PET is indicated in initial evaluation of gastric adenocarcinomas and gastrointestinal stromal tumors. However, the role of PET in assessment of small bowel or colon adenocarcinoma is primarily for characterization of equivocal findings on CT or MR imaging and assessment of peritoneal disease. DWI is superior to CT and can be complementary to PET for assessment of the extent of peritoneal involvement. Patients with elevated biomarkers with negative CT and endoscopy could potentially benefit most from the superior sensitivity of PET/MR imaging compared with other techniques.
In patients with rectal cancer, MR imaging and, in particular, small field of view T2WI can identify the extent of tumor beyond the muscularis propria and extension to mesorectal fascia, anal sphincter, or other pelvic structures, assess pelvic lymph nodes, and distinguish between posttreatment change and recurrence. FGD PET can help characterize small lymph nodes, and in equivocal cases, complementary information from PET improves T and N staging accuracy and detection of recurrence. Detection of distant metastases on whole-body PET-MR imaging affects management. The accuracy of N and M staging or restaging on PET/MR imaging is similar if not superior to PET/CT. , In one series, PET/MR imaging was found to incrementally increase accuracy compared with MR imaging for both T staging (92% vs 89%) and N staging (92% vs 86%) compared to pathology gold standard after neoadjuvant therapy and resulted in a change in management in 11% of the patients. High soft-tissue contrast of MR imaging can significantly improve diagnostic value of PET for assessment of recurrence. Plodeck and colleagues report a sensitivity and specificity of 94% and positive and negative predictive value of 97% and 90%, respectively, for detection of recurrence using a nonsimultaneous PET/MR system, with PET/MR imaging leading to a change in management in 18% of their patients. Simultaneous PET/MR imaging systems provide excellent coregistration between anatomy and metabolic activity which can be used to match individual mesorectal nodes and deposits to histopathologic samples. Addition of single-bed prolonged PET acquisition over the pelvis which can be performed after voiding and simultaneous with dedicated high-resolution volumetric MR imaging increases image quality and sensitivity compared with standard-of-care PET/CT or generic multistation oncologic PET/MR imaging.
The literature on clinical utility of PET/MR imaging in rectal carcinoma compared with conventional imaging has not been entirely conclusive so far. One study, for example, reported upstaging in more than 21% and downstaging in 14% that led to a change in management. In contrast, the preliminary results from the REctal Cancer Trial on PET/MR imaging/CT has not yet shown a change in management for any of the patients who underwent PET/MR imaging. It has been argued that the incremental added diagnostic value of PET/MR imaging compared with MR imaging alone might not be sufficient to justify its preferential clinical use in rectal cancer. Liver is a frequent site of metastases in gastrointestinal malignancies (including neuroendocrine neoplasms [NENs]), and the combination of PET and MR imaging has shown to improve accuracy and diagnostics confidence for hepatic metastases detection. ,
Neuroendocrine Neoplasms
68 Ga- and 64 Cu-labeled somatostatin receptor (SSTR) ligand PET agents have high sensitivity and specificity for detection and localization of SSTR-expressing neoplasms including many tumors of neuroendocrine origin particularly for gastroenteropancreatic well-differentiated neuroendocrine tumors (GEP-NETs) and pheochromocytoma/paragangliomas. MR imaging is superior to CT (particularly in the absence of intravenous contrast) for identifying anatomic correlates to the PET signal. Multiparametric MR imaging of the abdomen and pelvis has excellent sensitivity for hepatic lesions and can help identify lesions in areas of high physiologic uptake that are not conspicuous on PET because of small-size and partial-volume effect and/or low somatostatin analog overexpression (Krenning score 1–2). Delayed liver MR imaging with gadoxetate has been shown to have superior sensitivity for liver lesions compared with 68 Ga-DOTATOC PET. Complementary information on MR imaging such as growth over time or restricted water diffusion can be crucial in identifying pseudoprogression or pseudoresponse. Many patients with GEP-NETs therefore undergo both whole-body PET/CT and abdominopelvic MR imaging at both initial evaluation and for assessment of response to therapy, particularly for liver-dominant disease as shown in Fig. 4 . Combining whole-body PET and MR imaging and dedicated abdominal MR imaging for these patients at the least achieves workflow efficiency and reduces ionizing radiation exposure. The diagnostic performance of PET/MR imaging even without administration of intravenous contrast is comparable if not superior to multiphase contrast-enhanced PET/CT, and a combination of DWI and SSTR-PET was able to detect more lesions than either technique by itself.
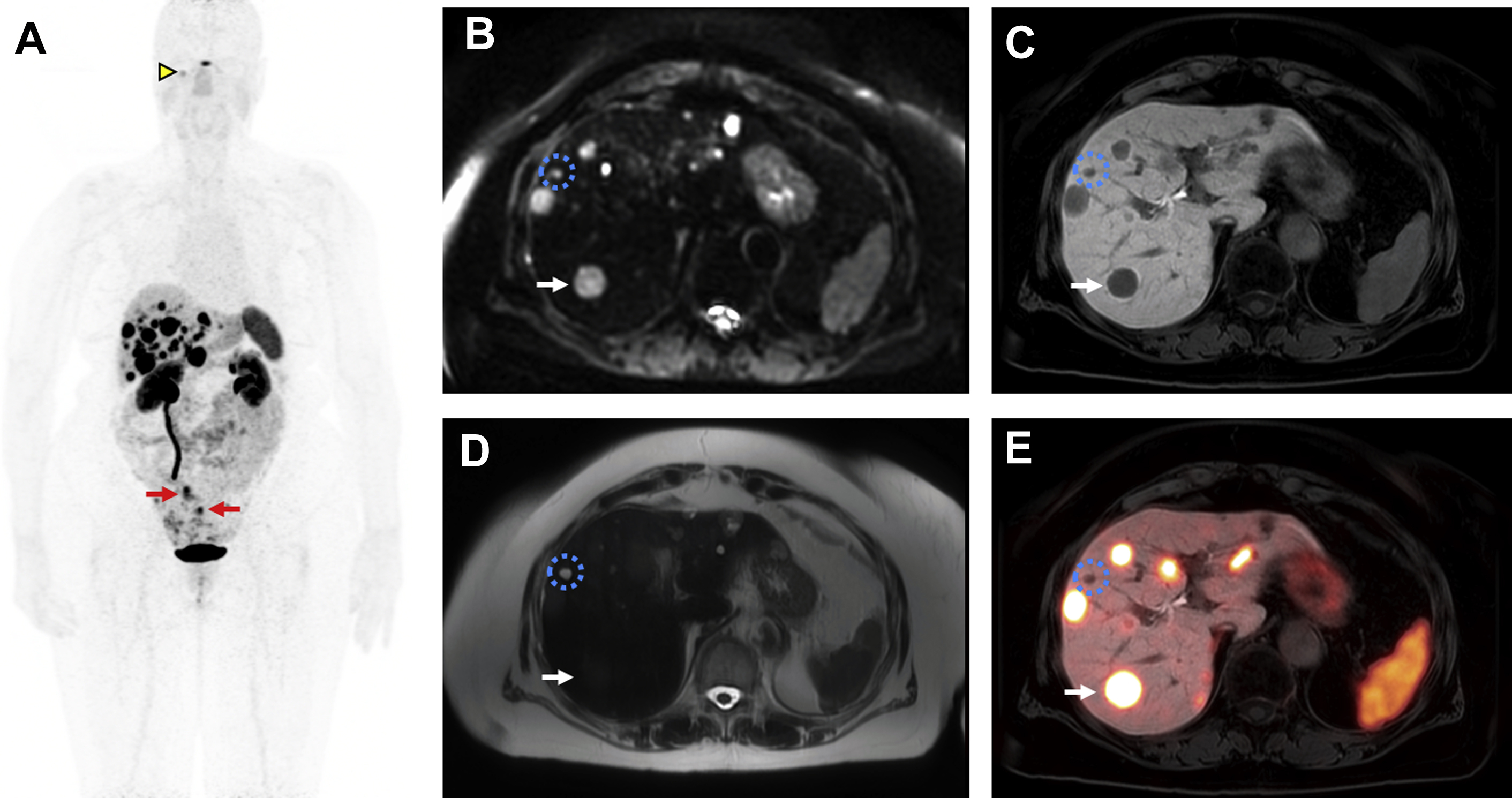
Prostate Carcinoma
The role of multiparametric MR imaging in diagnosis and staging of prostate cancer (PCa) is well established, and the recent development of several PET agents for PCa imaging make PET/MR imaging a promising modality for PCa imaging. A combination or high-resolution T2-weighted (T2W) and diffusion-weighted images can identify most clinically significant PCas particularly at with a 3-T MR imaging system. T2W image delineate central and peripheral gland and may detect extracapsular extension. , High b-value images (≥1400 s/mm 2 ) facilitate detection of clinically significant cancers. Dynamic contrast-enhanced (DCE) MR imaging uses increased neovascularity and permeability of microvasculature within PCa to identify and characterize lesions that equivocal on other sequences or in areas difficult to evaluate on DWI due to artifact. Targeted biopsy of lesions seen on MR imaging improves detection of clinically significant cancers and reduced detection of insignificant cancer compared with systematic biopsy in patients with elevated prostate-specific antigen (PSA). Nonetheless, MR imaging fails to detect primary cancer or underestimates the extent of disease in a small but significant number of patients. PET using prostate-specific membrane antigen (PSMA) ligands has better diagnostic performance than multiparametric MR imaging in the detection of PCa in intermediate- or high-risk PCa patients. In one case report, PET-guided biopsy was necessary to establish the diagnosis of cancer in a patient who had four negative MR imaging studies and six negative systematic biopsies despite persistently elevated PSA. Combining PET with multiparametric MRI (mpMRI) is particularly useful as it enables image-guided fusion biopsy and planning for targeted localized therapy.
MR imaging and CT have limited sensitivity and accuracy for nodal metastases which are a poor prognostic factor [62]. Patients with nodal involvement may benefit from treatment options such as radiation therapy [64]. However, surgical staging with extensive nodal dissection can have high morbidity and not feasible if nonsurgical management is pursued. PET can detect metastases in subcentimeter lymph nodes [67]. Various PET radiopharmaceuticals such as choline-analogs [68], 18 F-fluciclovine [69], and, in particular, PSMA PET outperform anatomic imaging for nodal staging [70] [71–73]. A high negative predictive value of PSMA PET for nodal involvement in initial staging of PCa , makes it a cost-effective alternative to extended pelvic dissection and has been shown in multicenter randomized studies to inform management, altering panned management in more than a quarter of patients. An example of PSMA-PET/MR imaging for initial staging of PCa is shown in Fig. 5 .
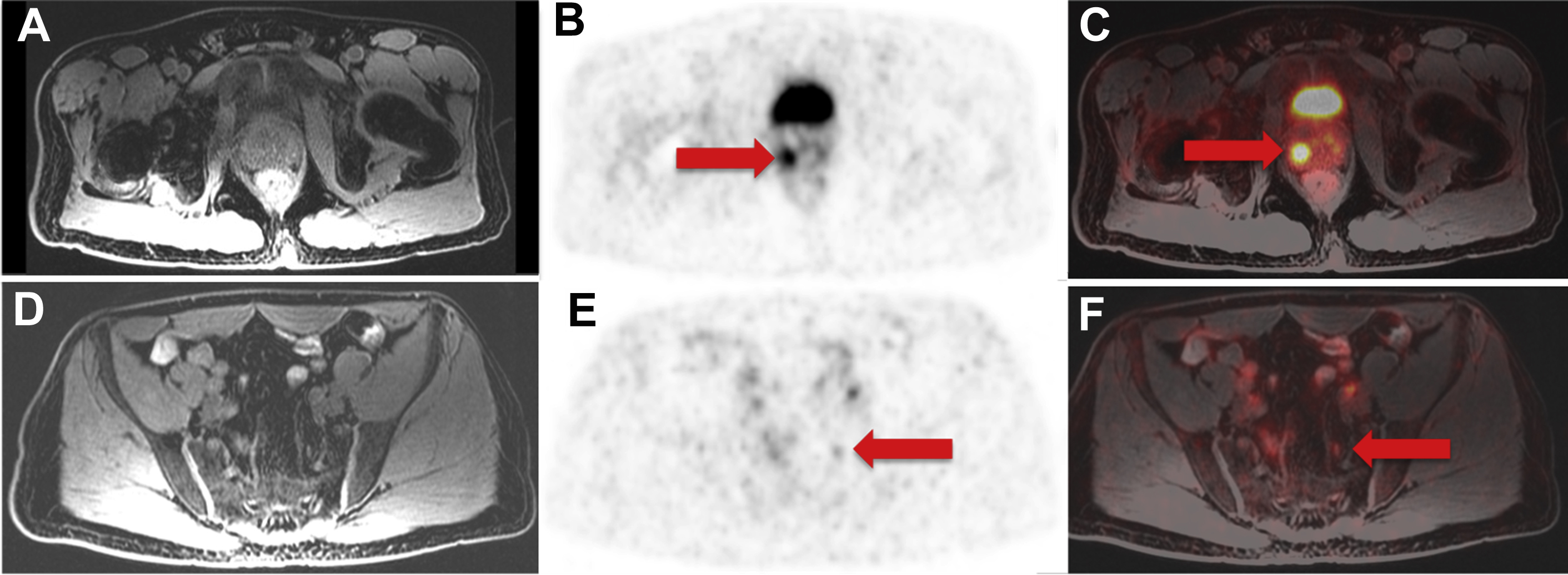
PET/MR imaging is also a promising tool for evaluation of patients with persistent elevation or rising of serum PSA from the nadir after definitive treatment (biochemical recurrence) without evidence of disease on anatomic imaging (structural recurrence). Early localization of disease with PET or MR imaging enables targeted therapies which can affect survival or prevent futile. In one series, PET was able to detect occult metastases in more than half of patients. In another retrospective study of more than 1000 men with biochemical recurrence, the positivity rate of PSMA PET was close to 80%. Even if anatomic imaging reveals abnormalities in patients with biochemical recurrence, accurate characterization of these abnormalities and identification of the extent of disease with PET/MR imaging can prevent futile and potentially harmful interventions. Inclusion of multiparametric pelvic MR imaging improves diagnostic accuracy in prostate bed, particularly for radiopharmaceuticals with urinary excretion. Whole-body MR imaging is an accurate and cost-effective tool in detecting distant nodal and osseous metastases , with higher sensitivity than bone scintigraphy and approaching NaF-PET/CT. , Inclusion of PET, mpMRI of the prostate, and whole-body MR imaging in the same session enables a one-stop shop for evaluation of patients with biochemical recurrence. An example of fluciclovine-PET/MR imaging for PCa is shown in Fig. 6 .
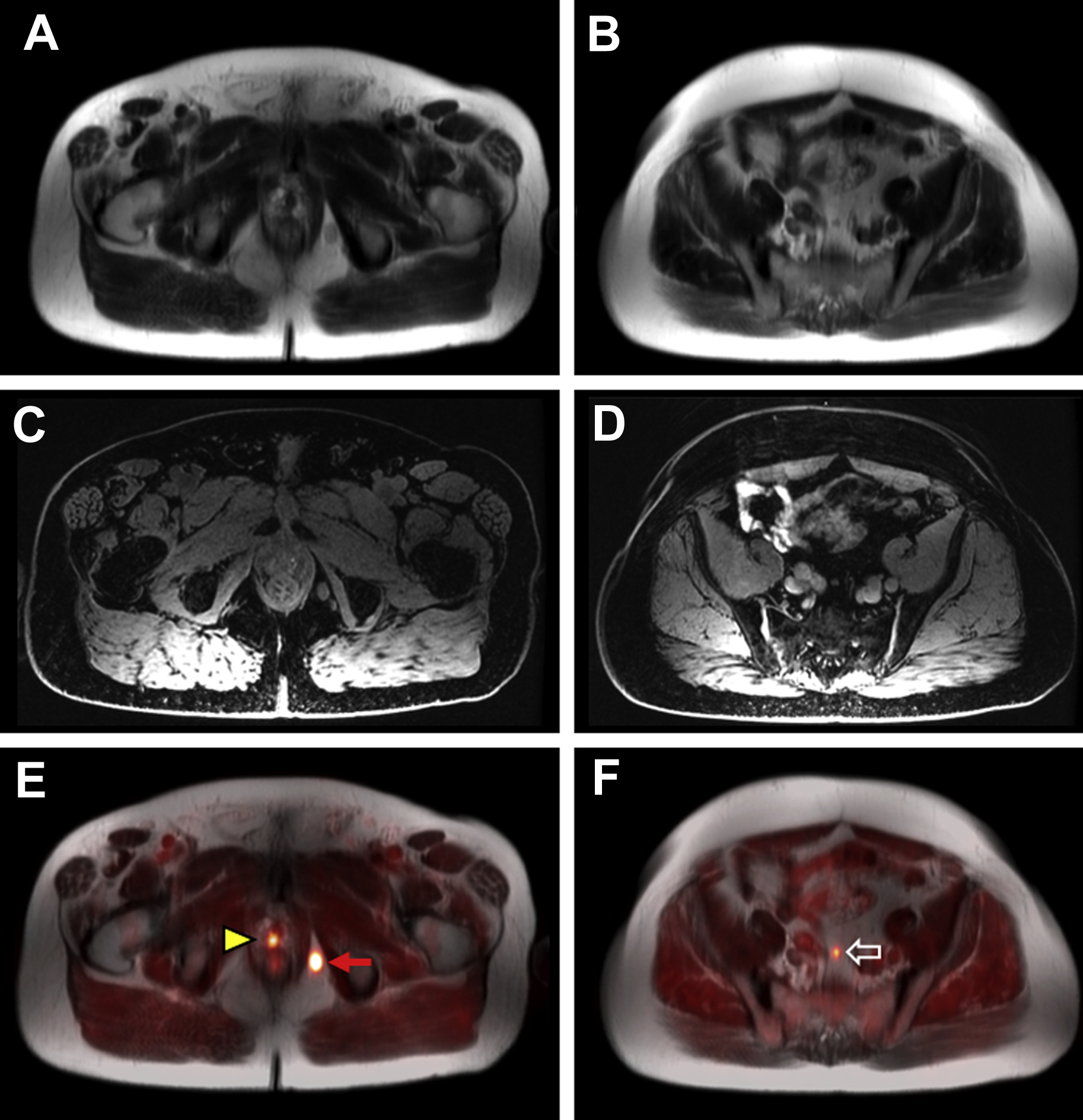
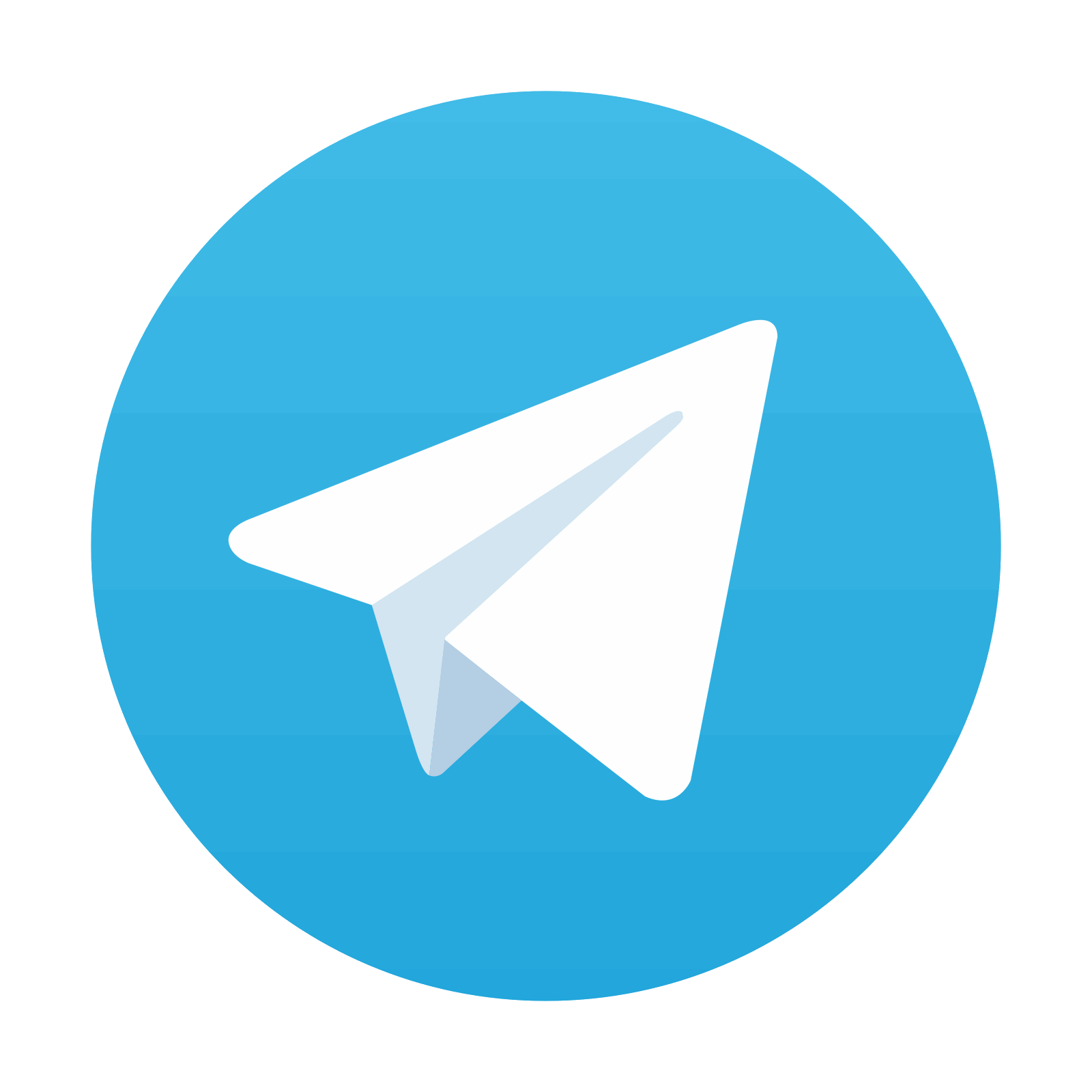
Stay updated, free articles. Join our Telegram channel

Full access? Get Clinical Tree
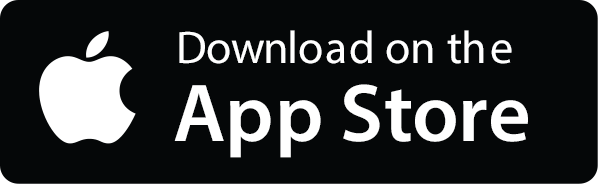
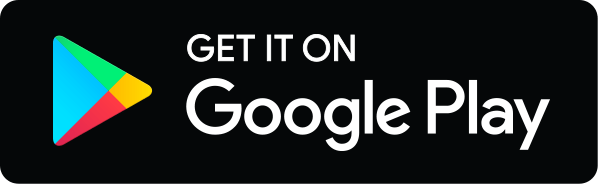
