MR imaging without and with gadolinium-based contrast agents (GBCAs) is an important imaging tool for defining normal anatomy and characteristics of lesions. GBCAs have been used in contrast-enhanced MR imaging in defining and characterizing lesions of the central nervous system for more than 20 years. The combination of unenhanced and GBCA-enhanced MR imaging is the clinical gold standard for the noninvasive detection and delineation of most intracranial and spinal lesions. MR imaging has a high predictive value that rules out neoplasm and most inflammatory and demyelinating processes of the central nervous system.
- •
Combined MR imaging without and with gadolinium-based contrast agents (GBCAs) is an important imaging tool for defining normal anatomy and characteristics of lesions.
- •
GBCAs have been used in contrast-enhanced (CE) MR imaging in defining and characterizing both cranial and spinal lesions of the central nervous system (CNS) for more than 20 years.
- •
Normally, the blood-brain barrier (BBB) prevents GBCAs from crossing from the blood to the brain.
- •
The combination of unenhanced and GBCA-enhanced MR imaging is the clinical gold standard for the noninvasive detection and delineation of most intracranial and spinal lesions.
- •
MR imaging has a high predictive value that reliably rules out neoplasm and most inflammatory and demyelinating processes of the CNS.
Introduction
MR imaging is a proved and well-established imaging modality in the evaluation and assessment of normal and abnormal conditions of the brain with superior accuracy compared with other imaging modalities. With intravenous administration of GBCAs, conventional CE MR imaging has been a valuable method for visualization of lesions with breakdown of the BBB, with improved sensitivity and specificity. At present, there are 7 GBCAs approved in the United States or the European Union for use in the CNS ( Table 1 ). Most available GBCAs that shorten the T1 relaxation time to enhance MR images are low-molecular-weight polyaminocarboxylate compounds. All these GBCAs share low rates of qualitatively similar adverse drug reaction in surveillance studies. The standard dose of GBCA for most MR imaging applications is 0.1 mmol/kg body weight; although it is proved that higher doses can improve lesion detection.
Generic Name | Product/Trade Name | Manufacturer | Formulation | Concentration (mol/L) | Serum Albumen Binding |
---|---|---|---|---|---|
Gadopentetate dimeglumine | Magnevist | Bayer Healthcare | Ionic linear | 0.5 | No |
Gadoterate meglumine | Dotarem | Guerbet | Macrocyclic | 0.5 | No |
Gadodiamide | Omniscan | GE Healthcare | Nonionic linear | 0.5 | No |
Gadoteridol | ProHance | Bracco Diagnostics | Macrocyclic | 0.5 | No |
Gadobutrol | Gadovist | Bayer Healthcare | Macrocyclic | 1 | No |
Gadoversetamide | Optimark | Mallinckrodt | Nonionic linear | 0.5 | No |
Gadobenate disodium | MultiHance | Bracco Diagnostics | Ionic linear | 0.5 | Weak |
GBCAs can be classified on the basis of ionicity (ionic vs nonionic), biochemical structures (macrocyclic vs linear), or binding to serum albumin (see Table 1 ).
Effect of GBCAs on MR sequences on neuroimaging
In clinical practice, CE MR imaging is performed with either spin-echo or gradient-echo (GRE) T1-weighted (T1W) MR imaging sequences, and there has been controversy regarding which sequence is better for use for CE MR imaging in neuroimaging. Spin-echo images have been the preferred sequence due to better contrast enhancement of the brain lesions. GRE sequences, alternatively, provide improved spatial resolution in reduced imaging time and reduced motion artifact; thus, small neoplastic lesions may be better visualized in thin-slice 3-D GRE images. When it comes to 3-T MR, in which the T1 relaxation times of gray and white matter tend to become longer, T1 contrast of the brain on sequences without contrast administration is reduced, and specific absorption rate limitation matters. Therefore, opportunity to use the GRE sequence may be increased in clinical examination at 3 T. Although there have been several reports that GRE cannot surpass SE imaging for detecting brain lesions because of inherently weaker enhancement, GRE at 3 T with administration of high relaxavity GBCAs, high doses of gadolinium, and suitable scanning delay time improves diagnostic accuracy of brain tumor. Recent studies that used 3-D T1 turbo spin-echo sequence with variable flip angle echo train in reasonable imaging duration and specific absorption rate limit showed higher enhancement brain metastasis than 3-D GRE.
Contrast enhancement is also seen on fluid-attenuated inversion recovery (FLAIR) brain MR imaging after intravenous injection of GBCA. Contrast enhancement on FLAIR imaging is the result of a mild T1 effect, produced by the long inversion time used to nullify the signal intensity of water. As in CE T1W imaging, choroid plexus, pituitary stalk, pineal gland, and dural sinuses show normal enhancement on CE FLAIR MR imaging. Degree of contrast enhancement in normal intracranial structures on CE FLAIR is less intense than that on CE T1W imaging, because of a mild T1 effect on the FLAIR sequence. Alternatively, most blood vessels do not enhance on CE FLAIR, probably due to a T2 effect of the FLAIR sequence. Thus, CE FLAIR is useful in depicting the superficial abnormalities, such as leptomeningeal inflammation or carcinomatosis, cranial nerve lesion, and inner ear abnormalities ( Fig. 1 ). CE FLAIR is also suggested as having a complementary role for detecting intracranial tumors and demyelinating disease. In stroke imaging, vascular hyperintensity on CE FLAIR in patients with acute ischemic stroke, intracranial stenosis, moyamoya disease, and transient ischemic attack represents disordered blood flow from collaterals distal to the arterial occlusion or stenosis (not an indicator of infarction but rather of tissue at risk for infarction).
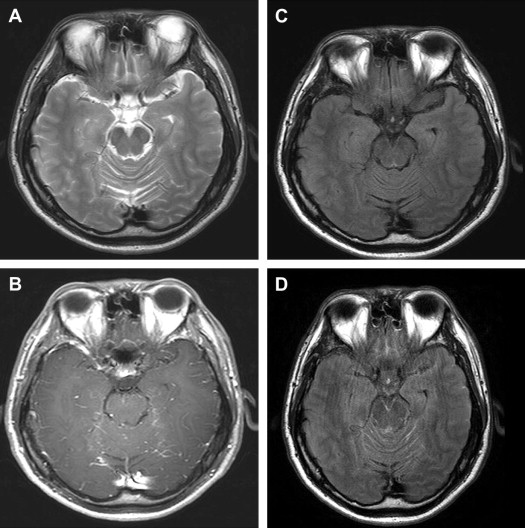
Susceptibility-weighted imaging (SWI) is sensitive sequence for parenchymal bleed and calcifications, allows noninvasive visualization of small veins in the brain, and can be used to depict venous architecture in normal and pathologic tissues. In SWI images, veins appear hypointense due to the presence of deoxyhemoblobin and the arteries are hyperintense due to time-of-flight effects and lack of T2* effects, giving natural separation of arteries and veins. SWI can be performed after gadolinium has been injected, without loss or signal change in the parenchyma ( Fig. 2 ). CE SWI is useful in the evaluation of arteriovenous shunt in patients with brain vascular malformation, in which presence of high signal intensity within the venous structure is an accurate indicator of arteriovenous shunt. CE SWI can also visualize the architecture of brain neoplasm, demonstrating the leakage of contrast material due to breakdown of the BBB.
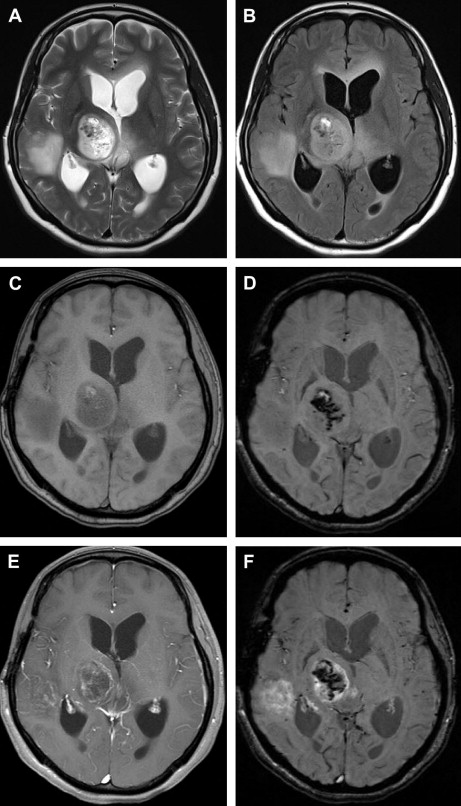
Diffusion-weighted imaging (DWI) has become a powerful tool in the evaluation of brain lesions and mainly used in assessment of acute stroke, but it has become widely used in the assessment of tumor, infection, inflammation, and trauma. DWI after administration of contrast agent may be necessary in circumstances with motion of the head, especially in acute stroke. DWI can be obtained after contrast administration of GBCA without causing a significant change in visual evaluation of normal and infarcted brain. Apparent diffusion coefficient (ADC) values decrease, however, after contrast agent injection on early CE DWI in normal brain tissue (1%) and brain lesions (3%–6%). Thus, if ADC measurements are planned, DWI must be performed after an acceptable delay after contrast medium injection of at least 6 to 10 minutes.
Magnetic resonance spectroscopy (MRS) is increasingly used in neuroimaging, including evaluation of brain tumor. Magnetic resonance spectra of brain tumors show elevated levels of Cho peak and decreased levels of N-acetyl aspartate (NAA) peak relative to normal brain, reflecting increased cellularity. MRS is helpful in differentiation of brain tumors and characterization of metabolic changes associated with tumor progression, degree of malignancy, and response to treatment. In some cases, need for MRS may not be determined until after contrast agent had been administered, and the placement of region of interest at the enhancing part of the tumor may be more valuable than that of remaining nonenhancing part. Sijens and colleagues reported a decrease in the Cho peak after administration of gadolinium contrast that lasted for nearly 5 minutes, and with little further change with time. The presence of contrast material, however, after 5 to 10 minutes of administration does not alter the diagnostic quality of MRS in brain tumors. Manual reshimming the gradients immediately before obtaining the postcontrast spectra might further decrease any effect of magnetic field changes by gadolinium.
Effect of GBCAs on MR sequences on neuroimaging
In clinical practice, CE MR imaging is performed with either spin-echo or gradient-echo (GRE) T1-weighted (T1W) MR imaging sequences, and there has been controversy regarding which sequence is better for use for CE MR imaging in neuroimaging. Spin-echo images have been the preferred sequence due to better contrast enhancement of the brain lesions. GRE sequences, alternatively, provide improved spatial resolution in reduced imaging time and reduced motion artifact; thus, small neoplastic lesions may be better visualized in thin-slice 3-D GRE images. When it comes to 3-T MR, in which the T1 relaxation times of gray and white matter tend to become longer, T1 contrast of the brain on sequences without contrast administration is reduced, and specific absorption rate limitation matters. Therefore, opportunity to use the GRE sequence may be increased in clinical examination at 3 T. Although there have been several reports that GRE cannot surpass SE imaging for detecting brain lesions because of inherently weaker enhancement, GRE at 3 T with administration of high relaxavity GBCAs, high doses of gadolinium, and suitable scanning delay time improves diagnostic accuracy of brain tumor. Recent studies that used 3-D T1 turbo spin-echo sequence with variable flip angle echo train in reasonable imaging duration and specific absorption rate limit showed higher enhancement brain metastasis than 3-D GRE.
Contrast enhancement is also seen on fluid-attenuated inversion recovery (FLAIR) brain MR imaging after intravenous injection of GBCA. Contrast enhancement on FLAIR imaging is the result of a mild T1 effect, produced by the long inversion time used to nullify the signal intensity of water. As in CE T1W imaging, choroid plexus, pituitary stalk, pineal gland, and dural sinuses show normal enhancement on CE FLAIR MR imaging. Degree of contrast enhancement in normal intracranial structures on CE FLAIR is less intense than that on CE T1W imaging, because of a mild T1 effect on the FLAIR sequence. Alternatively, most blood vessels do not enhance on CE FLAIR, probably due to a T2 effect of the FLAIR sequence. Thus, CE FLAIR is useful in depicting the superficial abnormalities, such as leptomeningeal inflammation or carcinomatosis, cranial nerve lesion, and inner ear abnormalities ( Fig. 1 ). CE FLAIR is also suggested as having a complementary role for detecting intracranial tumors and demyelinating disease. In stroke imaging, vascular hyperintensity on CE FLAIR in patients with acute ischemic stroke, intracranial stenosis, moyamoya disease, and transient ischemic attack represents disordered blood flow from collaterals distal to the arterial occlusion or stenosis (not an indicator of infarction but rather of tissue at risk for infarction).
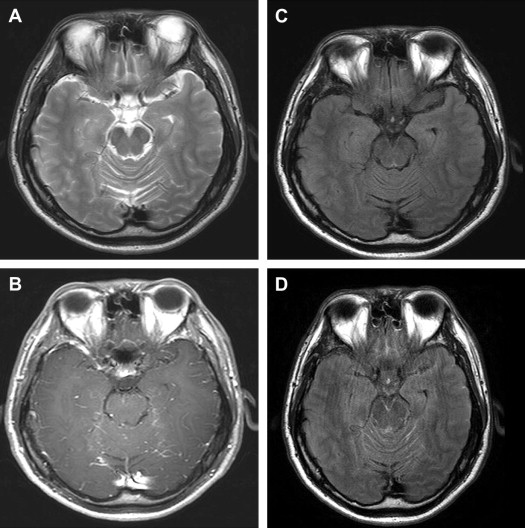
Susceptibility-weighted imaging (SWI) is sensitive sequence for parenchymal bleed and calcifications, allows noninvasive visualization of small veins in the brain, and can be used to depict venous architecture in normal and pathologic tissues. In SWI images, veins appear hypointense due to the presence of deoxyhemoblobin and the arteries are hyperintense due to time-of-flight effects and lack of T2* effects, giving natural separation of arteries and veins. SWI can be performed after gadolinium has been injected, without loss or signal change in the parenchyma ( Fig. 2 ). CE SWI is useful in the evaluation of arteriovenous shunt in patients with brain vascular malformation, in which presence of high signal intensity within the venous structure is an accurate indicator of arteriovenous shunt. CE SWI can also visualize the architecture of brain neoplasm, demonstrating the leakage of contrast material due to breakdown of the BBB.
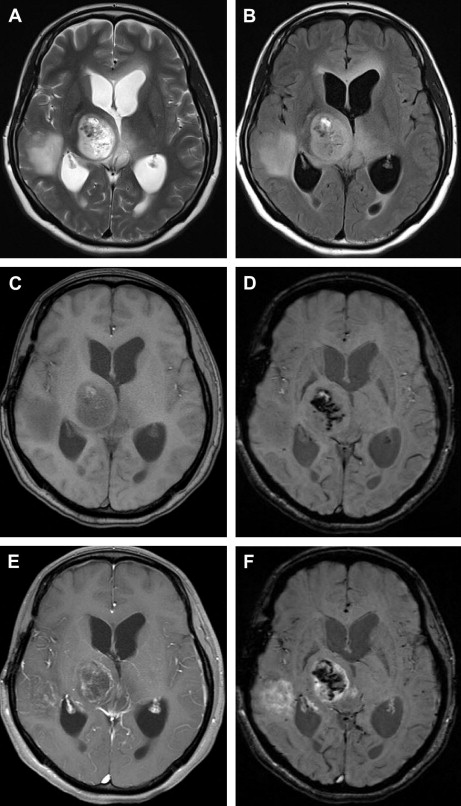
Diffusion-weighted imaging (DWI) has become a powerful tool in the evaluation of brain lesions and mainly used in assessment of acute stroke, but it has become widely used in the assessment of tumor, infection, inflammation, and trauma. DWI after administration of contrast agent may be necessary in circumstances with motion of the head, especially in acute stroke. DWI can be obtained after contrast administration of GBCA without causing a significant change in visual evaluation of normal and infarcted brain. Apparent diffusion coefficient (ADC) values decrease, however, after contrast agent injection on early CE DWI in normal brain tissue (1%) and brain lesions (3%–6%). Thus, if ADC measurements are planned, DWI must be performed after an acceptable delay after contrast medium injection of at least 6 to 10 minutes.
Magnetic resonance spectroscopy (MRS) is increasingly used in neuroimaging, including evaluation of brain tumor. Magnetic resonance spectra of brain tumors show elevated levels of Cho peak and decreased levels of N-acetyl aspartate (NAA) peak relative to normal brain, reflecting increased cellularity. MRS is helpful in differentiation of brain tumors and characterization of metabolic changes associated with tumor progression, degree of malignancy, and response to treatment. In some cases, need for MRS may not be determined until after contrast agent had been administered, and the placement of region of interest at the enhancing part of the tumor may be more valuable than that of remaining nonenhancing part. Sijens and colleagues reported a decrease in the Cho peak after administration of gadolinium contrast that lasted for nearly 5 minutes, and with little further change with time. The presence of contrast material, however, after 5 to 10 minutes of administration does not alter the diagnostic quality of MRS in brain tumors. Manual reshimming the gradients immediately before obtaining the postcontrast spectra might further decrease any effect of magnetic field changes by gadolinium.
Contrast enhancement in normal CE MR imaging
Most of brain is not enhanced with administration of contrast agent because the BBB prevents the contrast from reaching the cerebral extravascular space. The pituitary gland and stalk, choroid plexus, and intracavernous portions of cranial nerves III–VI, which have an incomplete BBB, enhance greatly ( Fig. 3 ). Enhancement in these structures persists for 1 hour but is usually maximal or nearly maximal at 3 minutes. Also, the mucosal surfaces of the nasopharynx and sinuses, slowly flowing blood, and the retinal choroid all show prominent contrast enhancement after administration of a magnetic resonance contrast agent. The dura mater, which usually shows enhancement of the falx and tentorium on CE CT images, does not routinely demonstrate similar enhancement on MR images. Although normal dura mater does not have a BBB, it lacks sufficient water to show the T1 shortening required for enhancement on MR images. Meningeal enhancement, alternatively, has been seen in the pathologic conditions, including leptomeningeal metastases, bacterial and granulomatous meningitis, and after intracranial surgery. Also visualized is the enhancement of normal superficial venous structures, although this may be both variable and asymmetric. Normally, there is little or no enhancement of gray matter, white matter, cerebrospinal fluid (CSF), muscle, fat, and cortical bone.
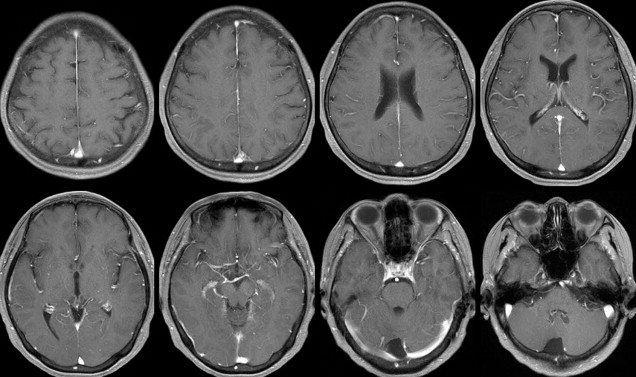
Clinical application
MR imaging has been accepted as the most sensitive method, and diagnosing brain lesions and has been the first step in classifying a lesion according to location, contrast enhancement, perilesional edema, mass effect, necrosis, and hemorrhage. Contrast enhancement in brain lesion could be a sign of BBB breakdown by the abnormal structure of tumor vessels (extravascular) or of increased vascularity (intravascular). Interstitial enhancement is related to alterations in the permeability of the BBB, whereas intravascular enhancement is proportional to increases in blood flow or blood volume. Conventional CE MR imaging improves the capability to identify lesions not visible on unenhanced MR imaging and to provide additional information on lesion morphology, delineation, physiology, and biology. In the imaging of neoplastic brain lesion, however, conventional CE MR imaging has the limitation in the evaluation of early response after treatment and differential diagnosis of recurrent tumor, pseudoprogression, and radiation necrosis. Use of functional MR imaging techniques, such as DWI, MRS, and perfusion MR imaging, can improve the diagnostic accuracy and provide more reliable differentiation of brain tumors. Use of GBCAs in perfusion MR imaging, including dynamic susceptibility contrast and dynamic CE MR imaging, are discussed by Law elsewhere in this issue.
Knowledge of the patterns and mechanisms of contrast enhancement facilitates radiologic differential diagnosis, and understanding the classic patterns of lesion enhancement can improve image assessment and differential diagnosis of brain lesions. Smirniotopoulos and colleagues summarized patterns of contrast enhancement in the brain and meninges ( Table 2 ). Extra-axial enhancing lesions include primary neoplasms (meningioma), granulomatous disease (sarcoid), and metastases. Linear pachymeningeal (dura-arachnoid) enhancement occurs after surgery and with spontaneous intracranial hypotension. Leptomeningeal (pia-arachnoid) enhancement is present in meningitis and meningoencephalitis. Superficial gyral enhancement is seen after reperfusion in cerebral ischemia, during the healing phase of cerebral infarction, and with encephalitis. Nodular subcortical lesions are typical for hematogenous dissemination and may be neoplastic (metastases) or infectious (septic emboli). Deeper lesions may form rings or affect the ventricular margins. Ring enhancement that is smooth and thin is typical of an organizing abscess, whereas thick irregular rings suggest a necrotic neoplasm. Some low-grade neoplasms are fluid secreting, and they may form heterogeneously enhancing lesions with an incomplete ring sign as well as the classic cyst-with-nodule morphology. Demyelinating lesions, including both classic multiple sclerosis and tumefactive demyelination, may also create an open ring or incomplete ring sign. Thick and irregular periventricular enhancement is typical for primary CNS lymphoma. Thin enhancement of the ventricular margin occurs with infectious ependymitis (see Table 2 ).
Location | Enhancement Pattern | Lesions | |
---|---|---|---|
Extra-axial | Pachymeningeal |
|
|
Leptomeningeal |
|
| |
Intra-axial | Superficial gyral |
|
|
Cortical/subcortical |
|
| |
Deep gray/white matter |
|
| |
Periventricular |
|
|
Neoplastic disease
The goals of tumor imaging are multiplex according to the stage of management for initial diagnosis, during the treatment, and on follow-up immediately and longer after therapy. During the initial diagnosis of brain tumor, neuroimaging has essential roles: (1) to differentiate it from nontumorous condition, (2) to precisely define the location and extent of the lesion, (3) to make a diagnosis and a differential diagnosis, (4) to estimate the grade of tumor, (5) to evaluate possible complication made by the tumor (hemorrhage, herniation, hydrocephalus, and so forth.). During the therapeutic planning and surgical/radiosurgical treatment, neuroimaging is an essential part of the decision-making process for therapy and provides valuable anatomic information to guide biopsy, resection, and radiosurgical therapy. For therapeutic follow-up, neuroimaging is also mandatory to monitor disease progression and therapeutic response, including the differentiation of recurrent tumor from radiation necrosis and possible side effects.
Evolution of applications and protocols for MR imaging and GBCA prompts a continued reassessment of the optimal use of these technique and agents. From the consensus discussion of small group of experts in neuroradiology, neuro-oncology, neurosurgery and radio-oncology, Essig and colleagues have recently proposed recommendations on practical application of MR imaging of neoplastic CNS lesions as follows. (1) MR imaging is the technique of choice for the differential diagnosis, tumor grading, and treatment planning of neoplastic CNS lesions, and advanced MR imaging techniques provide physiologic data relevant to diagnosis and grading that may assist conventional MR imaging. (2) MR imaging is an important reference point for monitoring treatment response and recurrence, but the McDonald criteria have limitations. New criteria for assessing enhancing/nonenhancing lesions offer amended guidance for response assessment. Advanced MR imaging techniques may help assess the post-therapeutic brain when contrast enhancement is nonspecific. (3) As optimization of the protocol sequence enhances CNS lesion characterization, a standardized protocol sequence for conventional MR imaging includes T1W precontrast, T2-weighted (T2W) DWI, and T1W contrast imaging. Additional advanced MR imaging techniques can be selected according to the clinical scenario. Higher field strengths (eg, 3 T vs 1.5 T) provide superior image quality, if available. Image acquisition at up to 20 minutes post–contrast injection offers improved lesion detection; (4) a single dose (0.1 mmol/kg of body weight) of GBCA is recommended for suspected primary lesions, with a second administration in cases of diagnostic doubt. In patients with a glomerular filtration rate below 30 mL/min/1.73 m 2 , a single dose only is recommended. With a glomerular filtration rate of 30 mL/min/1.73 m 2 to 60 mL/min/1.73 m 2 , a single dose or double dose may be used; and (5) contrast media with a macrocyclic structure (eg, gadobutrol, gadoterate dimeglumine, and gadoteridol) have a higher chelate stability than linear agents. Contrast agents with high gadolinium concentration and higher relaxivity are preferred for superior enhancement. Gadobutrol offers the highest gadolinium concentration (1 mol/L) and high relaxivity to provide the highest T1 shortening effect among currently available agents.
Tumors of Neuroepithelial Tissue
Astrocytic tumors
Glioma is a tumor arising in the supportive parenchymal cells or glial cells of the brain, which are made up of astrocytes, oligodendrocytes, ependymal cells, and microglial cells. Primary brain tumors account for two-thirds of all brain tumors, and gliomas are approximately 45% to 50% of primary brain tumors. They occur most commonly in the cerebrum and occur most frequently between the ages of 40 and 70 years. Grading of tumors can be difficult both by diagnostic imaging and from surgical biopsy, because gliomas may be heterogeneous in terms of tumor grade.
In glioma, contrast enhancement on CE MR imaging is determined by the degree of intratumoral angiogenesis and BBB breakdown (see Fig. 2 ). Contrast enhancement is generally correlated with grade of malignancy for diffuse astrocytomas (low-grade astrocytoma, anaplastic astrocytoma, and gliblastoma multiforme) ; however, it is not usually for circumscribed astrocytomas (pilocytic astrocytoma, subependymal giant cell astrocytoma, and pleomorphic xanthoastrocytoma). It is frequent that a single astrocytoma has both low-grade and high-grade histologic components. If there is focal area of contrast enhancement within the low-grade astrocytoma, it may have high chance of localized malignant transformation ( Fig. 4 ).
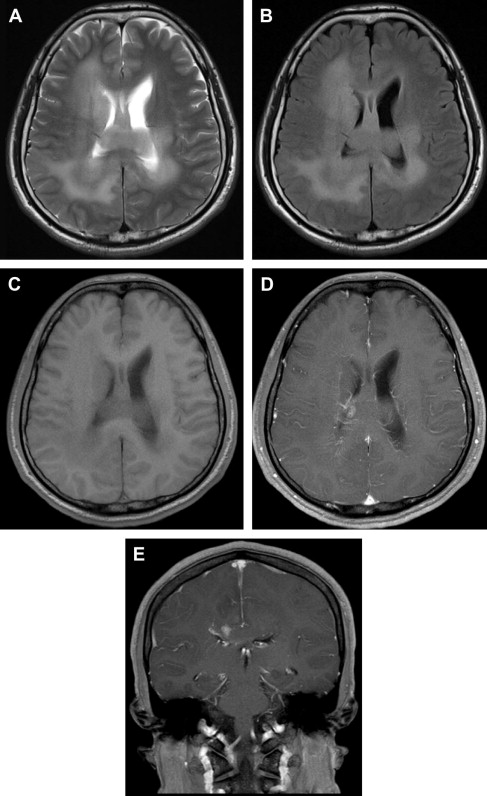
Low-grade astrocytoma consists of well-differentiated fibrillary astrocyte and subtle increase in cell density and cellular atypia but has no other finding of anaplasia. Low-grade glioma may eventually progress to high-grade glioma. Because low-grade astrocytoma has low cellular density, it shows T1 low, T2 bright signal intensity, and, rarely, contrast enhancement because BBB is generally intact. The absence of contrast enhancement is also consistent with the absence of any histologic vascular changes.
Anaplastic astrocytoma is a lesion of intermediate aggression, between simple astrocytoma (World Health Organization grade 2) and glioblastoma multiforme (World Health Organization grade 4). Anaplastic astrocytomas show variable enhancement characteristics. They may present as a nonenhancing, homogeneous region of abnormal signal intensity and/or expanded brain. In some cases, there may be patchy contrast enhancement ( Fig. 5 ). Because there is no necrosis by pathologic definition, heterogeneous ring-like enhancement does not occur.
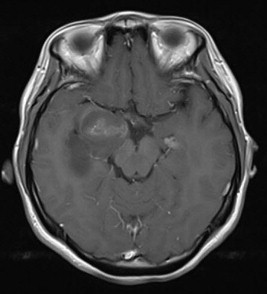
Glioblastoma multiforme is the most malignant of the glial neoplasm and comprises more than half of all adult glial tumors. The magnetic resonance appearance of glioblastoma multiforme includes a mass lesion with irregular ring-like enhancement, prominent surrounding vasogenic edema, and heterogeneity of signal. These tumors may exhibit cystic and solid components and hemorrhage is common. The central necrosis does not enhance and is surrounded by living tumor, which shows prominent ring-like, irregular enhancement of variable thickness ( Fig. 6 ). Because the tumor vessels sprout from pre-existing normal vasculature, the enhancing rim may be thicker on the cortical or outer surface compared with the thinner, deep or white matter margin. Contrast enhancement is important in patient management. CE MR imaging can be particularly helpful in planning of surgical biopsy, because enhancement correlates with tumor viability.
Pilocystic astrocytoma is one of the most benign of all primary CNS tumors and is the most common posterior fossa tumor in childhood. It is often cystic or partially cystic, usually encapsulated, and grows slowly. It can be totally cured by surgical removal with the best prognosis of all astrocytomas. On MR imaging, pilocytic astrocytomas may appear as a well-circumscribed cystic mass with prominent contrast-enhancing nodule in posterior fossa (cyst with nodule). This nodular portion usually demonstrates contrast enhancement, and variable degree of wall enhancement may also occur ( Fig. 7 ). In addition to pilocytic astrocytoma, supratentorial brain tumor having cyst with contrast-enhancing nodule may also include pleomorphic xanthoastrocytoma, gangliogioma, and hemangioblastoma.
Pleomorphic xanthoastrocytoma originates from subpial astrocytes and occurs superficially in the brain. Although the histology may appear malignant, most pleomorphic xanthoastrocytoma have a benign clinical course. It occurs mainly in childhood and adolescence. The most common tumor location is the temporal lobe followed by parietal frontal and occipital lobes. The tumors are mostly superficially located with common involvement of the cortex. They appear as cystic, mixed cystic-solid, or solid lesions. The mural nodules of the mixed cystic-solid tumors are closed to the leptomeningeal surface ( Fig. 8 ). Multiple minute cysts are also noted in the solid tumors. The solid components of tumors, including the mural nodules or thick cystic walls, show marked enhancement with contrast media.
Subependymal giant cell astrocytoma is a clinically benign tumor arising from the wall of the lateral ventricle near the foramen of Monro. It is associated with tuberous sclerosis and is believed to originate from subependymal tuberous sclerosis nodules. On MR imaging, subependymal giant cell astrocytoma typically shows a well-circumscribed mass at the foramen of Monro, which frequently exhibits partial calcification or cyst formation. Enhancement after contrast administration is strong but inhomogeneous.
Oligodendroglial tumors
Oligodendroglioma is a well-differentiated, diffusely infiltrating tumor of adults that is typically located in the cerebral hemispheres. The tumors are derived from supportive cells and may also present as oligoastrocytoma with mixed histology. They are most commonly located in the frontal lobes. They tend to grow toward the cortex rather than infiltrating into the centrally located corpus callosum. They are slowly growing and often heterogeneous. Because of the slow-growing manner, the lesions may be large at the time of presentation; 90% of oligodendrogliomas show histologic calcification ( Fig. 9 ). Oligodendrogliomas have been reported to show frequent postcontrast enhancement on MR imaging. Contrast enhancement is suggested as proportional to tumor vascularity. Contrast enhancement as well as perilesional edema is associated with high-grade oligodendroglioma.
Ependymal tumors
Ependymomas are rare tumors of neuroectodermal origin, arising from the epithelium lining the ventricles. They are classified as myxopapillary ependymoma and subependymoma (grade I), ependymoma (grade II), and anaplastic ependymoma (grade III). Ependymoma is generally a tumor of childhood and located in the fourth ventricle region, although in adults they can be seen in the lateral and third ventricles. Lesions appear as soft plastic masses that squeeze out fourth ventricle foramina into cerebellopontine angle or cisterna magna, pushing against the walls of the ventricle without invasion. Myxopapillary ependymomas are typically and almost exclusively located in the conus–cauda equina–filum terminale region. Subependymomas are typically located in the fourth and lateral ventricles. On CE MR imaging, ependymoma is well vascularized and enhances intensely with gadolinium. On the contrary, subependymoma shows different MR imaging features depending on anatomic location; calcification and heterogeneous contrast enhancement are common features of fourth ventricular subependymomas, whereas lack of calcification and minimal or no contrast enhancement in the lateral ventricular lesions ( Fig. 10 ).
Choroid plexus tumors
Choroid plexus tumors arise from choroid plexus, and there are 2 primary types: the well-differentiated and benign choroid plexus papilloma and the malignant less-differentiated choroid plexus carcinoma. They are most common in the first decade of life. Choroid plexus tumors are almost invariably intraventricular masses, usually seen in lateral ventricle in children, not infrequently in fourth ventricle in adults. On CE MR imaging, choroid plexus papilloma typically shows densely contrast-enhancing intraventricular mass ( Fig. 11 ). Choroid plexus papilloma usually has limited focal parenchymal invasion; thus, presence of extensive subependymal invasion should raise the suspicion of choroid plexus carcinoma.
Neuronal and mixed neuroglial tumors
Dysembrioplastic neuroepithelial tumor (DNT) is mixed neuroglial tumor, usually associated with chronic epilepsy in adolescents and young adults. It is most commonly located in the temporal lobe as intracortical lesion and causes no neurologic deficit. Multicystic appearance on MR images is a characteristic feature of DNT and corresponds to its myxoid matrix and multinodular architecture. The cystic, in most cases even multicystic, appearance on MR images is a typical feature of DNT despite the absence of true cysts intraoperatively or on microscopic examination. On CE MR imaging, multifocal faintly nodular or ring enhancement is seen in one-third of cases ( Fig. 12 ).
Gangliogliomas are slow-growing neoplasms composed of 2 types of cells: neoplastic glial cells and neoplastic ganglion cells. They most commonly occur in children and young adults. The most common location in the brain is the temporal lobe, followed by frontal lobe. On MR imaging, they are typically well-circumscribed masses, often with partially cystic change and with no or little edema. Contrast enhancement is reported in approximately one-half of tumors ( Fig. 13 ).
Central neurocytoma occurs in adults and typically in the lateral ventricle or in the subependymal region. The imaging appearance of central neurocytoma is characteristic and consists of an entirely intraventricular mass near the foramen of Monro, showing well-circumscribed tumor lesion with isointense to hyperintense signal on most MR sequences. Contrast enhancement is variable in degree and pattern, from slight to heterogeneously intense contrast enhancement ( Fig. 14 ).
Tumors of the pineal region
Pineocytoma and more malignant pineoblastoma are rare and have been estimated as less than 15% of all pineal region tumors. On MR imaging, pineocytomas on T1W images show rounded, sometimes or slightly lobulated low signal intensity masses with strong and homogeneous contrast enhancement. Their margin is clear, without invasion of adjacent structures. The pineoblastomas, however, show multilobulated tumor with heterogeneous contrast enhancement and poorly defined margin with adjacent structures, including posterior thalamus or corpus callosum by more invasive nature.
Embryonal tumors
Medulloblastomas (most common type of Primitive neuroectodermal tumors [PNETs]) comprise a heterogeneous group of undifferentiated malignant tumors of brain. PNETs of the cerebellum are historically referred to as medulloblastomas, whereas supratentorial PNETs have been called cerebral medulloblastoma, cerebral neuroblastomas or ganglioneuroblastoma, ependymoblastoma, and undifferentiated small cell neoplasms of the brain. They are highly malignant due to locally aggressive behavior and subarachnoid dissemination, for which contrast enhancement is helpful to delineate extent. Most of the lesions show variable contrast enhancement from mild to intense degree.
Tumors of Cranial and Paraspinal Nerves
Schwannoma is the second most common intracranial extra-axial neoplasm in adults, and 90% of them arise from the acoustic nerve, followed by trigeminal and facial nerves. Vestibular schwannoma is the most common solid mass involving the cerebellopontine angle and internal auditory canal. It is mostly a solitary lesion but may be multiple in patients with neurofibromatosis type 2. On CE MR imaging, it generally shows strong contrast enhancement, which is sometimes heterogeneous with cystic changes ( Fig. 15 ). Contrast enhancement pattern may be similar to cerebellopontine angle meningioma, but menigioma usually has broad base to the adjacent dura and dural tail, and schwannomas often cause flaring of the porus acousticus with extension into the internal auditory canal.
