In 1895 the German physicist Wilhem Konrad Röntgen discovered X-rays. He was awarded the first Nobel Prize in physics in 1901 for this discovery. Medical imaging based on this technology developed rapidly, and the basic concept has not changed in a major way since that time, although the technology has. When an object is irradiated with photons generated in an X-ray tube they traverse the material and, depending on the intensity of the photon beam and the nature of the material imaged, some of the energy is lost (or attenuated) so that less energy emerges from the other side. Traditionally, this energy was used to ionize a photographic plate for creation of an image. Today, computers are used to digitally encode density and spatial information about portions of the object.
Computed tomography (CT) is a method of medical imaging that utilizes X-rays, or photons, to generate cross-sectional, two-dimensional images of the body. The X-ray tube is rotated around the patient for 360°. A fan of X-ray beams is created and each beam passes through the tissues of interest. The emerging beam is recorded by a ring of detectors on the opposite side of the rotating gantry. The beams are collimated using lead shutters to a thickness of 1–10 mm. As the X-ray tube and detectors make a 360° rotation numerous profiles of the attenuated beams are recorded. The final image is generated using data relating to the differential passage of the X-rays through different tissues. Multiple X-ray projections are used to reconstruct tissue structures based on complex mathematical algorithms.
The earliest CT scanners acquired images one slice at a time by sequentially moving the X-ray source and detectors across the body, then moving the body incrementally a small amount to acquire the next slice. Advances in technology during the 1980s and 1990s allowed the X-ray source and detector arrays to rotate continuously around the patient without having to stop or reverse direction. The most recent generation machines utilize a spiral or helical technology in which the X-ray source rotates continuously in one direction within a ring of detectors as the patient moves forward (along the head to toe axis) so that the X-ray beam describes a spiral path around the body. In this manner data are acquired as a continuous volume and in a very short period of time, thus reducing movement artifacts. The newest multislice or multidetector machines employ a technology with multiple detector rings that can acquire more than one slice per rotation. The volume is therefore scanned very quickly as this increases the volume of the body that can be imaged per unit time. This technique is particularly suited to CT angiography which relies on precise timing of image acquisition following intravenous contrast administration. Dual source CT imaging is a more recent technology that utilizes two X-ray sources and a dual array of slice detectors. This reduces the angle necessary to acquire a complete image, allowing imaging of moving structures such as the heart.
Each slice of a CT image is formed by the intersection of numerous X-ray beams passed through the body at different angles. The cross-sectional area defined by any two intersecting beams is referred to as a pixel , and is analogous to a single dot in a newspaper photograph ( Figure 1.1 ). Because the X-ray beam has a certain thickness, the area of beam intersection actually defines a volume of elements, referred to as the voxel . These intersecting beams create a matrix of up to 1024 × 1024 voxels . As the photons pass through tissues, they are attenuated, or weakened as described below, and the resultant energy is measured by the detectors. The computer then calculates the attenuation coefficient for each voxel based on the matrix data. A specific attenuation value is assigned to each voxel, and an image is then reconstructed to produce a two-dimensional matrix of pixels which form the picture elements.
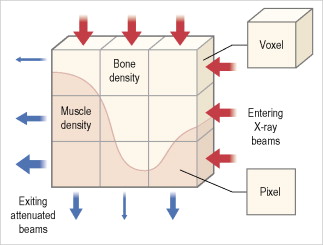
Physics of computed tomography
Photons are elementary particles responsible for electromagnetic radiation of all wavelengths. These include gamma rays, X-rays, ultraviolet light, visible light, infrared light, microwaves, and radio waves. Because the photon has no net mass, in a vacuum it travels at the speed of light. Photons can show wavelike characters, whereby they can be refracted by a lens, and they also act like particles in the manner by which they transfer energy to other particles.
An X-ray is one form of electromagnetic radiation with a wavelength in the range of 0.01–10 nm. This corresponds to a frequency of 30 PHz (Petahertz) to 30 EHz (Exahertz). For clinical radiology so-called ‘hard’ X-rays are used with a wavelength of 0.01–0.1 nm. They are longer than gamma rays but shorter than ultraviolet rays. X-rays are produced by accelerating electrons to collide with a metallic target (usually tungsten with a small amount of rhenium). The electrons decelerate upon initial collision, and if they have enough energy they will knock out an electron from the inner shell of the metallic atom. As electrons from the outer shell rush in to fill the void, X-rays are produced.
X-rays and gamma rays are a form of ionizing radiation with energy that is so high they can be regarded as a stream of single photons. Therefore, instead of using the wavelength, the energy of each photon is usually characterized with the formula:
E = h c / γ
where:
- •
E is energy measured as keV
- •
c is the speed of light
- •
h is Planck’s constant
- •
γ is the wavelength.
Because of their high energy, X-rays are a form of ionizing radiation. Ionizing radiation is highly energetic particles or waves that can detach, or ionize, an electron from an atomic molecule. X-rays lie on a spectrum from the very energetic gamma and X-rays on one end, which will ionize almost any molecule, to photons (visible light), which will ionize very few molecules (most notably photosynthetic chlorophyll), to radio waves which are non-ionizing.
X-rays interact with matter in several ways: photoelectric effect, Compton scattering, pair production, and Rayleigh scattering. For the energy levels used in clinical imaging, only the first two interactions predominate.
- •
The photoelectric effect is the complete absorption of a photon when it interacts with an atomic inner electron. This happens when the energy of the photon exceeds the binding energy of the electron. All of the energy of the photon is transferred to the electron and the latter is emitted from the atom ( Figure 1.2 ). At higher photon energies, more electrons participate in the interaction.
Figure 1.2
Photoelectric effect. The incoming X-ray photon is absorbed and an electron is emitted, thus attenuating the incoming X-ray beam.
- •
Compton scattering is a process whereby a photon of high energy interacts with an outer electron but is not absorbed. Instead, the photon transfers part of its energy, emitting the electron, but the photon is deflected in a different direction, that is, it is scattered ( Figure 1.3 ).
Figure 1.3
Compton scatter. The incoming X-ray photon is deflected and an electron is emitted, thus reducing or attenuating the X-ray beam that passes through the tissue.
As a result of the photoelectric effect and Compton scattering phenomena, when X-rays interact with tissue the photons either lose energy and scatter, or they are completely absorbed. Therefore, photons in a beam passing through the tissue will disappear with only those that do not interact at all emerging from the other side. The beam is therefore attenuated in intensity ( Figure 1.4 ).
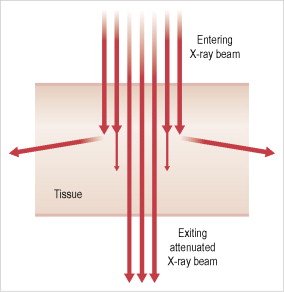
Attenuation is an important concept since it is the basis of CT contrast imaging. The probability of photon attenuation as it passes through a uniform tissue is expressed by the Beer equation:
I = I 0 e − μ x
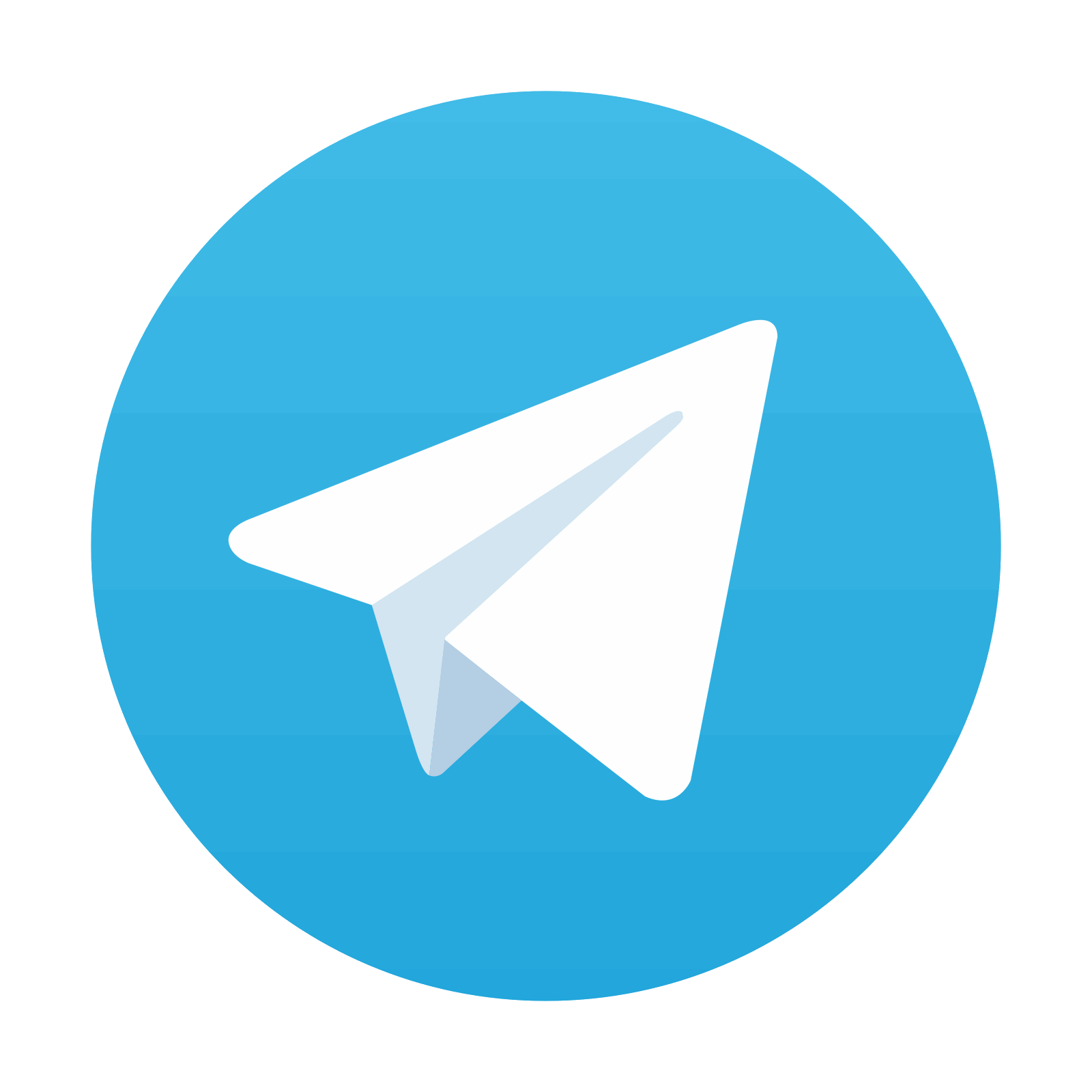
Stay updated, free articles. Join our Telegram channel

Full access? Get Clinical Tree
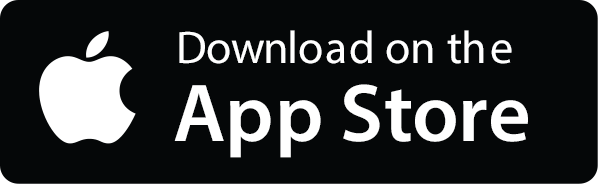
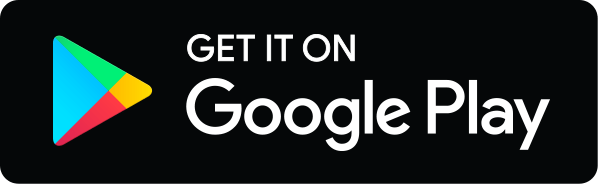