
polarized cellular protrusions that enable the cells to migrate medially and intercalate with neighboring cells close to the midline (3). This midline convergence of cells causes an anteroposterior elongation and narrowing of the neural plate (4,5). This process is strongly related to the development of cell polarity (4,5). Along with the process of shaping, the neural plate also bends. At approximately 17 days of gestation, the lateral portions of the neural plate begin to thicken bilaterally, forming the neural folds; the process of bending elevates these folds and brings them to the dorsal midline (6). The process involves the formation of “hinge points” at two sites: the median hinge point (MHP) in the ventral midline and extending over the rostrocaudal extent of the neural plate and the paired dorsolateral hinge points (DLHPs), which form mainly at the levels of the developing brain (2) and lower spine. The formation of these hinge points is controlled by secretion of the signal transduction protein sonic hedgehog by the notochord as well as an inhibitory interaction between BMP2 and Noggin, particularly in the lower spine (7). After formation of the hinge points, the more lateral aspects of the neural plate are elevated around the MHP, bringing the DLHPs upward and toward the midline (Fig. 9-2). This elevation is accomplished by a poorly understood process called apical constriction, in which columnar cells of the neural tube are converted into wedge-shaped cells (8). Eventually, the lateral folds contact one another in the dorsal midline and adhere to one another, with their fusion forming the neural tube (neurulation). This midline contact (also called neural fold apposition) results from constriction of the open posterior neural tube, which is biomechanically coupled to the zippering point by an F-actin network (9). Neurulation seems to begin separately at least two different levels in humans, when cellular protrusions (possibly cilia) project medially from the most dorsal cells of the neural folds on either side. A third site of closure at the caudal end of the embryo has recently been identified in mouse embryos; if present in humans, this may account for the high incidence of spina bifida at this level (9). Cell recognition and adhesion occur under the influence of many molecules (Ephrin-A5, EphA7, neural cell adhesion molecule, and neural cadherin among them (3)), closing the tube at each point. Immediately following closure, the overlying ectoderm separates from the neural tissue and the edges of the ectoderm meet in the midline and fuse, forming a continuous ectodermal covering of the neural structures, with the mesenchymal cells of the neural crest migrating between the cutaneous and neural ectoderm layers (2). Progressive folding and closure of the neural structures and separation from ectoderm then proceed both cranially and caudally from each point of initial closure, ultimately resulting in complete closure (10,11). The initial closure of the neural tube in humans, the posterior neuropore, is believed to be at the hindbrain-cervical junction, from which closure extends in both directions. A second site of closure in mice is at the forebrain-midbrain boundary; this site has not been confirmed in humans. The necessity of this site even in mice is questionable, as about 80% of mice that lack the second closure point still achieve complete cranial closure (12). The “third” site of closure occurs at the most rostral extremity of the forebrain, the lamina terminalis (2). The exact site of the most caudal end of the neurulation-formed neural tube has been debated, but most experts believe that it is at the S2 level (10,11). Others point out, however, that neural tube defects are not restricted to any specific location(s) and propose that the human neural tube initially closes at a single site with closures extending from that location (13).
we review these cellular, molecular, and biomechanical mechanisms involved in neural tube closure, based on studies of various vertebrate species, focusing on the most recent advances in the field.
![]() Figure 9-2 Normal and abnormal neurulation. A-E. Normal neurulation. The neural plate is composed of neural ectoderm, which is continuous with cutaneous ectoderm on either side. The cells at the junction of the neural ectoderm and cutaneous ectoderm will eventually differentiate into neural crest cells (A). At approximately 17 days, the lateral portions of the neural plate begin to thicken, forming the neural folds (B). Contractile filaments located in the neuroepithelial cells in the neural folds contract, causing the neural folds to bend dorsally along the entire length of the neuroaxis, bringing the edges of the neural folds toward one another in the midline (C). Neurulation (closure of the neural tube) begins when the neural folds meet in the midline. At the time of closure, the overlying ectoderm separates from the neural tissue and fuses in the midline, forming a continuous ectodermal covering of the neural structures. At the same time, the neural crest cells are extruded from the neural tube to form a transient structure immediately dorsal to the tube (D). Eventually, these neural crest cells will migrate to form dorsal root ganglia and multiple other structures (E). F-G. Abnormal neurulation. When there is premature disjunction of neural ectoderm from cutaneous ectoderm, the surrounding mesenchyme gains access to the inner surface of the neural tube. When mesenchyme comes in contact with this primitive ependymal lining, it evolves into fat. This is believed to be the process underlying the formation of spinal lipomas (F). Complete nondisjunction of cutaneous ectoderm from neural ectoderm results in the formation of myelomeningoceles (Fig. 9-4). Focal nondisjunction results in a persistent epithelium-lined connection between the central nervous system and the skin (G). This persistent connection has been labeled a dorsal dermal sinus. |
rests in the normal filum terminale and distal conus medullaris of the adult are believed to result from the disorder of this process. Several neuronal markers expressed in vertebrate embryos are thought to modulate the differentiation of structures derived from the caudal cell mass and be involved in the maturation of the caudal spinal cord. These include N-CAM, synaptophysin, 3A10, and NeuN (21). The final stage in the formation of the distal spinal cord begins at about 38 days of gestation, at which time the cell mass and central lumen of the caudal neural tube decrease in size as a result of programmed cell death (apoptosis) of the portion derived from primary neurulation (20) and necrosis of the portion derived from secondary neurulation (22). This process has been named retrogressive differentiation (Fig. 9-3). The caudal segment (formed by canalization and retrogressive differentiation) eventually becomes the most caudal portion of the conus medullaris, the filum terminale, and a focal dilation of the central canal (within the conus medullaris) known as the terminal ventricle (ventriculus terminalis) (23,24,25,26,27,28).
arch (31). Notochordal remnants persist between the newly formed vertebral bodies and become incorporated into the intervertebral discs as the nuclei pulposi. Portions of the thoracic sclerotomes later migrate ventrolaterally to form ribs. The anterior and posterior longitudinal ligaments form during chondrification, from mesodermal cells.


the simple dysraphic states (fatty and/or fibrous thickening of the filum terminale, intraspinal lipoma, spina bifida, and persistent terminal ventricle) and the complex dysraphic states (split-cord malformation, dorsal dermal sinus, caudal regression syndrome, segmental spinal dysgenesis, neurenteric cyst, and dorsal enteric fistula).
Table 9-1 Clinical-Radiological Classification System for Spinal Dysraphism | |||||||||||||||||||||||||||||||||
---|---|---|---|---|---|---|---|---|---|---|---|---|---|---|---|---|---|---|---|---|---|---|---|---|---|---|---|---|---|---|---|---|---|
|


common, in regions where these modalities are widely available. The ongoing prospective multi-institutional follow-up study will determine the long-term benefits of prenatal repair of this spinal anomaly on neurological and functional outcome.
of the spinal column at the affected level, by the posterior fossa contents extending caudally through the foramen magnum and, in many cases, by ventriculomegaly. In general, when the OSD is uncovered and opens to the amniotic fluid, hindbrain herniation is more severe because the rostrocaudal pressure gradient is increased (Fig. 9-5E-G). Early (prenatal) repair is very important in these cases to minimize these consequences.
malformation. The spinal cord may be split above (31%), below, (25%) or at the same level (22%) as the OSD (94). In addition to the patients with frank split-cord malformation, 5% of patients with OSD have a duplication of the central canals of the spinal cord cephalic to and at the level of the placode, indicating a mild form of splitting that is insufficient to affect the gross contour of the cord (94).
![]() Figure 9-9 Epidermoid tumor in a patient who underwent fetal myelomeningocele repair. A-D. The epidermoid (arrows) can be difficult to detect by routine sagittal and axial T1 and T2 MR sequences. E. Sagittal FLAIR with fat suppression shows the epidermoid clearly (arrow). Diffusion imaging can also be valuable (see Chapter 7). |
brain stem is pulled/stretched inferiorly and narrowed in the anteroposterior diameter, often lying at the level of the foramen magnum or in the cervical spinal canal. The cervical spinal cord is displaced inferiorly and the upper cervical nerve roots have to ascend toward their respective neural foramina. The medulla is also displaced inferiorly. In 70% of patients, it folds caudally at the cervicomedullary junction, dorsal to the cervical spinal cord (which is tethered by the dentate ligaments and therefore limited in its vertical descent), forming a characteristic cervicomedullary kink (Figs. 5-178 and 5-179). The cerebellar vermis often herniates inferiorly, forming a tongue of tissue posterior to the medulla that usually extends down to the C2 or C4 level. Rarely, it extends down into the upper thoracic segments of the canal. The cerebellum wraps around the brain stem (Fig. 5-179). The fourth ventricle is vertical in orientation (Figs. 5-178 and 5-179), extending inferiorly between the medulla and cerebellar vermis; occasionally, it extends down below the medulla, posterior to the cervical spinal cord, in a cyst-like fashion. The quadrigeminal plate is stretched posteriorly and inferiorly (Figs. 5-178 and 5-179). The small posterior
fossa and the downward herniation of its contents result in a low-lying, abnormally vertical tentorium cerebelli (115,116,117,118). Many of these changes improve or completely resolve after fetal repair of the OSD, as discussed in Chapter 5.
diffusion-weighted images can be very helpful in this setting (135,136); they will guide the surgeon to remove as much tumor as possible.
an entity distinct from the myelocystocele. The latter by definition should be an encysted spinal cord. Other authors differentiate cervical myelocystoceles from cervical myeloceles, but consider the latter to be meningoceles (67). However, meningoceles by definition do not contain neural tissue and the cervical myeloceles clearly have a stalk of neural tissue extending through the spinal bifida, as shown by Rossi et al. (141). Cervical (nonterminal) myelocystoceles should also be differentiated from terminal myelocystoceles, which are anomalies of the caudal cell mass and are located at the lumbosacral level. Terminal myelocystoceles are discussed later in this chapter.
motor deficit is usually present, often requiring orthopedic intervention (143). The head size is often enlarged (67).
much greater near the interface of the cord and lipoma and considerably less near the skin surface (130,146). Calcification and ossification are sometimes seen (130,147), as are muscle fibers, nerves, glial tissue, arachnoid, ependyma, and many other types of tissue (148).
the fat. The faulty disjunction between neural ectoderm and cutaneous ectoderm that results in the formation of spinal lipomas may also explain the frequent association of lipomas with dorsal dermal sinuses, which result from a focal area of faulty disjunction.
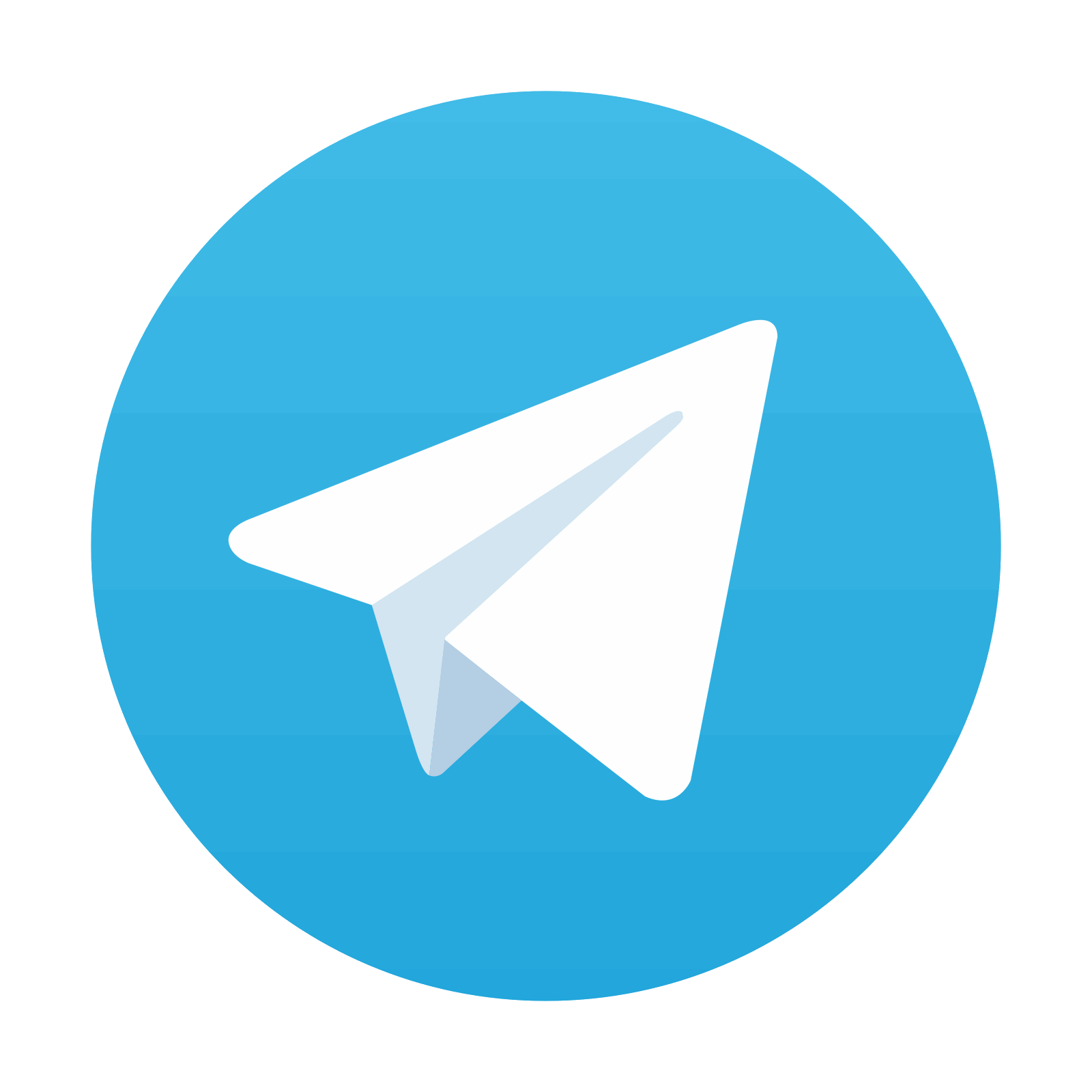
Stay updated, free articles. Join our Telegram channel

Full access? Get Clinical Tree
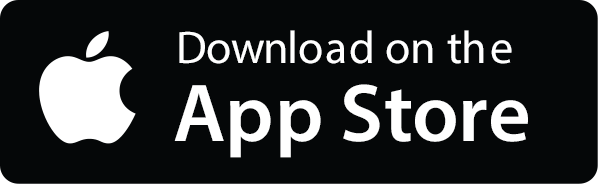
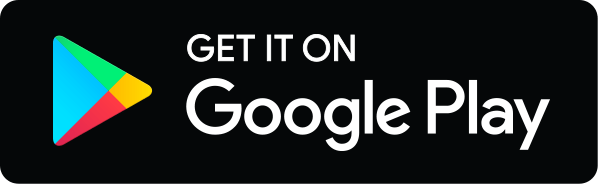
