Collateral Flow, Luxury Perfusion, and Vasospasm: Clinical Applications and Theoretical Principles
Mateo Calderon-Arnulphi
Peter D. Schellinger
David S. Liebeskind
Collateral Flow
Evaluation of the cerebral collateral circulation is fundamental in the management of cerebrovascular occlusive disease. In selected groups of patients, it may provide key information for therapeutic decision making as well as serve an important prognostic role. However, the exact use and protocols for how these techniques should be used in the clinical setting have not been completely resolved. This chapter presents the use of computed tomography perfusion (CTP) and magnetic resonance perfusion (MRP) for the evaluation of collateral circulation in the brain. Furthermore, it describes how such imaging depicts the pathophysiology of ischemic disease and can provide aid in therapeutic planning and prognosis.
The cerebral collateral circulation refers to the subsidiary network of vascular channels that stabilize cerebral blood flow when principal conduits fail.1 Collateral circulation in the brain is one of the most influential factors in mediating the potentially devastating effects of cerebral ischemia.2 The pace of cellular death in the brain after an arterial occlusion is closely related to the severity of decreased of blood flow within that region. Normal blood flow is approximately 50 mL/100 g/min and is tightly controlled by cerebral autoregulation. When blood flow is less than 10 mL/100 g/min, cells die within minutes.3,4,5 During blood flow states between 10 and 20 mL/100 g/min, neuronal function ceases without loss of structural integrity, allowing for potentially revival if normal blood flow is restored. Brain collateral circulation provides perfusion distal to arterial occlusions or stenoses. Depending on the extent of such collaterals, infarction may not be completed for hours or even days or the course may remain asymptomatic. In this manner, collateral circulation determines the hemodynamic features of ischemic penumbra, pace of infarct evolution, and the propensity of hemorrhagic transformation. From a therapeutic standpoint, robust collaterals are a potent predictor of arterial recanalization, preserved tissue fate, and good clinical outcomes.
Anatomy
The collateral circulation to the brain can be divided into three principal groups.6
First, large artery communication between the extracranial and intracranial circulation, including retrograde flow through the ophthalmic artery (Fig. 44.1),7 superficial temporal artery to the intracranial vessels, and anastomoses between the vertebral arteries and muscular branches at the cervical level (e.g., via caudal neuromeningeal branches of the ascending pharyngeal artery).
Second, the circle of Willis (COW), which equilibrates blood flow distribution and can redirect flow in the event of arterial stenosis or occlusion.7,8 The COW is complete in approximately 50% of individuals. Common variations of the COW include absence of anterior or posterior communicating arteries, absence of A1 or P1 branches, multiplication of vessels, or fetal origin of the posterior cerebral artery (PCA) (i.e., PCA is coming of from the anterior circulation). These abnormalities can significantly alter or impair the COW’s function.
Third, leptomeningeal anastomoses that provide blood flow to the cortical surface and adjacent regions (Fig. 44.2). In these vessels, blood can flow in both directions as function of hemodynamic and metabolic needs.8 These arteriolar anastomoses often connect the anterior cerebral artery with the superior divisions of the middle cerebral
artery (MCA) and the posterior cerebral artery with the inferior divisions of the MCA. Furthermore, the venous collateral circulation is able to accommodate large volumes of flow diversion when an occlusion of a principal arterial route ensues (Fig. 44.3).
artery (MCA) and the posterior cerebral artery with the inferior divisions of the MCA. Furthermore, the venous collateral circulation is able to accommodate large volumes of flow diversion when an occlusion of a principal arterial route ensues (Fig. 44.3).
Diagnostic Evaluation Strategies and Recommendations
Conventional cerebral angiography remains the standard method to evaluate cerebral collateral circulation. It provides excellent temporal and spatial resolution of collateral vessels (Fig. 44.4).1 It allows assessment of extra- and intracranial anastomoses and Willisian and leptomeningeal collaterals.9 Variation in contrast volume and pressure during injection may distort the appearance of distal vessels. Incomplete information regarding collaterals is also obtained unless multivessel injections are performed. This technique is invasive and not routinely used to assess collateral blood flow.
Noninvasive methods such as CT and MR angiography can provide detailed anatomical image of the intracranial vessels. Time-of-flight MRA (TOF-MRA) can provide information about the amount of flow in a vessel, with limited information about anatomical details. Phase-contrast MRA provides unique data on not just the amount but also the direction of blood flow in large vessels. Contrast-enhanced MRA techniques provide better characterization of the luminal anatomy or degree of stenosis.
These vascular imaging studies demonstrate occlusion or blockage of a feeding artery to the brain in the setting of acute ischemic stroke. Nevertheless, the evaluation of collateral flow patterns may be equally important.1 CT angiography can be used to assess collateral circulation,10,11,12 provides rapid detailed images of the intracranial vessels, and its source images may contain valuable information regarding collaterals.13 Nevertheless, its inferior temporal resolution can result on overestimation of the collateral flow.6 MR can show hyperintense vessels on fluid-attenuated inversion recovery in patients with acute stroke due to intracranial artery thrombosis.
One study showed that patients with prominent distal hyperintense vessels had smaller ischemic lesion volumes and lower initial National Institutes of Health Stroke Scale (NIHSS) scores than patients with no distal hyperintense vessels.14 TOF-MRA underestimates the presence of collaterals, but use of contrast improves this limitation.15 Transcranial Doppler (TCD) ultrasound has been used to study collateral circulation in patients with spontaneous carotid artery dissection.16 Flow through the ophthalmic, anterior, and posterior communicating arteries was measured. Patients with good collaterals had better modified Rankin scale at 90 days than patients with poor collaterals. Nevertheless, TCD only provides information of collateral flow at the COW among other limitations of the technique. Perfusion techniques should not be directly compared with angiographic modalities because each technique has specific advantages and limitations. Moreover, timing of studies is crucial because collateral evolution is inherently a dynamic process. The best therapeutic planning is achieved when all potential collateral flow is evaluated with objective scales, rather than the presence or absence of specific arterial segments.1
One study showed that patients with prominent distal hyperintense vessels had smaller ischemic lesion volumes and lower initial National Institutes of Health Stroke Scale (NIHSS) scores than patients with no distal hyperintense vessels.14 TOF-MRA underestimates the presence of collaterals, but use of contrast improves this limitation.15 Transcranial Doppler (TCD) ultrasound has been used to study collateral circulation in patients with spontaneous carotid artery dissection.16 Flow through the ophthalmic, anterior, and posterior communicating arteries was measured. Patients with good collaterals had better modified Rankin scale at 90 days than patients with poor collaterals. Nevertheless, TCD only provides information of collateral flow at the COW among other limitations of the technique. Perfusion techniques should not be directly compared with angiographic modalities because each technique has specific advantages and limitations. Moreover, timing of studies is crucial because collateral evolution is inherently a dynamic process. The best therapeutic planning is achieved when all potential collateral flow is evaluated with objective scales, rather than the presence or absence of specific arterial segments.1
Imaging of Collateral Perfusion
The functional adequacy of the cerebral collaterals to preserve brain perfusion is better determined by techniques that can measure brain perfusion. Numerous techniques, including xenon-enhanced CT, single photon emission CT, positron emission tomography (PET), CTP, and MRP, assess tissue cerebral blood flow and thereby infer the status of collaterals.1
Perfusion imaging with CTP or MRP has become a routine tool for evaluation of patients with ischemic stroke. Cerebral hypoperfusion can be inferred when there is a mismatch between clinical neurologic deficits and CT or diffusion-weighted imaging (DWI) on MRI. Furthermore, evidence of hypoperfusion states is documented by delay in time parameters that reflect cerebral blood flow (CBF) or cerebral blood volume (CBV) within a region (Fig. 44.5). MRP/CTP imaging typically uses bolus tracking of intravascular contrast, as opposed to arterial spin labeling (ASL) techniques, which employ endogenous contrast
approaches by labeling the water protons in blood. The most commonly used perfusion parameters include CBF, CBV, and mean transit time (MTT). In the case of ASL, labeling of the patient’s blood in the proximal arteries is used to generate perfusion maps of the downstream circulation without the need of exogenous contrast.17 The detection of hypoperfusion lesions is not significantly different between the ASL and DSC approaches.18
approaches by labeling the water protons in blood. The most commonly used perfusion parameters include CBF, CBV, and mean transit time (MTT). In the case of ASL, labeling of the patient’s blood in the proximal arteries is used to generate perfusion maps of the downstream circulation without the need of exogenous contrast.17 The detection of hypoperfusion lesions is not significantly different between the ASL and DSC approaches.18
Delay in bolus arrival time, referred to as time-to-peak (TTP observed), delay in the maximum of the residue function (Tmax), and prolonged MTT can occur when perfusion is achieved via longer collateral routes (Fig. 44.6). For example, delay in TTP can be seen in scenarios such as internal carotid artery occlusion with collateral circulation through the COW.6 In acute ischemia, prolonged MTT identifies areas with decreased perfusion. Increased CBV within these regions indicates the presence of preserved collateral circulation, and it corresponds to areas of penumbra, while areas with decreased CBV correspond to the infarct tissue (Fig. 44.7). Similar to CTP, quantification of diffusion-weighted images and perfusion-weighted images can also identify ischemic penumbra.19,20,21
Potential limitations of perfusion imaging techniques include poor signal-to-noise ratio, timing errors due to decreased cardiac output, patient motion, and permeability changes in the blood–brain barrier. Perhaps the most significant challenge in perfusion imaging with either CT or MRI is the variability of results produced by different postprocessing software packages.22
Collateral Recruitment, Maintenance, and Impact on Tissue Fate
Collateral Circulation in Acute Arterial Occlusion
The most promising use of perfusion techniques in acute ischemic stroke is to identify areas of penumbra to select patients for thrombolytic therapy.23,24,25,26,27,28 When an acute arterial occlusion occurs, collateral circulation constitutes the main network to maintain cerebral perfusion. The process of collateral recruitment depends on the caliber and patency of primary pathways that may rapidly compensate for decreased blood flow and the adequacy of secondary collateral routes. Primary collaterals provide immediate diversion of cerebral blood flow to ischemic regions through existing anastomoses. Secondary collaterals, such as leptomeningeal anastomoses, may be anatomically present, although enhanced capacity of these alternative routes for cerebral blood flow likely requires time to develop. (Note that lacking time to develop such anastomoses is one of major limitations in animal models where vessels in otherwise healthy animals are simply ligated.) The drop in perfusion pressure distal to the occluded vessel generates an immediate pressure gradient between neighboring arterial territories that changes the flow direction within 1 to 4 seconds.29 Changes in intraluminal pressure also result in relaxation of smooth muscle cells, vasodilation, and a drop of vascular resistance. This facilitates blood flow to ischemic tissue, as long as systolic blood pressure is maintained at or above 50 mm Hg; otherwise, the collateral circulation fails.30,31 Blood pressure fluctuations may influence the patency of collaterals. Similarly, distal fragmentation of a thrombus may occlude distal branches supplying retrograde collateral flow from cortical arteries. Furthermore, the collateral vessels may also facilitate clearance of a fragmented thrombus from more proximal locations.32 Opening of collaterals likely depends on several compensatory hemodynamic, metabolic, and neural mechanisms, and its persistence is not guaranteed. Efficacy of collateral vessels likely depends on age, duration of ischemia, and associated comorbidities.1
Collateral Circulation in Chronic Arterial Occlusion
Chronic hypoperfusion due to extra- or intracranial arterial occlusion develops collateral circulation. Nevertheless, the relationship with clinical symptomatology remains unclear. Leptomeningeal collaterals are presumed to be developed when the COW has failed. The presence of these secondary collateral pathways may indicate impaired hemodynamics.1 Increasing severity of carotid stenosis has been correlated with a greater extent of collateralization. Van Everdingen et al.33 studied collateral pathways in patients with angiography proven unilateral carotid occlusion. The study showed that different collateral flow patterns via the anterior communicating artery, posterior communicating artery, or ophthalmic artery have no effect on the hemodynamics as long as one of these pathways is present. Other studies attempting to correlate collateral pathways with metabolic parameters have yielded conflicting results.34,35
Collateral circulation may play an important role in modifying stroke risk in the presence of carotid stenosis. In a study of 30 patients, cases with unilateral or bilateral extra- and intracranial occlusive disease only resulted in a severe hemispheric hemodynamic compromise if the COW was not intact.36 In patients with chronic carotid artery occlusion, the most likely mechanisms of stroke are embolic, hypoperfusion, or a combination of these mechanisms.32 Kamath et al.37 studied the correlation between CTP and PET in patients with chronic cervical carotid artery occlusion. It was determined that MTT has the best correlation with PET oxygen extraction fraction. In the North American Symptomatic Carotid Endarterectomy Trial, the presence of collateral circulation increased with progressive degrees of carotid stenosis.38 The adjusted risk of hemispheric stroke was significantly lower in medically treated patients with collaterals compared with those without collaterals. This benefit was observed independently of the degree of stenosis. In a post hoc analysis of the Asymptomatic Carotid Atherosclerosis Study, it was found that patients with contralateral internal carotid artery occlusion were actually protected from ischemic events.39 Although collateral circulation was not directly measured, the authors speculate that the presence of collaterals may have been a potential cause of this result. Patients with decreased cerebrovascular reserve are at a greater risk of stroke and have greater dependence on collaterals. Evaluation of the cerebral collateral circulation may help stratify the risk of therapeutic measures in patients with chronic cerebrovascular disease. In summary, the outcome of acute or chronic cerebrovascular occlusive disease likely depends on multiple variables, such as degree of luminal impairment, time course, and, most importantly, the presence and sustainability of the collateral circulation.
Validity of Animal Models: Lack of Chronic Stenosis and Collaterals
The advantage of animal models for the study of collateral circulation is the possibility of performing experiments in a controlled setting to study the myriad variables that can
influence collateral circulation. These models offer the possibility of measuring different biologic parameters that are not accessible in humans and to correlate in vivo measurements with postmortem brain evaluation. Frequent animal models that are used include rats, monkeys, cats, and dogs. Nevertheless, there are several differences in this area between humans and animals (i.e., single anterior cerebral artery in rats and monkeys as well as a rete mirabile caroticum connecting the internal carotid artery to arteries on the surface of the brain in cats and rabbits).8
influence collateral circulation. These models offer the possibility of measuring different biologic parameters that are not accessible in humans and to correlate in vivo measurements with postmortem brain evaluation. Frequent animal models that are used include rats, monkeys, cats, and dogs. Nevertheless, there are several differences in this area between humans and animals (i.e., single anterior cerebral artery in rats and monkeys as well as a rete mirabile caroticum connecting the internal carotid artery to arteries on the surface of the brain in cats and rabbits).8
Early animal studies were performed to understand the compensatory capacity of collateral circulation in cerebrovascular disorders. They determine the importance of systemic blood pressure to maintain flow through the anastomosis29,30,40 and the pressure gradient from healthy to occluded artery territories. It was shown that during MCA occlusion, there was a shift in the anterior cerebral artery and PCA territories that was dependent on the baseline vessel diameter,41 and there was increased flow in leptomeningeal collaterals associated with hypertension.42 A number of factors influence collateral circulation: diameter and length of leptomeningeal collaterals,43 age of the animal,44 cerebral edema,45 and the type of experimental occlusion.46 The efficient collateral blood supply delays ischemic injury47 and can also facilitate clearance of distal thrombus.48 There is much variation in the collateral vessels’ density and diameter and in their capacity to enlarge during ischemia. Permanent MCA occlusion in mice has shown that infarction size is significantly smaller in inbred mice with extensive collateral vessels than in those with fewer collaterals. Infarct volume was inversely correlated with collateral number, diameter, and penetrating arteriole number.49 Furthermore, vascular endothelial growth factor plays an important role in the development of collateral circulation.50 Mice with low vascular endothelial growth factor had a twofold larger infarct after MCA occlusion.
In addition to rapid responses to arterial occlusion, slower compensatory responses have been demonstrated. Internal carotid artery occlusion on a rat model showed that the PCA enlarged by 39% after 1 week and by 72% after 3 weeks. This enlargement was associated with the appearance of immunohistochemical markers of arteriogenesis.51 Similarly, other experimental models have shown that basilar-carotid anastomoses enlarged over a period of 6 weeks after carotid artery occlusion.52
In summary, the efficacy of collateral circulation in animal models depends on systemic blood pressure and metabolism. The adaptation of collateral circulation plays an important role on sustaining penumbra.
Endurance and Quality of Collateral Perfusion and Prediction of Sustenance of Penumbra
The knowledge that collateral circulation strongly influences stroke outcome has had only limited therapeutic applications. The main measure to improve collateral circulation remains the management of blood pressure to potentially increase perfusion pressure. Permissive hypertension for 24 hours is commonly employed in stroke units after onset of acute ischemic stroke. Furthermore, a selected group of patients with documented cerebrovascular arterial occlusion and good collateral circulation manifests as smaller than expected DWI changes on MRI or radiologic–clinical mismatch, and blood pressure may even be pharmacologically increased (Fig. 44.8). Other techniques such as aortic occlusion for cerebral ischemia have been proposed for collateral circulation enhancement.53 The use of perfusion imaging to evaluate the status of collaterals in ischemic stroke has the potential to improve therapeutic measures.
Predicting Response to Endovascular Therapy and Risk to Hemorrhagic Transformation in Acute Stroke
From the physiologic standpoint, collateral perfusion is adequate when CBF and CBV are maintained within the territory of an occluded artery.
Patients with better leptomeningeal or pial collaterals have lower infarct volume, lower NIHSS scores, and lower discharge-modified Rankin scale scores.54 Christoforidis et al.54 studied if the extent of pial collaterals during acute thromboembolic stroke predicts clinical outcome and infarct volume following intra-arterial thrombolysis. It was found that infarct volume and discharge-modified Rankin scale scores were significantly lower for patients with better pial collaterals, regardless of whether they had complete or partial recanalization. Bang et al.55 studied the influence of baseline collateral flow on infarct growth using conventional angiography and MRP. It was shown that the degree of pretreatment collateral circulation and volume of diffusion–perfusion mismatch were correlated with infarct growth. The analysis revealed that pretreatment collateral grade was independently associated with infarct growth. Kim et al.56 developed a collateral grading system for patients with confirmed acute MCA occlusion to predict regional infarction. The score accurately predicted the extent and location of the cerebral infarction in patients with MCA occlusion and correlated with follow up NIHSS scores for patients who received thrombolysis, but not for control patients. In summary, collateral flow has a greater impact on ultimate infarct volume than time from symptom onset to treatment,54 and the pretreatment collateral status has been independently associated with infarct growth (Fig. 44.7).55
Influence of Venous Hemodynamics on Collateral Perfusion
The current focus of ischemic stroke treatment is heavily focused around revascularization of arterial inflow.
Nevertheless, critical pathophysiology may involve downstream resistance of venous outflow pathways. Maintenance of cerebral blood volume is key for the survival of tissue during ischemic stroke. Given the collapsible nature of the venous system, cerebral venous steal or diversion of blood volume to the periphery of the ischemic territory may occur during ischemic stroke.57 When the venous pressure equals the external tissue pressure, blood flow diversion (cerebral venous steal) is abolished. Increased venous pressure may recruit the collapsed vascular network and correct perifocal perfusion maldistribution. Conversely, increased pressure in the distal venous system may increase blood volume, which, in turn, may enhance tissue perfusion. Aortic occlusion for cerebral ischemia has been proposed for collateral circulation enhancement. This procedure may enhance collateral perfusion due to increased blood volume within the cerebral veins, elevating downstream venous tension in excess of critical closing pressures and diminishing downstream resistance.53 Nevertheless, an important component of enhancement of cerebral blood flow and prevention of collateral failure may be reduction of downstream vascular resistance through venous manipulation in addition to increasing upstream or arterial pressure.
Nevertheless, critical pathophysiology may involve downstream resistance of venous outflow pathways. Maintenance of cerebral blood volume is key for the survival of tissue during ischemic stroke. Given the collapsible nature of the venous system, cerebral venous steal or diversion of blood volume to the periphery of the ischemic territory may occur during ischemic stroke.57 When the venous pressure equals the external tissue pressure, blood flow diversion (cerebral venous steal) is abolished. Increased venous pressure may recruit the collapsed vascular network and correct perifocal perfusion maldistribution. Conversely, increased pressure in the distal venous system may increase blood volume, which, in turn, may enhance tissue perfusion. Aortic occlusion for cerebral ischemia has been proposed for collateral circulation enhancement. This procedure may enhance collateral perfusion due to increased blood volume within the cerebral veins, elevating downstream venous tension in excess of critical closing pressures and diminishing downstream resistance.53 Nevertheless, an important component of enhancement of cerebral blood flow and prevention of collateral failure may be reduction of downstream vascular resistance through venous manipulation in addition to increasing upstream or arterial pressure.
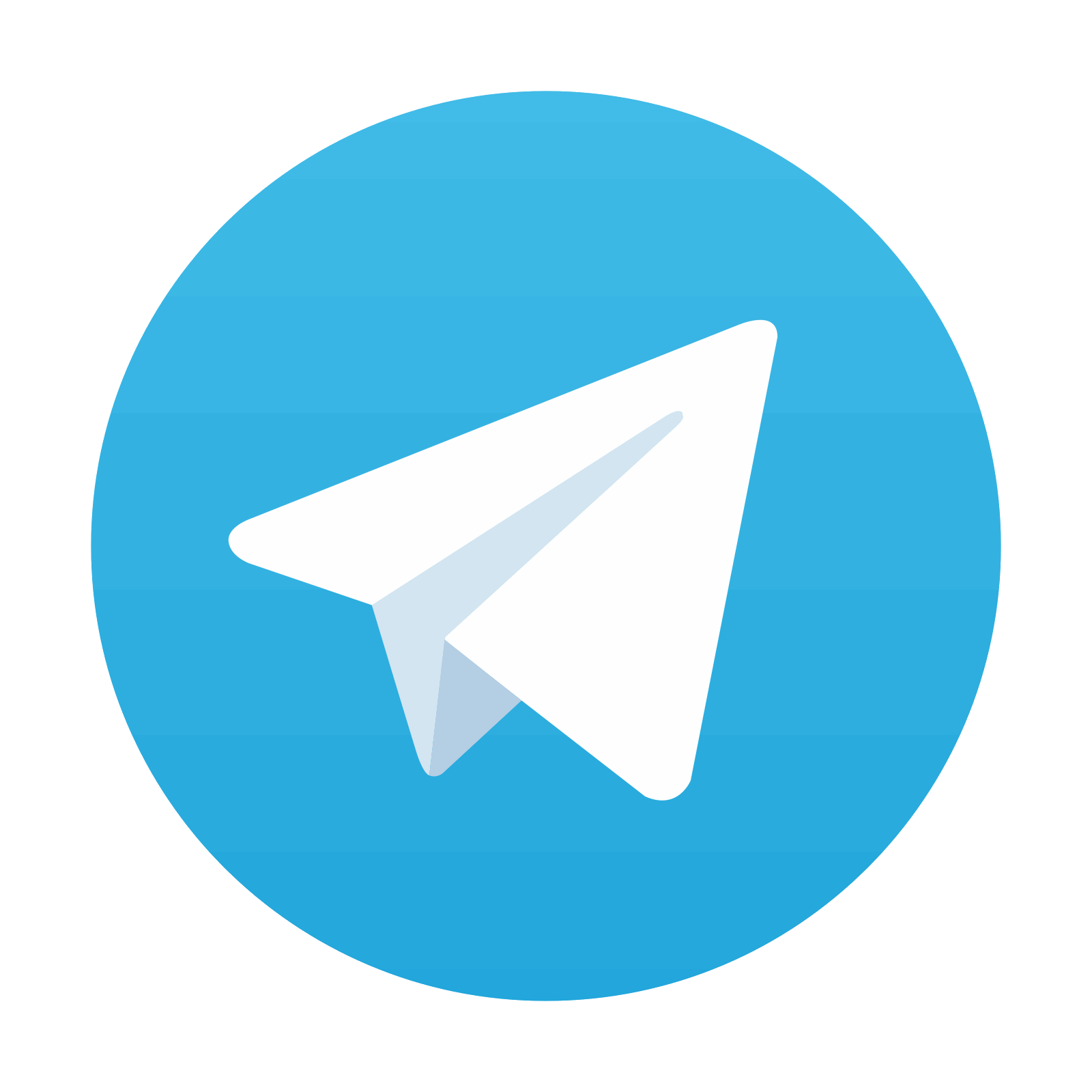
Stay updated, free articles. Join our Telegram channel

Full access? Get Clinical Tree
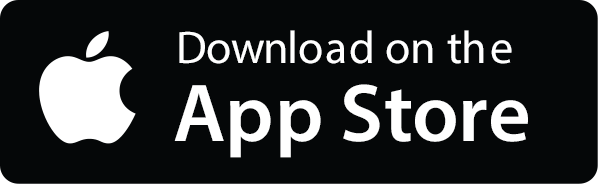
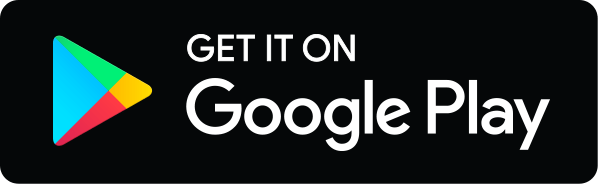